CHAPTER 4 Physical training and injury
Principles of training
Supercompensation
The process of adaptation to an imposed demand (stress) is succinctly described by the general adaptation syndrome or GAS (Seyle, 1956). Initially there is an alarm reaction or ‘shock’. This may last for several weeks, and during this time an athlete’s performance will be impaired, leading to stiffness. Over time, the body adapts to the stress and the athlete enters the resistance phase which is the process of ‘supercompensation’, enabling the body to cope more effectively with stress at that imposed level.
Overtraining syndrome
Training involves stressing the body so that it overcompensates and gains a training effect. Some fatigue after exercise is therefore desirable and this type of acute fatigue leads to an increase in performance. However, if the training volume is too great, or the recovery period between training bouts too brief, athletes will overreach giving a temporary reduction in performance. Where overreaching (OR) is marked, stagnation will result in a brief performance reduction. This may occur for example after a hard training camp, and the effect would be positive providing recovery is adequate. Overreaching of this type is termed ‘functional OR’. Continuing the increase in training intensity without allowing recovery will lead to a reduction in performance called non-functional OR. A short-term decrement in performance occurs which allows the athlete to recover within a 2-week period (Lehmann et al., 1999). If recovery is not adequate and training continues, the progression is to overtraining. Recovery in this case may takes months or in some cases years (Meeusen et al., 2006).
Overtraining syndrome (OTS) occurs when the body is subjected to stresses beyond its capacity to adapt. Lack of adaptation to training leads in turn to a reduction in sports performance and a number of important potential health concerns. The symptoms of OTS are initially similar to those of hard training, such as muscle aching and fatigue. However, the experienced coach and athlete can detect when these changes are greater than normal. Several physiological effects are thought to underlie OTS, including autonomic nervous system changes, alteration in endocrine response, suppression of immune function and variation in brain neurotransmitters (Wilmore, Costill and Kenney, 2008). Alteration in sympathetic nervous system drive is seen in athletes who emphasize high intensity resistance training giving increased heart rate, blood pressure, and basal metabolic rate, loss of appetite and decreased body mass.
Symptoms of OTS
One of the adaptations to normal training is a change in the hypothalamic pituitary axis (HPA) characterized by an increase in the ACTH/cortisol ratio during the post workout recovery period. In OTS there is a change in the rise in ACTH following exercise and this has been used to identify non-functional OR and OTS. Using two exercise bout tests Meeusen et al. (2004) were able to demonstrate large increases in hormonal release following the first exercise bout but suppression following the second. Time to fatigue tests which are specific to an athlete’s sport have also been used to detect OTS (Halson and Jeukendrup, 2004). Typically athletes suffering from OTS are able to begin an exercise session performing normally but then suffer from an unexplained performance drop. Fatigue tests are therefore more predictive than incremental tests (Meeusen et al., 2006).
Mood state questionnaires are very useful in the identification of OTS as negative affective states characterize the condition. Questionnaires such as the recovery-stress questionnaire (RestQ-Sport) may be used but athletes should ideally be tracked throughout their training to identify a baseline to show deviation. This is a 76-item questionnaire which assesses the physical and mental impact of training (Kellman, 2001). Heart rate variability (HRV) has also been used as an assessment tool of OR. HRV increases with heightened parasympathetic tone and has been shown to be significantly elevated following chronic training (Meeusen et al., 2006).
Many athletes describe an increase in URT infections following hard training—the so called ‘open window’ effect. This effect seems to be more prevalent with OR and OTS. A 2-week period of intense training has been shown to reduce bacterial defense response (bacterially stimulated neutrophil degradation) by 20% (Robson et al., 1999) and a 1-week intense programme to lower T-cell count (Lancaster et al., 2004)
The initial management OTS is dependent on identifying OR. This is made easier by having the athlete keep a training log (Table 4.1) so that changes in response to their baseline measure may be recognized early and appropriate action taken. Where OR is suspected it is vital that training volume be reduced, and in more severe cases total rest may be called for over a period of many weeks. Prevention focuses on periodization of training to vary training variables including frequency, intensity, time and type (FITT). High quality diet and adequate sleep play a pivotal role in exercise recovery and the prevention of OTS.
