CHAPTER 1 Healing
The stages of healing are, to a large extent, purely a convenience of description, since each stage runs into another. The term phasing rather than separate stages may be more suitable. Traditionally, the initial tissue response has been described as inflammation, but some authors see inflammation as a response separate to the processes occurring at the time of injury. Van der Meulen (1982) described both in terms of the ‘reaction phase’, arguing that the classical inflammatory period is preceded by a short (10 minute) period before the inflammatory mechanism is activated. Others (Hunter, 1998), looking at the changes in strength of the healing tissue, have termed the first stage the ‘lag phase’ because tissue strength does not change. In any traumatic injury the initial stage is bleeding which is the precursor for the inflammatory cascade seen as both a vascular and cellular response.
The second stage of healing has been variously called repair, proliferation and regeneration. The tertiary stage has been termed remodelling (Van der Meulen, 1982; Kellett, 1986; Dyson, 1987). The terms injury, inflammation, repair and remodelling will be used in this text.
When describing the stage of healing, the terms acute, subacute and chronic are helpful. The acute stage (up to 48 hours following injury) is the stage of inflammation. The subacute stage, occurring between 14 and 21 days after injury, is the stage of repair. The chronic stage (after 21 days) is the stage of remodelling. The term chronic is also sometimes used to describe self-perpetuating inflammation, where the inflammatory process has restarted due to disruption or persistent irritation of the healing tissues. The total healing process occurs over a continuum, shown in Fig. 1.1.
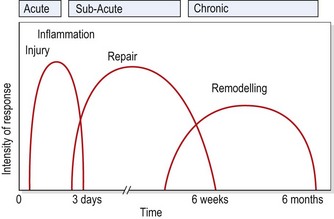
Figure 1.1 Timescale for healing.
From Oakes, B.W. (1992) The classification of injuries and mechanisms of injury, repair and healing. In Textbook of Science and Medicine in Sport (eds J. Bloomfield, P.A. Fricker and K.D. Fitch). Blackwell Scientific Publications, Melbourne. With permission.
Injury
The changes occurring at injury are affected by age (Lachman, 1988). Intramuscular bleeding, and therefore haemorrhage formation, are more profuse in individuals over 30 years of age. The amount of bleeding which occurs will be partially dependent on the vascularity of the injured tissues. A fitter individual is likely to have muscle tissue which is more highly vascularized, and therefore greater bleeding will occur with muscle injury. In addition, exercise itself will affect gross tissue responses. Muscle blood flow is greatly increased through dilatation of the capillary bed, and again bleeding subsequent to injury will be greater.
Inflammation
The next stage in the healing sequence is that of inflammation summarized in Fig. 1.2. This may last from 10 minutes to several days, depending on the amount of tissue damage which has occurred. The inflammatory response to injury is the same regardless of the nature of the injuring agent or the location of the injury itself (Hettinga, 1990). Various agents can give rise to injury, and Evans (1990a) listed physical, thermal, radiational, electrical and chemical causes.
Inflammation is not simply a feature of soft tissue injuries. It occurs when the body is infected, in immune reactions and when infarction stops blood flowing to an area. Some of the characteristics of the inflammatory response have even been described as excessive (Cyriax, 1982) and better suited to dealing with infection, by preventing bacterial spread, than healing injury (Evans, 1990a).
Heat and redness
Chemically, a number of substances act as mediators in the inflammatory process. The amines, including histamine and 5-hydroxytryptamine (5-HT or serotonin) are released from mast cells, red blood cells and platelets in the damaged capillaries and cause vessel dilatation and increased permeability lasting 10–15 minutes (Lachman, 1988). Kinins (physiologically active polypeptides) cause an increase in vascular permeability and stimulate the contraction of smooth muscle. They are found normally in an inactive state as kininogens. These in turn are activated by the enzyme plasmin, and degraded by kininases.
The initial vasodilatation is maintained by prostaglandins. These are one of the arachidonic acid derivatives, formed from cell membrane phospholipids when cell damage occurs, and released when the kinin system is activated. The drugs aspirin and indometacin act to inhibit this change—hence their use as anti-inflammatory agents in sports injury treatment. The prostaglandins E1 and E2 are two of the substances responsible for pain production, and they will also promote vasodilatation, blood vessel permeability and lymph flow (Oakes, 1992).
