CHAPTER 9 The knee
Biomechanics of the extensor mechanism
The patella is the largest sesamoid bone in the body. It is attached above to the quadriceps tendon, below to the patellar tendon, and medially and laterally to the patellar retinacula. The breadth of the pelvis and close proximity of the knee creates a valgus angulation to the femur. Coupled with this, the direction of pull of the quadriceps is along the shaft of the femur and that of the patellar tendon is almost vertical (Fig. 9.1). The difference between the two lines of pull is known as the Q angle and is an important determinant of knee health. Normal values for the Q angle are in the region of 15–20°, and knees with an angle greater or less than this can be considered malaligned.
The lower fibres of the vastus medialis can be considered as a functionally separate muscle, the vastus medialis oblique (VMO) (Speakman and Weisberg, 1977). The quadriceps as a whole have been shown to undergo reflex inhibition as the knee swells (de Andrade, Grant and Dixon, 1965; Stokes and Young, 1984). However, the VMO can be inhibited by as little as 10 ml effusion while the vastus lateralis and rectus femoris require as much as 60 ml (Arno, 1990). Minimal effusion occurs frequently with minor trauma and may go unnoticed by the athlete. However, this will be enough to weaken the VMO and alter the biomechanics of the patella.
Patellar contact area
In full extension the patella does not contact the femur, but lies in a lateral position. As flexion progresses, the patella should move medially. If it moves laterally it will butt against the prominent lateral femoral condyle and the lateral edge of the patellar groove of the femur. As flexion progresses, different areas of the patella’s undersurface are compressed onto the femur. At 20° flexion the inferior pole of the patella is compressed, and by 45° the middle section is affected. At 90° flexion, compression has moved to the superior aspect of the knee. In a full squatting position, with the knee reaching 135° flexion, only the medial and lateral areas of the patella are compressed (Fig. 9.2). Compression tests of the patella to examine its posterior surface must therefore be performed with the knee flexed to different angles.
Patellofemoral loads may be as high as three or four times body weight as the knee flexes in walking, and nine times body weight when descending stairs (Cox, 1990). While the posterior surface of the patella is compressed, the anterior aspect receives a tensile force when seen in the sagittal plane (Fig. 9.3B). The effect of the Q angle is to create both horizontal and vertical force vectors which tend to compress the lateral aspect of the patella but submit the medial aspect to tensile stress (Fig. 9.3A). Clearly, alterations in Q angle will change the pattern of stress experienced by the patellar cartilage.
Patellofemoral pain syndrome
Pathology
Pain to the undersurface of the patella is variously called anterior knee pain, chondromalacia patellae, patella malalignment syndrome and patellofemoral pain syndrome (PFPS). The last term is used in this text. It is a condition affecting the posterior surface of the patella, and is sometimes attributed to cartilage damage and, on occasion, incorrectly seen as a direct precursor to osteoarthritis. Since hyaline cartilage is aneural, changes in the patellar cartilage surface itself would not result in PFPS. Furthermore, at arthroscopy cartilage changes are often seen in patients who have no PFPS. If cartilage degeneration does occur with this condition, it is to the ground substance and collagen at deep levels on the lateral edge of the patella. This results in a blistering of the cartilage as it separates from the underlying bone, but the cartilage surface itself is still smooth (Gruber, 1979). In osteoarthritis (OA) the initial changes occur to the cartilage surface of the odd facet (medial) and are followed by fibrillation.
The retinacula supporting the patella may be a major source of pain (Fulkerson, 1982), or the subchondral bone of the odd facet (Hertling and Kessler, 1990). As we have seen, the odd facet is only occasionally compressed in a full squatting position, and so its subchondral bone is less dense and weaker. Lateral movement of the loaded patella could pull the odd facet into rapid contact with the patellar surface of the femur, causing pain. Sources of pain are summarized in Table 9.1.
Table 9.1 Source of pain in PFPS
PFPS has a multifactorial etiology. Associated factors may be categorized as local and remote (Crossley et al., 2007). Local factors are those directly associated with the patella structure; remote factors have an effect on the patella through other structures. Table 9.2 shows some of the most common factors associated with PFPS.
Table 9.2 Factors associated with patella femoral pain syndrome (PFPS)
Factor | Clinical sign |
---|---|
Remote | |
Internal rotation of femur | Squinting patella due to femoral internal rotation |
Knee valgus increased | Knock knee position, more noticeable during squatting. Often associated with poor gluteus medius tone |
Tibial rotation | Often associated with foot biomechanics |
Foot (subtalar) pronation | Drop foot or high arch position linked to tibial rotation |
Muscle flexibility | Hamstrings, rectus femoris, ITB/TFL, gastrocnemius |
Local | |
Patella position | Patellar resting position and passive motion |
Soft tissue characteristics | Compliance of medial and laterally placed tissues |
Muscular control of quadriceps | Muscle wasting/weakness. Timing of VMO contraction. Tracking of patella |
ITB/TFL—iliotibial band/tensor fascia lata; VMO—vastus medialis obliqus.