FITT: frequency, intensity, time, type; RPE: rating of perceived exertion; UTI: upper respiratory tract infection; MSK: musculoskeletal.
Halson S., Jeukendrup A. Does overtraining exist? An analysis of overreaching and overtraining research. Sports Medicine. 2004;34:967-981.
Kellmann M., Kallus K.W. Recovery-Stress Questionnaire for athletes: User manual. Champaign, IL: Human Kinetics Publishers; 2001.
Lancaster G.I., Halson S.L., Khan Q., Drysdale P., Jeukendrup A. The effects of acute exhaustive exercise and intensified training on type 1/ type 2 T cell distribution and cytokine production. Exercise Immunology Review. 2004;10:91-106.
Lehmann M., Foster C., Gastmann U., et al. Overload, performance incompetence, and regeneration in sport. New York: Plenum; 1999.
Meeusen R., Duclos M., Gleeson M., Rietjens G. Prevention, diagnosis and treatment of the Overtraining Syndrome. ECSS position statement. European Journal of Sports Science. 2006;6(1):1-14.
Meeusen R., Piacentini M.F., Busschaert B., Buyse L. Hormonal responses in athletes: the use of a two bout exercise protocol to detect subtle differences in overtraining status. European Journal of Applied Physiology. 2004;91:140-146.
Robson P.J., Blannin A.K., Walsh N.P. The effect of an acute period of intense interval training on human neutrophil function and plasma glutamine in endurance trained male runners. Journal of Physiology. 1999;515:84-85P.
Wilmore J.H., Costill D.L., Kenney W.L. Physiology of sport and exercise, fifth ed. Champaign, IL: Human Kinetics Publishers; 2008.
Progressive exercise
In addition to a minimum intensity, the training load must be continued for a certain time. High intensity training which is too brief may not allow time for the physical adaptations required by the body. The frequency of training—that is, how often it is carried out—is also important. Training is a stimulus which causes an anabolic adaptation. This adaptation will take time, and so adequate recovery must be allowed between training sessions for the body tissues to modify themselves. The type of training will dictate the type of tissue adaptation which occurs, a principle known as specificity (McCafferty and Horvath, 1977) (see below).
Training effects are not permanent. The motor system adapts to the level (overload) and type (specificity) of stress that is imposed on it. If the stress is removed, and training ceases, the motor system will again adapt to the new, now lower, level of stress, and detraining will occur. This transient nature of training adaptation is known as the reversibility principle (Thorstensson, 1977; Enoka, 1994).
When training for aerobic (cardiopulmonary) fitness or stamina, exercise intensity may be assessed by measuring heart rate or maximal oxygen uptake (VO2 max). The American College of Sports Medicine (1978) recommended the quantity and quality of exercise required to develop and maintain aerobic fitness and body composition. A training frequency of 3–5 days per week is required, at an intensity of 60–90% of the maximum heart rate reserve, or 50–85% VO2 max. This should be carried out for a duration of 15–60 minutes, and be continuous or rhythmical in nature. These recommendations were later updated to include the provision of resistance training, flexibility and weight loss (ACSM, 1990, 2002). For strength gains, one set of 8–12 repetitions was recommended, with 8–10 exercises for the major muscles groups, for 2 days per week. A balanced flexibility programme should include both static and dynamic range of motion exercise to work the major muscle/tendon groups. Each stretch should be held for at least 10–30 seconds, and four repetitions should be used for each group two to three times per week. To achieve significant weight loss, hours of moderate exercise with an energy expenditure of at least 2000 calories per week is recommended. To achieve this, either continuous or accumulative exercise may be used at an exercise intensity of 55–69% maximal heart rate. Accumulated daily duration should be 30–40 minutes per day (Table 4.2).