In a damaged capillary, however, fluid is lost and so the axial flow slows. Marginalization occurs as the slower flow rate allows white blood cells to move into the plasmatic zone and adhere to the vessel walls. This, in turn, reduces the lubricating effect of this layer and slows blood flow. The walls themselves become covered with a gelatinous layer (Wilkinson and Lackie, 1979), as endothelium changes occur (Walter and Israel, 1987).
Some 4 hours after injury (Evans, 1980) diapedesis occurs as the white cells pass through the vessel walls into the damaged tissue. The endothelial cells of the vessel contract (Hettinga, 1990), pulling away from each other and leaving gaps through which fluids and blood cells can escape (Fig. 1.3). Various substances, including histamine, kinins and complement factors, have been shown to produce this effect (Fox, Galey and Wayland, 1980; Walter and Israel, 1987).
Swelling
The normal pressure gradients inside and outside the capillary balance the flow of fluid leaving and entering the vessel (Fig. 1.4). The capillary membrane is permeable to water, and so water will be driven out into the interstitial fluid. However, because the tissue fluids usually contain a small amount of protein, and the blood contains a large amount, an osmotic pressure is created which tends to suck water back from the tissue fluid and into the capillary once more. The magnitude of this osmotic pressure is roughly 25 mmHg. At the arteriole end of the capillary the blood pressure (32 mmHg) exceeds the osmotic pressure and so tissue fluid is formed. At the venous end of the capillary the blood pressure has reduced (12 mmHg) and so, because the osmotic pressure now exceeds this value, tissue fluid is reabsorbed back into the capillary.
Protein exudation in mild inflammation occurs from the venules only and is probably mediated by histamine (Evans, 1990a). More severe inflammation, as a result of trauma, results in protein exudation from damaged capillaries as well.
Pain
Types of pain
Pain may be classified as somatogenic (acute or chronic), neurogenic or psychogenic. Chronic pain may be considered as pain which generally lasts for more than 6 weeks, while acute pain is pain of sudden onset which lasts for less than 6 weeks (Donley and Denegar, 1990).
Deep pain is normally an aching, ill-defined sensation. It usually radiates in a characteristic fashion, and may be associated with autonomic responses such as sweating, nausea, pallor and lowered blood pressure (Lynch and Kessler, 1990). Pain referral corresponds to segmental pathways, most often dermatomes. The extent of radiation largely depends on the intensity of the stimulus, with pain normally radiating distally, and rarely crossing the mid-line of the body (Cyriax, 1982).
Neurogenic pain is different again. Compression of a nerve root gives rise to ill-defined tingling, especially in the distal part of the dermatome supplied by the nerve. This is a pressure reaction, which quickly disappears when the nerve root is released. Greater pressure causes the tingling to give way to numbness. Compression or tension to the dural sleeve covering the nerve root gives severe pain, generally over the whole dermatome. In contrast, pressure on a nerve trunk usually causes little or no pain, but results in a shower of ‘pins and needles’ as the nerve compression is released. Pressure applied to a superficial nerve distally gives numbness and some tingling, with the edge of the affected region being well defined (Cyriax, 1982).
Irritability
Irritability may be defined as ‘the vigour of activity which causes pain’ (Maitland, 1991). It is determined by the degree of pain which the patient experiences, and the time this takes to subside, in relation to the intensity of activity that brought the pain on in the first place. The purpose of assessing irritability is to determine how much activity (joint mobilization, exercise, etc.) may be prescribed without exacerbating the patient’s symptoms.
Treatment note 1.1 Pain description in examination
During both the subjective examination and the objective examination (see Treatment note 1.5, p. 24) the patient will usually describe pain. Both the type (nature) of pain and its behaviour are important factors in making an accurate clinical diagnosis, and a number of factors should be considered:






The description of pain itself may indicate the structure causing it (see Table 1.1) and the behaviour of the pain on physical examination clarifies the picture.