From Crossley et al. (2007).
Muscular factors
Flexibility and strength of the knee tissues and muscles will often reveal asymmetry. The relationship between the hamstrings and quadriceps (HQ ratio) is particularly important and may require isokinetic assessment of peak torque values. Isokinetic testing also demonstrates characteristic changes in the PFPS patient (Fig. 9.4). Eccentric torque production during knee extension is often poor (Bennett and Stauber, 1986) and the torque curve may be irregular (Hoke, Howell and Stack, 1983). Both changes have been suggested to represent a deficiency in motor control, which would explain the often rapid response to quadriceps training that is achieved in these patients. One possibility is that malalignment and patellofemoral (PF) pressure alterations may result partly from subtle shifts in the timing or amount of VMO activity, in particular parts of the movement range (Reid, 1992). The aim of rehabilitation is therefore more a case of motor skill acquisition than pure strength training.
Weakness or malfunction in the VMO will allow the patella to drift laterally as the quadriceps contract. Using ultrasound imaging Herrington and Pearson (2008) were able to show medial displacement of the patella (6.8 mm) with VMO contraction and lateral displacement (5.6 mm) with vastus lateralis (VL) contraction in vivo. Normally the ratio of VMO to VL is approximately 1 : 1, and VMO activity is that of a stabilizing muscle in that it is tonic (Reynolds et al., 1983). In the PFPS patient the VMO to VL ratio is less than 1 as the VMO weakens. In addition, its contractile nature becomes phasic, as its endurance capacity is reduced.
Closed chain VMO re-education may be carried out by performing limited range squats (1/4 squat exercise) or lunges moving the knee from 20–30° flexion to full extension. Step downs from a single stair are useful as they can retrain correct knee motion. The patient should be instructed to keep the knee over the centre of the foot (avoiding adduction and medial rotation) throughout the movement. The use of surface electromyography (sEMG) can help with re-education. The sEMG electrode is placed over the VMO and the patient is taught to activate the muscle in standing and then to maintain this activation throughout the 1/4 squat exercise. The full motor pattern is of foot supination, slight hip abduction and external rotation while maintaining VMO contraction. This may be achieved by standing side on to a wall with the injured leg on the outside (Fig. 9.5). The inner knee and hip are flexed to 45° and this knee presses against the wall, enabling the athlete to hold the trunk vertical while standing on one leg. This body position places significant loading on the gluteus medius of the outer leg to maintain the horizontal pelvic alignment. The foot is supinated, leg turned out and knee slightly flexed to 20°. EMG biofeedback is used over the VMO, and palpation is used to facilitate gluteus medius activity (McConnell, 1994).
In cases where genu recurvatum is present, strengthening of the hamstrings may be required in an attempt to correct the knee hyperextension. In addition to knee musculature, hip strength is particularly important. The hip abductors and lateral rotators warrant special attention as weakness here has been associated with this condition (Beckman, Craig and Lehman, 1989). It is common for young athletes to allow the knee to adduct and medially rotate when descending stairs. This may be due to weakness in the hip abductors, particularly gluteus medius, causing the iliotibial band (ITB) to overwork and tighten. This structure in turn pulls on the patella laterally, displacing or tilting it. Manual muscle testing of the gluteus medius in a side-lying position will often reveal weakness in the affected leg, and tightness in the ITB should be evaluated.
Muscle tightness must be evaluated. The hamstrings, ITB, quadriceps, hip flexors (iliopsoas and rectus femoris), hip rotators and gastrocnemius should all be addressed, as tightness in these structures can alter both knee alignment and gait. Tests, which may also be used as stretching exercises, are shown with average values in Table 9.3. ITB tightness may pull the patella laterally during flexion, while tight hamstrings could result in increased knee flexion and a resultant increase in patellofemoral compression forces. A tight gastrocnemius, in addition to increasing or prolonging knee flexion during gait, will also cause compensatory subtalar pronation.
Table 9.3 Flexibility tests/exercises used in the management of anterior knee pain
Foot biomechanics
During normal running gait (see Chapter 7), the subtaloid joint (STJ) is slightly supinated at heel strike. As the foot moves into ground contact, the joint pronates, pulling the lower limb into internal rotation and unlocking the knee. As the gait cycle progresses, the STJ moves into supination, externally rotating the leg as the knee extends (locks) to push the body forward. This biomechanical action is combining mobility and shock absorption (STJ pronation and knee flexion) with rigidity and power transmission (STJ supination and knee extension), and shows the intricate link between foot and knee function.