Table 4.2 ACSM guidelines for maintaining fitness in apparently healthy individuals
Cardiovascular | |
Frequency | 3–5 times per week |
Intensity | 55–90% HRmax |
Time | 20–60 min |
Type | Large muscle groups. Rhythmic and continuous activity |
Muscular | |
Frequency | 2–3 times per week |
Intensity | 8–10 exercises 1 set of 8–12 repetitions to volitional fatigue 75% 1 RM resistance |
Time | 20 minutes |
Type | Resistance training for major muscle groups |
Flexibility | |
Frequency | 3 times per week |
Intensity | 3−5 repetitions for each exercise Maintain at point of mild discomfort |
Time | 10–30 second hold |
Type | Static stretch for major muscle groups |
HR max: maximal heart rate; 1 RM: maximum resistance lifted for single repetition.Source ACSM (1978, 2002).
Importantly each of us is different, and will respond differently to training. This principle of Individuality underlies successful exercise prescription. Variation in tissue adaptation, neural, cardiopulmonary and endocrine changes, and psychology dictate that we will all respond slightly differently to exercise. Individuality explains why when two people begin a gym programme, for example, one may progress quite rapidly (high responder) while the other may struggle to make gains (low responder). The main reason for individuality is hereditary. A study of a 20-week endurance training programme using identical twins (Prud’homme et al., 1984) showed a similar training response for each twin pair, but a substantial variation across subjects of maximal aerobic power improvement (0−40%). Assessing the difference in training response (VO2 max) using family members (Bouchard and Rankinen, 2001) has shown that high or low responders tend to be clustered within families, again representing a genetic and/or familial tendency.
Fitness
Physical fitness has been defined as a set of attributes that relate to the ability of people to perform physical activity (McArdle et al., 1991), or the ability of a person to function efficiently and effectively to enjoy leisure, to be healthy, to resist hypokinetic disease and to cope with emergency situations (Kent, 1994).
Fitness can be thought of as a continuum from optimal fitness at one side through average fitness to complete lack of fitness and death (Fig. 4.1). The exact components of fitness required to make an individual optimally efficient and effective will be determined largely by the physical activity to be performed.
The benefits of exercise are numerous (Table 4.3). However, as we have seen (Treatment Note 4.1) there is a balance between training sufficiently hard to gain the effects of overload, but not so hard that the athlete overreaches (OR) in the short term or eventually progresses to overtraining (OTS). Many athletes will be familiar with the symptom of staleness, and of an increased incidence of UTI.
Table 4.3 Benefits of regular exercise
Source US Department of Health and Human Sciences (1999) Physical Activity and Health. Report of the Surgeon General. http://www.cdc.gov.
Infection of this type occurs because although training in general has been shown to enhance immune function, following intense training the immune system is depressed. This temporary depression leaves the athlete susceptible to infection, and is called the ‘open window’. It is thought that intense exercise changes adrenocorticotropic hormone (ACTH) and cortisol concentrations and has a knock-on effect on blood glucose concentration, negatively affecting the immune system (Nieman and Pedersen, 1999). Moderate exercise does not have this effect, and the window remains closed giving the long term immune system the benefits of exercise without short term immunosuppression.
Fitness components
The fitness components may be conveniently defined as ‘S’ factors (Table 4.4). The term ‘stamina’ is used to encompass both cardiopulmonary and local muscle endurance. Cardiopulmonary endurance is associated with a reduced risk of coronary heart disease (Ashton and Davies, 1986), and local muscle endurance is a factor in any sustained activity, especially joint stability. Suppleness (flexibility) and strength (see below) are concerned with the health of the musculoskeletal system, to maintain both range of movement and joint integrity. Speed (rate of movement) and power (rate of doing work) are both needed in later stage rehabilitation as part of proprioceptive training. Skill training is important, not just for sports specific actions, but for the skill of individual movement such as scapulohumeral rhythm or gait re-education, for example.