Table 1.1 Pain descriptions and related structures
Type of pain | |
---|---|
Cramping, dull, aching, worse with resisted movement | Muscle |
Dull, aching, worse with passive movement | Ligament, joint capsule |
Sharp, shooting | Nerve root |
Sharp, lightning-like, travelling | Nerve |
Burning, pressure-like, stinging, with skin changes | Sympathetic nerve |
Deep, nagging, poorly localized | Bone |
Sharp, severe, unable to take weight | Fracture |
Throbbing, diffuse | Vasculature |
Source Magee (2002) and Petty and Moore (2001) with permission.
Recording pain
The intensity of pain may be recorded on a visual analogue scale (VAS). The patient is asked to indicate the pain description or number which best represents their pain. Where a 10 cm line is used the distance from the left of the scale to the point marked by the patient may be measured in millimetres and used as a numerical value (Fig. 1.5).
Red flags
It is important for the therapist to appreciate when pain and other symptoms may suggest serious pathology which requires medical investigation—so called ‘red flags’ (Table 1.2). Where the patient has persistent pain and is generally unwell, the indication is that a pathology other than a musculoskeletal condition exists. In addition, changes in bladder and bowel habits, alteration in vision or gross changes in gait all require further investigation.
Table 1.2 Red flags in sport examination indicating medical investigation
System/possible pathology | Pain behaviour |
---|---|
Cancer | Persistent night pain |
Constant (25 hour) pain | |
Unexplained weight loss (e.g. 4–6 kg in 10 days) | |
Loss of appetite | |
Unusual lumps or growths | |
Sudden persistent fatigue | |
Past history of carcinoma | |
Cardiovascular | Shortness of breath |
Dizziness | |
Pain or feeling of heaviness in the chest | |
Pulsating sensations in the body | |
Discolouration in the feet | |
Persistent swelling with no history of injury | |
Gastrointestinal/genitourinary | Frequent or severe abdominal pain |
Frequent heartburn or indigestion | |
Frequent nausea or vomiting | |
Change in bladder or bowel habits | |
Unusual menstruation | |
Neurological | Changes in hearing |
Frequent or severe headache | |
Problems in swallowing or changes in speech | |
Gait disturbance, or problems with balance/ coordination | |
Drop attacks (fainting) | |
Sudden weakness |
Source Magee (2002) and Waddell, G., Feder, G. and Lewis, M. (1997) Systematic reviews of bed rest and advice to stay active for acute low back pain. British Journal of General Practice, 47, 647–652. With permission.
Pain production
The pain receptors are supplied by a variety of different nerve fibres. Skin receptors are supplied by thinly myelinated (A delta) fibres which carry ‘fast’ pain and respond to strong mechanical stimuli and heat above 45°C (Low and Reed, 1990). They give the initial sharp well-localized pain feeling (pinprick). The function of fast pain is to help the body avoid tissue damage and it often provokes a flexor withdrawal reflex.
Impulses from free nerve endings found in deeper body tissues are carried by non-myelinated C fibres. This is ‘slow’ pain, which tends to be aching and throbbing in nature, and poorly defined. Its onset is not immediate, and the sensation it produces persists after the pain stimulus has gone. The function of slow pain seems to be to enforce inactivity and allow healing to occur and it is therefore often associated with muscle spasm. The C fibres respond to many different types of stimuli and, as such, are said to be ‘polymodal’. However, they are most sensitive to chemicals released as a result of tissue damage. Histamine, kinins, prostaglandins E1 and E2, and 5-HT have all been implicated in this type of pain production during inflammation (Walter and Israel, 1987; Lachman 1988).
Articular neurology
The type III fibres are found in the joint ligaments, and are again high threshold dynamic mechanoreceptors, but are slow adapting. These receptors monitor the direction of movement, and have a ‘braking’ effect on muscle tone if the joint is moving too quickly or through too great a range of motion. The type IV receptor is the nociceptor described above. Table 1.3 provides a synopsis of the various movement categories to which the receptors respond.