In certain circumstances the patella can cope with this extra stress, but if additional malalignment factors exist, they are compounded (Fig. 9.6). Anteversion of the femur (internal rotation), VMO weakness and tightness of the lateral retinaculum may all increase the lateral patellar tracking causing symptoms (Tiberio, 1987). For PFPS to be treated effectively therefore, a biomechanical assessment of the lower limb is mandatory. If hyperpronation is present, it must be corrected. This will involve assessment of sports footwear, patient education and orthotic prescription.
Although clinically patients with PFPS often improve with the prescription of orthoses, the evidence for their use is poor. In a study comparing physiotherapy management (PF mobilisation, taping, quadriceps muscle re-education) with physiotherapy and orthoses, Collins et al. (2009) studied 179 participants and found contoured foot orthosis to be superior to flat shoe inserts in the short term, but to be no better than physiotherapy with a follow-up of 52 weeks.
Patella position
A number of forces are imposed on the patella as a result of active and passive structures (Fig. 9.7). The vastus lateralis pulls at 12–15° to the long axis of the femur, while the vastus medialis longus pulls at 15–18° and the VMO at 50–55° (Lieb and Perry, 1968). The medial and lateral retinacula, if tight, may tilt the patella (Norkin and Levangie, 1992). The ITB attaches to the patella via a small slip from its lower end called the iliopatellar band (Terry, Hughston and Norwook, 1986). The ITB has a connection to the biceps femoris through the lateral intermuscular septum. Loading the ITB has been shown to both displace the patella laterally and move the contact area of the patellofemoral joint laterally. In addition the pull of the ITB imparts a lateral rotation stress onto the tibia (Kwak et al., 2000). Subjects with PFPS have been shown to have a significantly tighter ITB on their symptomatic side (Hudson and Darthuy, 2009). Lateral patella displacement has been shown to correlate with ITB length when measured using a modified Ober test where the upper leg is straight and pelvic position is monitored using pressure biofeedback (Herrington, Rivett and Munro, 2006).
Quantifying the position of the patella is important because, as we have seen above, excessive pressure on the odd facet may result if the patella position is at fault. McConnell (1986) described four different patellar position faults which could be assessed with the patient in the supine position with the quadriceps relaxed. By using the patellar poles as landmarks and comparing their position to the planes of the femur, any malalignment becomes evident. In addition, accessory patellar movements can be assessed with particular emphasis on medial and lateral gliding.
Patellar glide occurs when the patella moves from a neutral position. The distance from the centre of the patella to the medial and lateral femoral condyles is assessed. A difference in the medial distance compared to the lateral of greater than 0.5 cm is significant (Fig. 9.8A). Tightness in the lateral retinaculum, a frequent occurrence in PFPS sufferers, will cause lateralization of the patella. Patellar tilt evaluates the position of the medial and lateral facets of the patella, with PF pain patients frequently showing a more prominent medial facet with difficulty actually palpating the lateral and posterior edge of the patella (Fig. 9.8B). Patellar rotation occurs when the inferior pole of the patella deviates from a neutral position. Medial (internal) rotation occurs when the inferior pole of the patella lies medial to the long axis of the femur. Lateral (external) rotation is present when the inferior pole of the patella lies lateral to the long axis of the femur (Fig. 9.8C). Anteroposterior (AP) tilt exists when both the superior and inferior poles are not clear to palpate, indicating that one is lower in the surrounding soft tissue (Fig. 9.8D).
Clinical measurement of patella position has been shown to be reliable and valid. Using 20 experienced manual therapists Herrington (2000) was able to show good agreement between testers when assessing medial and lateral orientation of the patella (r = 0.91 medial measurement, r = 0.94 lateral measurement). In addition validity has been assessed using MRI as the criterion measure, and a good correlation found between clinical examination and MRI measurement (McEwan, Herrington and Thom, 2007).
Measurement of patellar glide is made easier and more accurate by placing a piece of zinc oxide tape over the patella (Fig. 9.9A&B). The knee is flexed to 20° to fix the patella in the trochlea groove of the femur. The medial and lateral epicondyes are marked on the tape together with the mid position of the patella. The tape is removed and the distance between the patella central position and the epicondyles measured.
Alternative measurements of patellar position
Arno (1990) attempted to quantify the patellar position clinically with a description of the A angle. This relates patellar orientation to that of the tibial tubercle. The poles of the patella are palpated and a line is drawn bisecting the patella. Another line is drawn from the tibial tubercle to the apex of the inferior pole of the patella and the angle of intersection forms the A angle (Fig. 9.10). The same author argued that an A angle greater than 35° constituted malalignment when the Q angle remained constant.
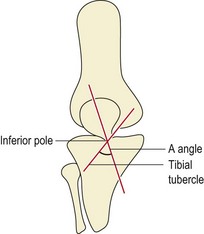
Figure 9.10 The A angle.
From Arno, S. (1990) The A angle: a quantitative measurement of patella alignment and realignment. Journal of Orthopaedic and Sports Physical Therapy, 12(6), 237–242. With permission.