Table 4.4 ‘S’ factors of fitness
Factor | Concept |
---|---|
Stamina | Cardiopulmonary and local muscle endurance |
Suppleness | Passive and active flexibility |
Strength | Isometric, isotonic (concentric and eccentric) isokinetic strength |
Speed | Speed (rate of movement) and power (rate of doing work) |
Skill | Motor skill |
Specificity | Overload must match tissue adaptation required |
Spirit | Psychological aspects of injury, including illness behaviour |
The term ‘spirit’ covers the psychological effects of exercise as discussed below.
Psychological effects of exercise
Enhanced well-being
Athletes often claim that exercise makes them ‘feel good’, and the ‘runners’ high’ is a widely reported phenomenon. Reductions in stress and anxiety have been reported, lasting for between 2 and 5 hours after the cessation of training (Morgan, 1985), and decreased depression has been demonstrated as a result of 6–20-week exercise programmes (Greist et al., 1979). In addition, altered states of consciousness have been described following distance running (Mandell, 1979). Weight training programmes have been shown to enhance self-concept in both male (Dishman and Gettman, 1981; Tucker, 1982) and female (Brown and Harrison, 1986) athletes. Three theories exist to explain these phenomena: the distraction hypothesis, and the production of monoamines and endorphins.
How exercise makes an athlete feel better
The distraction hypothesis proposes that participation in vigorous exercise distracts the athlete from stress. Comparisons between exercise, meditation and distraction show similar reductions in state anxiety, but the effect resulting from exercise appears to last longer (Morgan, 1985).
Depression is also affected by exercise. Reductions in the monoamine chemicals noradrenaline (norepinephrine) and serotonin (5-HT) are associated with depressed states in humans, and these same chemicals have been shown to increase in rats subjected to chronic exercise (Brown et al., 1979). Increases in the release of endorphins and enkephalins, or slowing of the dissociation rates of these chemicals has also been proposed (Pert and Bowie, 1979). By measuring plasma levels of these chemicals or using opiate antagonists to neutralize them, researchers have demonstrated some association between exercise and endorphins (Farrell et al., 1983).
Exercise addiction
The experience of exercise for an athlete, and the way in which this fits into the rest of his or her life, is one factor which determines whether or not an exercise becomes addictive (Crossman, Jamieson and Henderson, 1987). An individual’s need for exercise can be either positive or negative. Positive addiction exists when an athlete receives some psychological or physical benefit from an activity, and is able to control the activity.
The negatively addicted athlete is controlled by the activity and will experience severe negative effects (withdrawal) with a missed exercise bout. Such athletes often engage in an activity at the expense of their health or at the expense of other factors, such as relationships and career prospects. The negatively addicted athlete may be failing to gain approval from significant others and may harbour feelings of inadequacy or unattractiveness. This type of athlete often exercises alone or in isolation from the group. They experience feelings of enhanced self-concept and even euphoria during exercise. Importantly, such individuals are more likely to ignore pain or injury and work through this to complete a workout. In the same vein, they tend to be anxious if a workout is missed and almost appear to suffer ‘withdrawal symptoms’ (Table 4.5).
Table 4.5 Characteristics of exercise addiction
The athlete may: |
Adapted from Glasser, W. (1976) Positive Addiction. Reprinted by permission of HarperCollins Publishers Inc. and Anshel, M.H. (1991) A psycho-behavioral analysis of addicted versus non-addicted male and female exercisers. Journal of Sport Behavior, 14 (2), 145–154.
Warm-up
Many athletes conscientiously warm-up in the belief that they will protect themselves against injury, and enhance their sporting performance. While neither of these beliefs have been conclusively proven, there is mounting evidence in the literature to suggest that both may contain elements of truth. Two studies are of particular note. Wedderkopp, Kaltoft and Lundgaard (1999) found that warm-up significantly reduced both traumatic and overuse injury frequency. Players in the control group (non-warm-up) were found to be 4.9 times more likely to become injured than those who warmed up. Looking at knee and ankle injury incidence in hard ball players, Olsen, Myklebust and Engebretsen (2005) studied 1837 youth handball players in Norway and found a significantly lower incidence of injury in the warm-up group (0.5 injuries per 1000 player hours) compared to the control group (0.9 injuries per 1000 player hours). These same authors concluded that a warm-up programme reduced the incidence of injury by 50%.