Table 1.3 Function of joint receptors
Function | Receptor |
---|---|
Static position | Type I |
Speed of movement | Type I |
Change in speed | Type II |
Direction of movement | Types I and II |
Postural muscle tone | Type I |
Tone at initiation of movement | Type II |
Tone during movement | Type II |
Tone during harmful movements | Type III |
Adapted from Hertling, D. and Kessler, R.M. (1990) Management of Common Musculoskeletal Disorders. JB Lippincott, Philadelphia.
Pain pathways
Seventy percent of the C fibres (slow pain) enter the spine via the dorsal root, while 30% of the fibres enter via the ventral root. The C fibres synapse with second order neurones in the substantia gelatinosa (SG) of the cord and these neurones ascend in the anterolateral funiculus on the opposite side of the cord (Fig. 1.6). From here they travel via the reticular formation to the intralaminar nuclei of the thalamus. The neurones synapse here once more and travel to the prefrontal region of the cerebral cortex. Some of the C fibres travel to the limbic system (cingulate gyrus) and generate emotional responses to pain (described as anxiety, fear and dread). C fibre pain is therefore poorly localized with a large emotional effect (White, 1999).
Fast pain is registered in the parietal lobe and visceral pain in the insular cortex.
Pain relief mechanisms
Three concepts of pain control are generally used within physiotherapy:
The pain gate
The pain gate theory (Melzack and Wall, 1965) proposed that pain perception was regulated by a ‘gate’ which could be opened or closed. When stimulated, mechanoreceptors in the skin send impulses via A beta fibres to the posterior horn of the cord. Here, collateral branches are given off. These collaterals affect A delta and C pain fibres in the substantia gelatinosa (SG), reducing their excitability by presynaptic inhibition. Stimulation of A delta fibres by low intensity, high frequency transcutaneous electrical nerve stimulation (TENS) (100–200 Hz) will therefore reduce pain through this gating mechanism (Fig. 1.7).
Descending inhibition
Descending inhibition occurs when A delta fibres activate a chain of neurones which travel down the length of the spinal cord. Two separate systems are generally said to be involved (White, 1999), one involving serotonin, the other noradrenaline (norepinephrine). In the first, fibres from the periaqueductal grey matter (PAG) of the midbrain travel to the nucleus raphe magnus and then to the stalked cells in the dorsal horn of the spinal cord. In these cells, serotonin is released, which in turn causes the release of enkephalin to inhibit the cells of the SG. In the second system, the arcuate nucleus of the hypothalamus activates nuclei in the brainstem. Descending fibres in turn release noradrenaline (norepinephrine) into the dorsal horn of the cord to again inhibit the SG. Stimulation at frequencies above 50 Hz may affect this system (De Domenico, 1982).
Treatment note 1.2 Dry needling
Both painful points (trigger points) and classic acupuncture points may be used. Classic acupuncture points are needled to specific depths described in acupuncture literature (see Norris, 2001). Trigger points may be either needled superficially to a depth of 0.5 cm (Baldry, 1998) or up to 8–10 cm (Gunn, 1996), depending on the mechanism being used. Superficial needling is said to activate A delta nerve fibres responsible for acupuncture pain relief, while deep needling is said to reduce pain but also induce healing through the production of platelet derived growth factor (PDGF) to stimulate new collagen formation (Gunn, 1996).
It should be noted that dry needling carries risks, and requires postgraduate training. Deep needling of the type used in intramuscular stimulation (IMS) in particular is a technique which involves highly specialist training (see Training section at the end of this box).
Technique
The needles are normally inserted using a plastic guide tube which is slightly shorter than the needle (Fig. 1.8). The guide tube is placed on the skin with the needle within it and the needle inserted through the skin surface with a small, brisk tap. Once the needle is inserted the guide tube is removed, and the needle pressed in further to the required depth (Fig. 1.9). Throughout this procedure, sterile practice must be maintained. To guard against infection, each needle is only used once and the shaft of the needle should not be touched.
The needle is often manipulated by rotation, scraping or flicking to increase the sensation felt by the patient—known as sensory propagation along channels or deqi in Oriental medicine. The needle may be inserted perpendicular to the skin or at oblique and transverse angles (Fig. 1.10). Once the needle is withdrawn the acupuncture point may be pressed with a cotton bud or probe to reduce the likelihood of bruising.