Radiographic assessment of patellar position is more reliable than clinical measurements (Larsen et al., 1995). Three common measurements are used (Fig. 9.11). Patellofemoral congruence angle (PFCA) is the angle formed between a line bisecting the sulcus angle and a line connecting the apex of the sulcus to the lowest aspect of the patellar ridge. Lateral patellofemoral angle (LPFA) is the angle between lines drawn joining the summits of the femoral condyles and the patellar poles. Lateral patellar displacement (LPD) is the distance between the highest point of the medial femoral condyle and the most medial border of the patella.
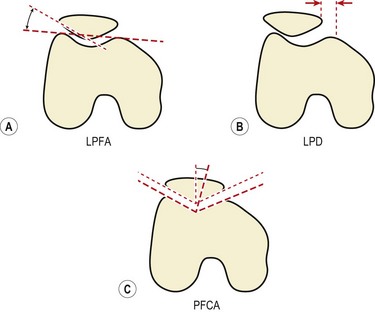
Figure 9.11 Radiographic measurements of patellar position.
Modified from Crossley et al. (2000) with permission.
Using these measurements, patellar malalignment is considered to exist when the LPD is greater than 1 mm, the PFCA is > +5° or the LPFA equals 1° (Crossley et al., 2000).
Patellar taping
Pain relief may often be provided by temporarily correcting any underlying fault in patella position through taping. Exercising with this taping in place may re-educate correct muscle sequencing to improve patellar alignment (McConnell, 1994). Initially, open web adhesive taping is applied to protect the skin against excessive tape drag. The pull of the final taping is applied using 5 cm zinc oxide tape. Decreased medial glide is corrected by pulling a piece of tape from the lateral border of the patella (Fig. 9.12A). The soft tissue over the medial femoral condyle is lifted towards the patella to give a skin bunching appearance. Lateral tilt is corrected again by a medially orientated tape. This time, however, the tape covers only the medial half of the patellar face, and again the medial soft tissue is lifted towards the patella (Fig. 9.12B). Rotation is corrected by pulling the patella around its central axis. Internal rotation is corrected by attaching the tape to the upper inner quadrant of the patella. The tape is pulled down medially to rotate the patella clockwise (Fig. 9.12C). External rotation is corrected by placing the tape over the lower inner quadrant of the patella and pulling anti-clockwise. A posterior tilt of the inferior pole should be corrected first to elevate the pole away from the fat pad. The tape is placed over the upper pole of the patella and the patella is taped medially (Fig. 9.12D).
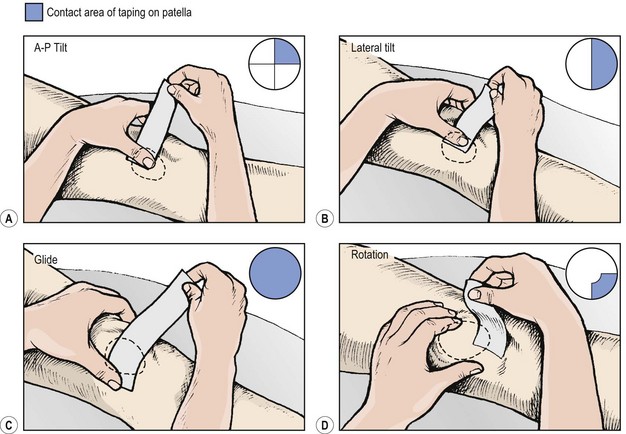
Figure 9.12 Correction of patellar position using tape.
After McConnell, J. (1992) McConnell Patellofemoral Course, London. With permission.
Evidence exists to support the clinical use of patellar taping. Roberts (1989) found a change in LPFA (1.2°) and a reduction in LPD of 1.1 mm in taped knees. Somes et al. (1997) showed a significant improvement in LPFA in weight bearing but none in non-weight bearing with taped knees. Larsen et al. (1995) showed improved PFCA in healthy subjects with taped knees, but this change lessened after 15 minutes of vigorous exercise.
One of the functions of patellar taping is to facilitate selective recruitment of the VMO in the belief that patellar pain patients contract their VMO after the VL (McConnell, 1986). Some studies have supported this hypothesis (Christou and Carlton, 1997; Millar et al., 1999), but others have not (Herrington and Payton, 1997). Interestingly, patellar taping seems to enhance proprioception, but only in those subjects where proprioception is poor to begin with (Callaghan et al., 2000).
Surgery
Before surgery is considered, conservative management must be attempted. Indeed, Insall (1979) stated that surgery was only indicated when continuous pain limited normal activities for at least 6 months and the condition had not responded to conservative management.
The complex aetiology of the condition has led to a number of different surgical procedures (Fig. 9.13).