Warm-up types
Warm-up may be either passive, involving an external heat source, or active, involving body heat. An active warm-up, in turn, may be general, using the whole body, or specific, working only those body parts to be used in competition, and studies have shown improvements from each (Table 4.6).
Table 4.6 Some historical studies on efficiency of warm-up
Reference | Warm up type | Result |
---|---|---|
Carlile (1956) | Passive | Improvements in swimming times after hot showers (8 minutes at 40°C) |
Davies and Young (1983) | Passive | Warmed the triceps surae muscles using hot water baths. Showed increases in peak power output with cycling and jumping tasks |
Sargeant (1987) | Passive | Water baths. Showed increases in peak force and power of 11% after heating, and reductions of up to 21% after cooling |
Richards (1968) | Active—general | Stool stepping before vertical jump task. 1- and 2-min warm-ups improved performance by 23%. 4 min had no effect. 6 min warm-up reduced performance by 27% |
DeVries (1959) | Active—specific | Compared passive (hot showers and massage), active (calisthenics) and specific (swimming) warm-up prior to swimming task. Significant improvement only after specific warm-up |
Effects of warm-up
Cardiovascular changes
A warm-up of sufficient intensity will cause an alteration in regional blood flow. When resting, only 15–20% of the total blood flow goes to the skeletal muscles, but after about 10 minutes of general exercise this figure is increased to 70–75% (Renstrom and Kannus, 1992). During a warm-up, blood flow is increased to active muscles and reduced to visceral tissues earlier than would occur without a warm-up. Increased blood flow causes the delivery of nutrients and removal of metabolic wastes to be enhanced.
Barnard et al. (1973) examined the effects of sudden strenuous exercise on men with no symptoms of cardiac problems. Each subject ran vigorously on a treadmill for 10–15 seconds without a warm-up. In 70% of these subjects, abnormal changes were seen on an ECG trace, indicative of subendocardial ischaemia. These changes were reduced, or even abolished, when a warm-up was performed before activity. Similarly, the effect of sudden onset exercise on blood pressure was improved. Average systolic blood pressures of 168 mmHg were seen without warm-up and these reduced to 140 mmHg when warm-up preceded exercise.
One of the reasons for these changes is that the adaptation of the coronary blood flow to strenuous exercise is not instantaneous. The cardiac output is unable to increase quickly enough to meet the demands of sudden high intensity work (Astrand and Rodahl, 1986), and a warm-up gives the cardiovascular system time to respond.
Tissue temperature
The ability to perform physical work is improved by elevated temperature (Bergh and Ekblom, 1979a). Warm-up prior to maximal exercise will enable the adaptations necessary for these changes to occur sooner.
Oxygen dissociation from haemoglobin is more rapid and complete, and oxygen release from myoglobin is greater at higher temperatures (Astrand and Rodahl, 1986). The critical level of various metabolic processes is lowered, causing an acceleration in metabolic rate and a more efficient usage of substrates. Muscle contraction is more rapid and forceful (Bergh, 1980). The sensitivity of nerve receptors and speed of transmission of nervous impulses are both increased as temperature rises (Astrand and Rodahl, 1986). This more rapid transmission of kinaesthetic signals is particularly important when complex highly skilled movements are used. These temperature-dependent changes are summarized in Table 4.7.
Improvement | Mechanism |
---|---|
Muscle work | Faster muscle contraction and relaxation speeds |
Economy of movement | Lowered viscous resistance within muscle |
Oxygen delivery and usage | Haemaglobin releases oxygen more easily as tissue temperature rises |
Nerve conduction | Increased temperature accelerates metabolic rate within nerve. Specific warm-up rehearses motor pattern |
Blood perfusion | Local vascular bed dilated |
Source McArdle, W.D., Katch, F.I. and Katch, V.L. (2001) Exercise Physiology, Energy, Nutrition and Human Performance, 5th edn. Lea and Febiger, Philadelphia. With permission.