Management of inflammation
Chronic inflammation
Chronic inflammation has been shown to have a low concentration of growth factors and a high concentration of protease (Hom, 1995). Adding growth factor to a chronic wound has been shown to improve healing in a number of soft tissues. Platelet derived growth factor has been used to treat ligaments and tendons in general (Evans, 1999) whilst insulin like growth factor (IGF-1) has been used with the achilles tendon (Kurtz, Loebig and Anderson, 1999) and articular cartilage (Nixon, Fortier and Williams, 1999).
Repair
Inflammation may continue for 5 days, but with minor trauma it is usually complete by the third day after injury (Evans, 1980). Following this, tissue repair can take place. Repair is by resolution, organization or regeneration, depending on the severity of the injury and the nature of the injured tissues. A minor injury will result in acute inflammation as described above, and the phagocytic cells will clear the area. If there is little tissue damage, the stage of resolution will result in a return to near normal (Lachman, 1988). True resolution rarely occurs with soft tissue injuries, but is more common with inflammatory tissue reactions such as pneumonia.
The individual fibrils form into parallel bundles lying in the direction of stress imposed on the tissue. If no movement occurs to stress the collagen bundles, they are laid down in a haphazard and weaker pattern (Cyriax, 1982). Controlled movement causes the fibrils to align lengthways along the line of stress of the injured structure (Burri, Helbing and Spier, 1973). Variation in longitudinal fibre alignment will determine the stress–strain response of the tissue to loading (Fig. 1.11). Where fibre alignment is parallel to the tissue body, the steep stress–strain curve (C) indicates that less deformation will occur for a given tissue loading. The tissue is therefore ‘stronger’.
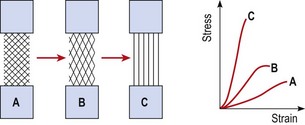
Figure 1.11 Relationship between fibre orientation and stress–strain response.
From Oakes, B.W. (1992). The classification of injuries and mechanisms of injury, repair and healing. In Textbook of Science and Medicine in Sport (eds J. Bloomfield, P.A. Fricker and K.D. Fitch). Blackwell Scientific Publications, Melbourne. With permission.
In some tissue full regeneration occurs, damaged cells being replaced by functioning normal tissue. Fractured bone exhibits this property, as do torn ligaments and peripheral nerves providing conditions are suitable (Evans, 1990b).
Remodelling
The remodelling stage overlaps repair, and may last from 3 weeks to 12 months (Kellett, 1986). During this stage, collagen is modified to increase its functional capacity. Remodelling is characterized by a reduction in the wound size, an increase in scar strength, and an alteration in the direction of the collagen fibres (Van der Meulen, 1982).
Contraction of granulation tissue will occur for as long as the elasticity of the fibres will allow (Van der Meulen, 1982). Fibroblast cells transform into myofibroblasts which then form intercellular bonds. These contain contractile proteins (actomyosin) and behave much like smooth muscle fibres.
Three weeks after injury, the quantity of collagen has stabilized (Van der Meulen, 1982) but the strength of the fibres continues to increase. Strength increases are a result of an expansion in the number of cross-bonds between the cells, and the replacement collagen cells themselves. There is a continuous turnover of collagen, a process influenced by a number of factors, including the age of the patient, the type of tissue injured, the quantity of scar tissue present, the site and direction of the scar and external forces (Van der Meulen, 1982; Frank et al., 1983).
Final collagen fibre alignment should match the tissue function (Fig. 1.12). Fibres within a ligament will respond to a range of motion exercises which tense the ligament rhythmically. This may cause mild discomfort (VAS 2−3) but not pain (VAS 7−8). Pain is an indication of tissue damage indicating that the healing process has reverted back from the remodelling stage to the inflammatory stage. Fibres within muscle respond similarly, but to force transmission encountered by active and light resisted exercise during rehabilitation.