Release of tight lateral retinaculum is performed through a small incision or arthroscopy to divide the retinaculum from the lower fibres of the vastus lateralis. Although this technique may be used to decrease a patellar tilt greater than 12° (Zachazewski, Magee and Quillen, 1996), the procedure has been shown to be ineffective at treating subluxation (Post and Fulkerson, 1992) or articular degeneration (Shea and Fulkerson, 1992).
Realignment procedures involve structural transfer to reduce or alter compression forces on the patella. The Maquet operation elevates the tibial tubercle to reduce patella reaction forces and the Hauser manoeuvre uses distal and medial transfer to reduce the valgus vector acting on the patellofemoral joint. The Goldthwait procedure involves release and transfer of part of the patellar tendon. Proximal realignment, by moving the attachment of the vastus medialis, aims at increasing the mechanical advantage of the VMO. This technique is used in the young where alteration of the tibial tuberosity will detrimentally affect the apophysis. Facetectomy involves excision of all or part of a single patellar facet, and patellectomy entails excision of the whole patella. It should be noted that the results for surgical treatment of PFPS are generally poor (Crossley et al., 2007).
Patellar fracture
Patellar fractures in sport occur most frequently in adolescent athletes, usually as a result of jumping. Fracture may occur at the pole of the patella, or as transverse, vertical or comminuted injuries. In the young, the bony fragment may pull off a substantial amount of articular cartilage from the patella undersurface, giving a ‘sleeve’ fracture. Stress fracture at the distal third of the patella has been reported after sprinting (Jerosch, Castro and Jantea, 1989). Conservative treatment, consisting of immobilizing the limb in a cast for 2–3 weeks, is sufficient in 50–60% of cases (Exler, 1991). Surgical treatment involves internal fixation of the patellar fragments, and hemipatellectomy or total patellectomy in the case of comminuted injuries, combined with immobilization in a cast.
Patellar dislocation
Patellar dislocation may occur traumatically with any athlete, but is more frequently seen in children between the ages of 8 and 15 years and in middle-aged women who are overweight and have poor muscular development of the quadriceps. Biomechanically, individuals are more susceptible to this condition if they demonstrate genu valgum, femoral anteversion or external rotation of the tibia, and if the VMO is weak. Patellar mobility may be assessed by lateral gliding. If the patella is divided into quadrants (Fig. 9.14), reduced mobility occurs when the patella can only glide laterally by 1 quadarant. Increased mobility and therefore susceptibility to dislocation is present when the patella glides by 2 quadrants or more. In this case, more than half of the patellar surface moves over the femoral condyle (Magee, 2002).
Early quadriceps exercises
The question of which quadriceps exercise to use at the beginning of rehabilitation is one of considerable debate within physiotherapy. The decision depends on a number of factors including PF reaction forces, the efficiency of an exercise to emphasize the VMO, and the relevance of an exercise movement to functional requirements (see Training specificity, Chapter 4).
The choice is often between open and closed chain movements, and bracing or lifting leg actions. In the gait cycle, the quadriceps are active during leg loading as the opposite leg moves into the swing phase, and to a lesser extent at the beginning of toe-off. In jumping, these muscles create very large concentric and eccentric forces in closed chain format. In a fast kicking action they work in an open chain action, but most of the work is from the two-joint rectus femoris (Richardson and Bullock, 1986). Both open chain and closed chain actions are important, but for early stage rehabilitation closed chain action emphasizing stability is more appropriate.
Comparing the leg extension with the leg press, Steinkamp et al. (1993) found PF joint stress, PF reaction force and quadriceps force to be significantly greater in a leg extension exercise from 0–30°, but significantly greater in a leg press action from 60–90°. These authors concluded that the leg press was more appropriate because it placed minimal stress on the PF joint in the functional range of motion and simulated normal movement patterns.
It is often argued that QS with isometric hip adduction will increase the recruitment of the VMO because some of the VMO fibres originate from adductor magnus (Reid, 1992). However, Karst and Jewett (1993) compared quadriceps setting (QS), straight leg raising (SLR), SLR with the hip laterally rotated, and SLR with isometric hip adduction with resistance equivalent to 5% bodyweight. These authors found that QS elicited a greater degree of activity than SLR. In addition, SLR with either hip adduction or lateral rotation failed to increase emphasis on the VMO over that of the rest of the quadriceps.
ITB friction syndrome
The ITB is a non-elastic collagen cord stretching from the pelvis to below the knee. At the top it is attached to the iliac crest where it blends with the gluteus maximus and tensor fascia lata. As the tract descends down the lateral side of the thigh, its deep fibres attach to the linea aspera of the femur. The superficial fibres continue downwards to attach to the lateral femoral condyle, lateral patellar retinaculum and anterolateral aspect of the tibial condyle (Gerdy’s tubercle). A large amount of the lateral retinaculum actually arises from the ITB to form the iliopatellar band having a direct effect on patellar tracking (Zachazewski, Magee and Quillen, 1996).