The increased tissue temperature created by a warm-up will alter the force−velocity curve of a muscle. The effect is to shift the curve to the right by 12% for each 1°C increase in temperature (Enoka, 1994). The change in contraction velocity (maximal velocity of shortening) results in an increase in peak power output of the muscle (Fig. 4.2).
Large temperature changes have been shown to affect maximal isometric force. Cooling the hand muscles to 15°C reduced maximum isometric force by 30% (Ranatunga, Sharpe and Turnbull, 1987), while warming the quadriceps changed maximal isometric torque from 262 Nm at 30.4°C to 312 Nm at 38.5°C, an increase of 2.4%/1°C (Bergh and Ekblom, 1979b).
Mobilization hypothesis
In the initial period of intense exercise, high amounts of energy are required immediately. The anaerobic reserves are quickly used up, and the aerobic system has not yet become fully functional. The difference between the energy needed and that which can be supplied is known as the oxygen deficit, and represents stored energy and the build up of metabolic wastes (Fig. 4.3). When exercise stops, the body continues to provide energy aerobically to replenish the energy stores and metabolize waste products which have accumulated. This, in turn, creates the oxygen debt.
Gutin and Stewart (1971) argued that a function of warm-up was to mobilize the body’s cardiovascular system to reach a steady state. As warm-up was stopped, a brief rest period before competition allowed the oxygen debt to be repaid, without letting the cardiovascular system return to normal levels. When competition commenced, the oxygen deficit would be smaller, and some anaerobic energy would be available to the athlete at the end of exercise.
Gutin et al. (1976) asked subjects to pedal a cycle ergometer at an intensity sufficient to produce a heart rate of 140 b.p.m., a rate which they claimed equated with a 50–60% VO2 max. The subjects’ performance in a subsequent exercise task was significantly better than a control group who did not undertake a warm-up, a result possibly due to the mechanism described above.
A rest period is essential after the warm-up, to allow the oxygen debt to be repaid. But, following rest, the body must be kept warm to maintain the warm-up effects until the athlete competes. As an illustration, Andzel and Gutin (1976) used bench stepping both as a warm-up and exercise task. A 30- or 60-second rest after the warm-up resulted in improved performance, but when no rest period followed the warm-up, performance remained unchanged.
How long should a warm-up last, and what intensity of exercise should be used? A number of papers have addressed these questions. Andzel (1982) compared warm-up periods at an intensity sufficient to produce a heart rate of 120 and 140 b.p.m., followed by a 30-second rest period. Performance was significantly better with the 140 b.p.m. group. Richards (1968) argued that while some warm-ups would enhance performance, others could interfere with performance if fatigue set in. By varying the length of stool stepping, she concluded that a 1- or 2-minute warm-up was superior to a 4- or 6-minute period in her study. Bonner (1974) saw similar effects by altering warm-up periods with a static cycle task. Where performance was reduced in these examples, the workload was obviously too high. Sargeant and Dolan (1987) compared warm-up periods with changing intensity assessed by percentage of VO2 max, and concluded that a 39% VO2 max intensity was superior to a warm-up at 56% VO2 max.
Biomechanical effects
Safran et al. (1988) showed that a greater force and length of stretch was required to tear isometrically preconditioned muscles (Fig. 4.4). They claimed that the rise in temperature occurring during the warm-up period could alter the viscosity of the connective tissue within the muscle, and that isometric contractions caused a stretch at the musculotendinous junction. LaBan (1962) showed a 1.5% increase in the length of a stretched tendon following a temperature increase to 42.5°C. Warren, Lehmann and Koblanski (1971) demonstrated increases of 5.8% in length and 58% in force to failure for tendons heated to 45°C.