Matching treatment to the healing timescale
The tensile strength of injured soft tissue will reduce substantially after injury due to mechanical damage to the tissues. By the first postinjury day, tensile strength may have reduced by some 50%. Although healing begins immediately, collagen is not laid down until the fifth postinjury day (Garrett, 1990). The period between injury and the beginning of collagen synthesis has been described as the ‘lag phase’ (Fig. 1.13). Manual therapy techniques applied in this period should be aimed at pain resolution and oedema reduction. Only when collagen synthesis begins should therapy aim to prevent adhesions and align collagen fibres in the direction of stress.
Treatment note 1.3 Influencing the mechanical properties of healing tissue
Soft tissue manipulation
The use of deep transverse frictional massage (DTF) has been claimed to assist soft tissue healing. By applying shear and gliding movements to an injured tendon, ligament or muscle, tensile strength of the healing scar may be improved and adhesions reduced. Gentle DTF applied in the acute phase of healing could increase the rate of phagocytosis by inducing agitation of tissue fluid. In the chronic stage of healing the therapeutic movement produced by DTF is said to soften and mobilize adhesions (Kesson and Atkins, 1998).
Specific soft tissue mobilization
Specific soft tissue mobilization (SSTM) is a technique pioneered by Hunter (1998). The procedure involves tensioning the tissue using physiological joint movement and accessory joint movement and adding a dynamic soft tissue mobilization. In the acute phase of healing, SSTM is claimed to influence the mechanical properties of healing tissue by altering collagen and ground substance synthesis.
A sustained load of five repetitions of a 30-second hold is used in a treatment session and the patient performs home exercise by self-stretching for three repetitions of a 30-second hold every 3–4 hours. Using the Achilles tendon as an example (Fig. 1.14), the mobility of the tendon is assessed by subjecting it to shearing forces, beginning distally and moving proximally. The aim is to fix the proximal segment of the tendon with the fingers and then move the tendon in the opposite direction with the fingers of the other hand. The shearing motion is moved up the tendon progressively, assessing range and quality of movement.
Eccentric loading
Eccentric loading has been used extensively for rehabilitation of the Achilles tendon. It is claimed that the controlled lengthening of the tendon during eccentric actions increases the tensile strength of the tendon (Stanish, Rubinovich and Curwin, 1986; Kannus, 1997) and allows for more storage of elastic energy in the stretch shorten cycle. In addition, eccentric loading may prepare the tendon for rapid unloading. The sudden release of force in this way produces shearing forces within the tendon which could conceivably break up adhesions within the Achilles itself (Curwin, 1994).
Individual tissue response to injury
Synovial membrane
The synovium consists of two layers, the intima, or synovial lining, and the subsynovial (subintimal) tissue. The intimal layer is made up of specialized cells known as synoviocytes, arranged in multiple layers. Two types of synoviocytes are present, type A cells, which are phagocytic, and type B cells, which synthesize the hyaluronoprotein of the synovial fluid. The two types are not distinct, however, and appear to be functional stages of the same basic cells (Hettinga, 1990).
The blood vessels of the joint divide into three branches, one travelling to the epiphysis, the second to the joint capsule and the third to the synovial membrane (Paget and Bullough, 1981). From here the vessels of the subsynovium are of two types. The first is thin walled and adapted for fluid exchange, and the second thick walled and capable of gapping to allow particles, especially nutrients, to pass through.
Response to injury
With minor trauma the synovium is not microscopically disturbed, but will instead suffer a vasomotor reaction (Hettinga, 1990). The synovium will dilate and fluid filtration increases. Protein leaks into the interstitium, changing the osmotic pressure and causing local oedema and joint exudation. This process constitutes a post-traumatic synovitis.