Aetiology
Tightness of the ITB can occur in a number of patient groups. The tall, lanky teenager who has recently undergone the adolescent growth spurt may experience pain if soft tissue elongation lags behind long bone development. Tightness in adolescent females is a consistent factor in PFPS, although the relationship between the ITB and the patella has been debated by some authors (Rouse, 1996). The second major group of sufferers are adult athletes, particularly distance runners. A number of factors can contribute to problems within this group. Running on cambered roads and using shoes worn on their lateral edge will increase varus knee angulation and may overstretch a tight ITB. Rapid increases in speed or hill work can place excessive stress on the structure. In addition, imbalances of muscle strength and flexibility around the knee and hip may lead to the gradual onset of symptoms.
Pain normally occurs over either the trochanteric bursa or the lateral femoral condyle (Fig. 9.15). Pain is experienced to palpation, but also to limited range squats or lunges on the affected leg. As the knee flexes and the ITB passes over the lateral femoral condyle, friction may occur, causing pain of increasing intensity. Flexibility tests, particularly the Ober manoeuvre and Thomas test, often reveal pain and a lack of flexibility. In addition, compressing the ITB over the proximal part of the lateral femoral condyle with the knee flexing and extending to 30° may elicit pain (Noble, 1980). Where the ITB is tight and the tensor fascia lata overactive, the gluteus medius muscle is normally lengthened. Both muscles must therefore be addressed in treatment.
Management
Weakness in the hip abductors may allow the pelvis to tilt or ‘dip’ during the stance phase of walking or running. This often gives the impression of a mild Trendelenburg gait, and may be habitual following lower limb injury. Gait re-education and abductor strengthening are called for. The abductors may be strengthened from an open chain or more functional closed chain starting position. Open chain strengthening is performed using a weight bag in a side-lying hip abduction exercise. Closed chain strengthening is carried out with the athlete standing on the affected leg, and keeping it locked. The unaffected leg is flexed at the knee. From this position, the pelvis is allowed to drop towards the unsupported side and pulled back to the horizontal position by hip abductor action (Fig. 9.16).
Collateral ligament injuries
The lateral collateral ligament (LCL) is a round cord about 5 cm long, which stands clear of the joint capsule. It travels from the lateral epicondyle of the femur to the lateral surface of the head of the fibula. In some subjects the ligament is continuous with the peroneus longus muscle. The ligament splits the tendon of biceps femoris, and is separated from the joint capsule by the popliteus muscle, and the lateral genicular vessels and nerve (Palastanga, Field and Soames, 1989). The lower end of the lateral ligament is pulled back in extension, and forwards in flexion of the knee.
Palpating the collateral knee structures
Palpation of the medial aspect of the knee is made easier by dividing the area into thirds (Fig. 9.17). The anterior third comprises the edge of the patellar tendon and extensor retinaculum and the superficial border of the MCL. Inferior and medial to the tibial tubercle are the insertions of semi-tendinosus, sartorius and gracilis (pes anserine structures). The middle third comprises the MCL and the coronary ligaments. The posterior third comprises the deep part of the MCL and the diverse expansion from the semi-membranosus. Palpation of the lateral aspect of the knee may be similarly divided into thirds (Fig. 9.18). The anterior third consists of the lateral edge of the patellar tendon and the lateral retinaculum. The middle third is dominated by the ITB and the posterior third consists of the fibular collateral ligament, the tendon of biceps femoris, the lateral head of gastrocnemius and popliteus.
Ligament tests
The easiest way to perform the varus/valgus test is with the patient’s hip abducted, thigh supported on the couch or a rolled towel and the lower leg over the couch side. Where a lower couch is used the practitioner may need to use his/her own thigh to rest the patient’s leg (Fig. 9.19). The practitioner’s hands are positioned for maximum leverage with pressure coming through the forearms rather than from the hands alone. The limb is tightly controlled by holding close to the joint line and supporting the leg against the practitioner’s body.
First and second degree injuries are generally treated conservatively. Third degree injuries (complete rupture) have been treated surgically, but some authors argue that stability of the knee is not improved to a greater extent than with non-operative intervention (Keene, 1990). First degree injuries are generally treated partial or full weight bearing with the ligament supported by strapping. Second and third degree injuries are managed non-weight bearing.
Initially, the aim is pain relief, swelling reduction and the start of mobile scar formation. Isometric quadriceps drill is begun and modalities used to reduce pain and swelling (Table 9.4). At night a knee brace may be used to protect the ligament. By the third or fourth day after injury (sometimes earlier with a first degree and later with a third degree injury) gentle mobility exercises are begun, either in a side-lying starting position or in the pool. Gentle transverse frictions are used to encourage mobile scar formation. The sweep should be quite broad and a large section of the ligament treated. Free or light resisted exercises are begun to the knee, hip and calf musculature within the pain-free range. Isokinetics may be used with the aim of restoring the HQ ratio to that of the uninjured limb.