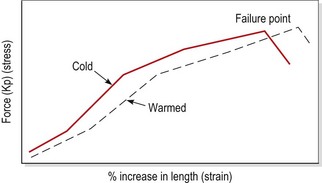
Figure 4.4 The effect of warm-up on tissue failure.
After Safran, M.R. et al. (1988) The role of warmup in muscular injury prevention. American Journal of Sports Medicine, 16 (2), 123–129. With permission.
Shellock and Prentice (1985) argued that muscle elasticity is dependent on blood saturation. They claimed that cold muscles with lower blood saturation levels were therefore more susceptible to injury. Fluids exhibit higher viscosity with lower temperatures, and so joint inertia will be greater when the synovial fluid of a joint is colder.
Changes have also been noted in structural stiffness of muscle following warm-up and exercise. Immediately following activity, muscle stiffness is increased, but can be significantly reduced by stretching. The increase in stiffness is thought to result from thixotropy (Enoka, 1994), the property exhibited by materials whereby they become more fluid when disturbed (shaken). Within muscle, stable bonds are formed between actin and myosin filaments. The bonds are increased following activity, but disengaged by stretching. This has important implications for both warm-up and cool-down. Warm-up will help minimize general muscle stiffness, while cool-down will reduce the actin and myosin bonding which remains following exercise (see also DOMS).
Proprioception has been shown to improve as a result of warm-up (Bartlett and Warren, 2002). Joint position appreciation is more sensitive in the knee after a warm-up, demonstrating that joints seem to accommodate to increased ligamentous laxity which results from a reduction in stiffness due to exercise. The method through which this occurs is thought to be an increase in the sensitivity of the proprioceptive mechanisms around the knee.
Psychological effects
Two psychological factors are important in the context of warm-up; these are rehearsal and arousal.
Rehearsal
When an athlete is performing a skilled task, a period of rest followed by resumption of the same task may result in impaired performance. This phenomenon is called warm-up decrement (WUD), and is well documented (Adams, 1961; Schmidt, 1982).
A number of explanations have been suggested to account for WUD. At a basic level it is seen as simply forgetting an aspect of the motor skill. Nacson and Schmidt (1971) suggested that WUD results from a loss of ‘activity set’. They claimed that a number of variables such as arousal level and attention had to be adjusted (tuned) to a specific task. With practice, the adjustments reach an optimal level, which is reduced with rest. They showed that WUD could be reduced if, during the rest period, a completely different movement was practised. This second movement could not contribute to the memory of the first task, but did require a similar activity set to the original skill.
Arousal
The second psychological effect of warm-up is that of arousal. The relationship between level of arousal and performance is demonstrated by the inverted-U hypothesis (Fig. 4.5). In a plot of arousal level against performance, initially increased arousal correlates initially with improved performance. But, as arousal continues to increase, an optimal level is reached. Above this point, further arousal is detrimental to performance.
Warm-up technique
During cold weather it will take longer for the body’s core temperature to increase, and so the warm-up should be longer or more vigorous. A warm-up should generally be of sufficient intensity and duration to raise the body’s core temperature by 1–2°C, recognized by the onset of mild sweating. The warm-up effects may persist for 45–80 minutes, the time variation being dependent on the rate of heat loss (DeVries, 1980).
Practically, the warm-up may be divided into three parts: pulse raising, mobility and rehearsal (Norris, 2002).
Warm-down
On cessation of exercise it is important to reverse the processes which occurred during the warm-up. The heart is no longer helped by the rhythmic contraction and relaxation of the leg muscles. Consequently, to stop intense exercise immediately will increase the demand on the cardiovascular system, causing the heart rate to rise. Metabolic waste products formed during exercise, such as lactic acid, will no longer be carried away from the working area with so much vigour. Instead they will remain in the area, causing pain. This is thought to be one possible cause of delayed onset muscle fatigue (Byrnes and Clarkson, 1985). Flushing the area with fresh blood by performing a gentle warm-down can reduce this effect.