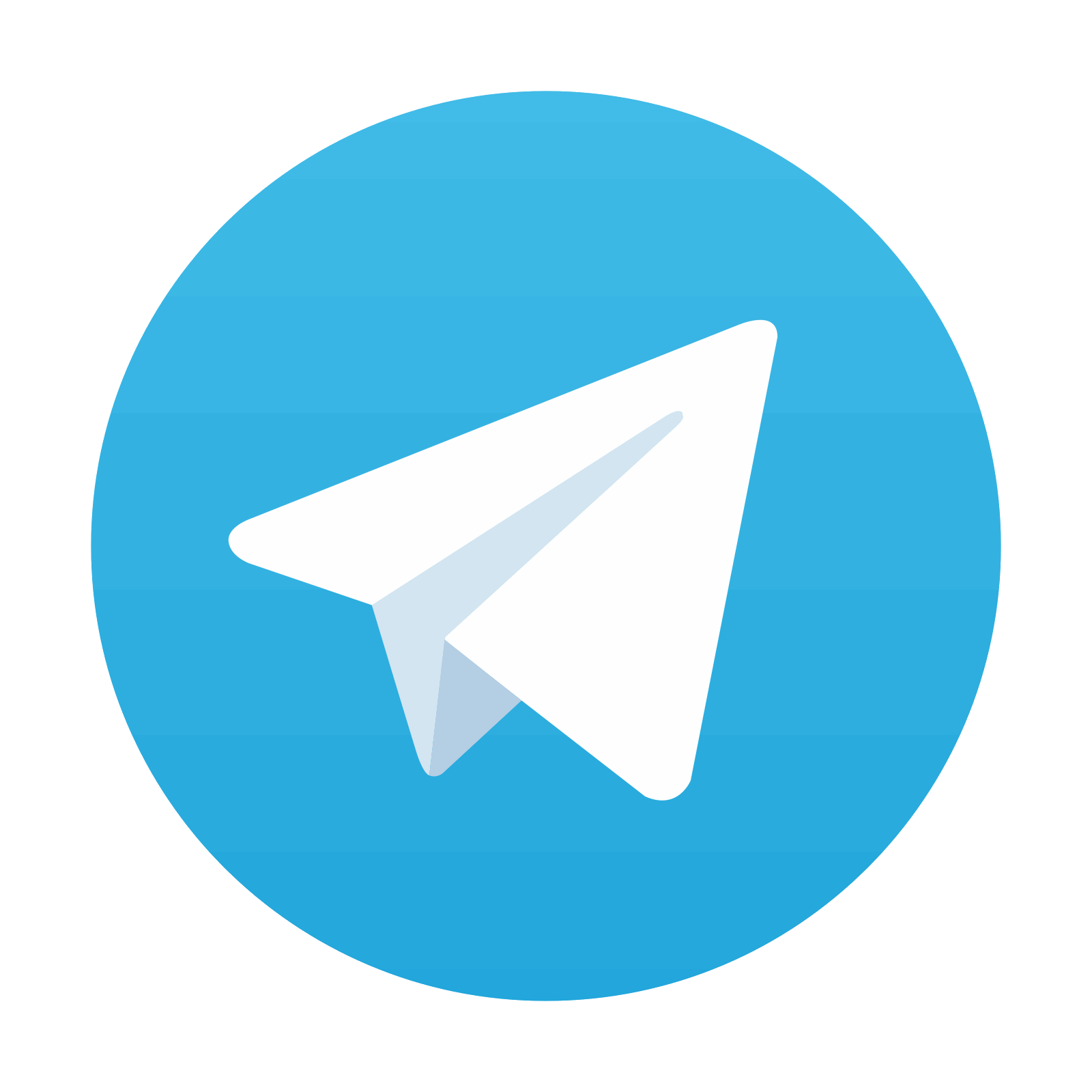
Stay updated, free articles. Join our Telegram channel

Full access? Get Clinical Tree
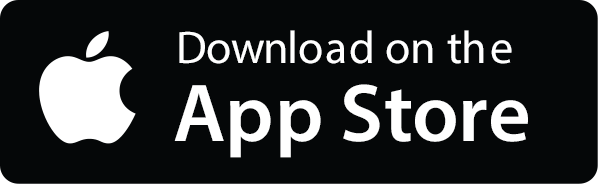
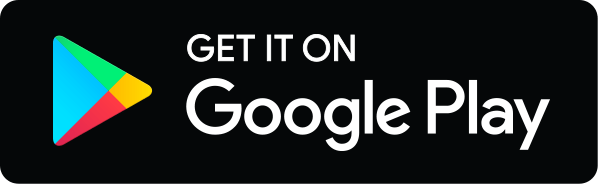