Table 9.4 Guidelines for medial collateral ligament rehabilitation
Multi-direction agility skills (sports specific), e.g. zig-zag run, shuttle run, plyometric exercises |
* Criterion for progression to phase three: no joint effusion; minimal pain to direct ligament palpation; full or near full painless range of motion; knee stable to hop/hop and turn tests. After Reid (1992), with permission.
Occasionally, anteroposterior X-ray will show a bony plaque under the femoral attachment of the MCL (Pellegrini-Stieda disease). The attachment of the adductor magnus onto the adductor tubercle may also be partially avulsed. The condition is normally due to ossification of the haematoma formed at the time of injury (Apley and Solomon, 1993), and MCL injuries which do not improve or get worse with treatment should be examined radiographically to check for this condition. Infrequently it may occur in the absence of apparent trauma. The condition will normally resolve with rest, but where pain is continuous, surgical removal is required.
Cruciate ligaments
Structure and function
The ACL provides 86% of the resistance to anterior displacement and 30% to medial displacement, while the PCL provides 94% of the restraint to posterior displacement and 36% to lateral stresses (Palastanga, Field and Soames, 1989).
Injury
Of the two ligaments, the ACL is far more commonly injured in sport, with over 70% of knee injuries with acute haemarthrosis involving ACL damage (Noyes, Bassett and Grood, 1980). The athlete has often participated in either a running/jumping activity or skiing. The history is usually of a non-contact movement such as rapid deceleration, a ‘cutting’ action in football, or a twisting fall. The combination is frequently one of rotation and abduction, a similar action to that which causes MCL or medial meniscus damage, and the three injuries often coalesce to form an ‘unhappy triad’.
Manual testing
The classic anterior draw test (Fig. 9.20) involves flexing the patient’s knee to 90° and stabilizing the foot with the examiner’s bodyweight. The proximal tibia is pulled anteriorly and the amount of movement compared to the ‘normal’ value of the uninjured leg. Various grades of movement may be assessed, grade 1 being up to 5 mm of anterior glide, grade 2, 5–10 mm and grade 3 over 30 mm. The test can, however, give false negatives if haemarthrosis prevents the knee being flexed to 90°. Movement can also be limited by protective hamstring spasm or if the posterior horn of the medial meniscus wedges against the medial femoral condyle.
Lachman test
The Lachman test, a modification of the anterior draw, has been shown to be highly reliable (Donaldson, Warren and Wickiewicz, 1985). The test is performed with the patient lying supine. The examiner holds the patient’s knee in 20° flexion, minimizing the effect of hamstring spasm and reducing the likelihood of meniscal wedging. The reduced angle of flexion compared with the anterior draw test is less painful for the patient, and comfort can be further enhanced by placing the knee over a pillow. One hand stabilizes the femur and the other applies an anterior shearing force to the proximal tibia, avoiding medial rotation (Fig. 9.21A).
Clinically, the test may be modified in a number of ways to avoid holding the weight of the whole leg. The therapist may place his or her flexed knee on the couch and rest the patient’s leg over it (Fig. 9.21B). Alternatively, the patient’s femur may be supported on the couch with the tibia over the couch end. The femur is stabilized with a strap, leaving both of the therapist’s hands free to shift the tibia (Fig. 9.21C). If anterior translation of the tibia is felt, the test is positive. The movement is compared to the uninjured knee, both for range and end-feel, an ACL tear giving a characteristically soft end-feel. The same grading system is used as with the anterior draw test.
With the anxious patient who is unable to relax, the reverse Lachman test may be used (Rebman, 1988). Here, the patient is in prone lying with the knee flexed to 20°. The examiner grasps the patient’s tibia with the forefingers over the tibial tubercle and the thumbs over the politeal fossa (Fig. 9.21D). Anterior displacement, rather than being felt (as in the classic Lachman test) is actually seen with this modified test.
Pivot shift tests
Another frequently used test is the pivot shift, and its adaptations (Galway, Beaupre and MacIntosh, 1973). These work on the basis that the ACL-deficient knee will allow the lateral tibial plateau to sublux anteriorly (Fig. 9.22). By applying forces to enforce this and then moving the knee, the tibia can be made to reduce rapidly, causing a ‘thud’. The pivot shift test starts with the affected leg in full extension. The examiner grasps the ankle of this leg with his or her distal hand and the outside of the ipsilateral knee with his or her proximal hand. The ankle and tibia are forced into maximum internal rotation, subluxing the lateral tibial plateau anteriorly. The knee is slowly flexed as the proximal hand applies a valgus stress. If the test is positive, tension in the ITB will reduce the tibia at 30–40° causing a sudden backward ‘shift’. The major disadvantage with this test is that the patient must be relaxed throughout the manoeuvre, a situation often not possible because of pain. Donaldson, Warren and Wickiewicz (1985) tested over 100 ACL-deficient knees preoperatively and found the pivot shift test to be positive in only 35% of cases. The same examination carried out under anaesthesia (muscles completely relaxed) gave 98% positive results.
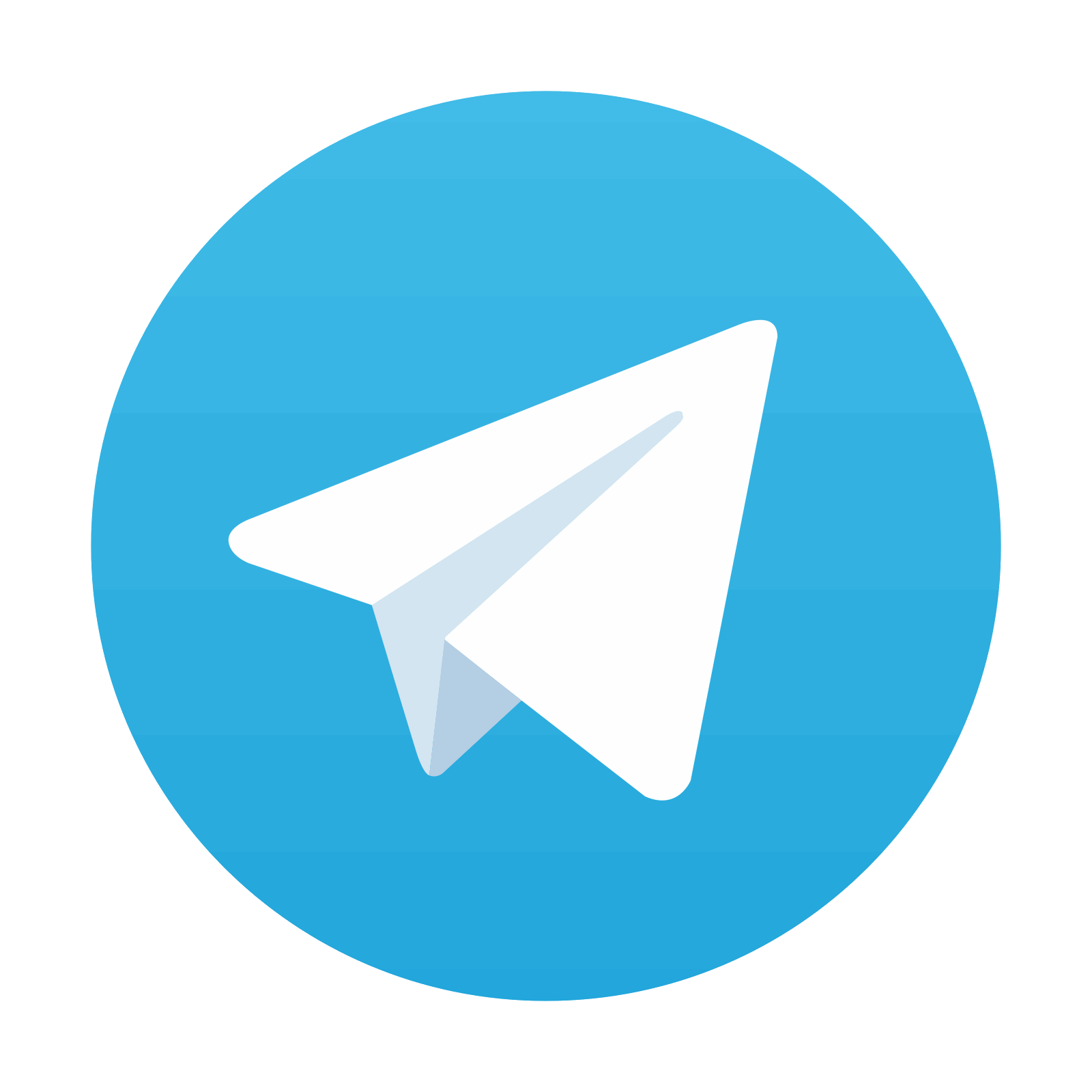
Stay updated, free articles. Join our Telegram channel

Full access? Get Clinical Tree
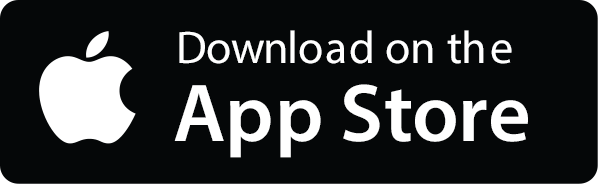
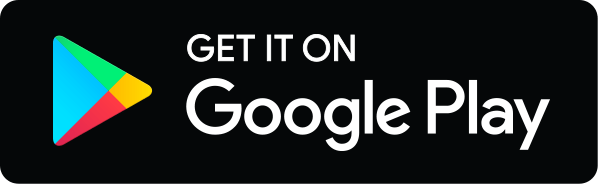