101 Metabolic Bone Disease
Osteoporosis is characterized by low bone density and a deterioration of bone microarchitecture that reduces bone strength and increases the risk of fracture. The hallmark of osteoporosis is the loss of bone mineral and bone matrix that results in maintenance of a normal mineral-to-matrix ratio. Bone consists of an organic matrix (collagen and noncollagenous proteins) and an inorganic mineral component (calcium and phosphate in hydroxyapatite crystals; see Chapter 4). Normally, bone turnover is tightly coupled with osteoclast-mediated bone resorption followed by osteoblast-stimulated bone formation. This delicate balance in bone remodeling results in no net change in skeletal mass. Osteoblasts synthesize osteoid—bone matrix that subsequently undergoes mineralization and becomes mature bone matrix. The skeleton contains approximately 80% cortical bone, which is concentrated in the appendicular skeleton and femoral neck, and 20% more metabolically active trabecular bone, which is located in the spine, epiphyses, and pelvis. Osteoporosis is characterized by reduced bone strength usually accompanied by a reduction in bone mass. Osteomalacia encompasses disorders in which there is decreased mineralization of bone matrix. Paget’s disease is a skeletal disorder characterized by increased rates of bone turnover with the development of disorganized woven bone.
Osteoporosis
Epidemiology and Clinical Signs
Osteoporosis, the most common metabolic bone disease, affects 200 million individuals worldwide. Approximately 28 million Americans have osteoporosis or are at risk for it. Osteoporosis, or “porous bone,” is a “disease characterized by low bone mass and structural deterioration of bone tissue, leading to bone fragility and an increased susceptibility to fractures, especially of the hip, spine and wrist.”1 Although usually asymptomatic, osteoporosis can produce loss of height, pain, dowager’s hump, and increased risk of fracture. After 50 years of age, there is an exponential rise in fractures, such that 40% of women and 13% of men develop one or more osteoporotic fractures in their lifetimes. In the United States alone, there are more than 1.5 million osteoporotic fractures annually including 250,000 hip, 250,000 wrist, and 500,000 vertebral fractures. Hip fractures are associated with a 12% to 24% mortality rate in women and a 30% mortality rate in men within the first year of fracture, and 50% of patients are unable to ambulate independently and require long-term nursing home care.2 These numbers will continue to grow exponentially as the elderly population of industrialized nations increases.
Bone accretion occurs during adolescence, when there is a large increment in bone mass. Peak bone density is normally achieved after puberty and into the third decade of life. However, by age 22, most individuals have achieved nearly all of their peak bone mass. At menopause, an acceleration of bone loss usually occurs over approximately 5 to 8 years, with an annual 2% to 3% loss of trabecular bone and a 1% to 2% loss of cortical bone. Both men and women lose bone with age. Over a lifetime, women lose approximately 50% of trabecular and 30% of cortical bone; men generally lose two-thirds of these amounts.3 Osteoporosis was previously thought to be a silent disease that was part of the normal aging process. However, the advent of bone densitometry has made it possible to accurately and reproducibly identify patients at risk for osteoporosis so that prevention and treatment strategies can be instituted to reduce fractures. With a health care expenditure of $13.8 billion annually for osteoporosis-related fractures and a projected threefold rise in these costs over the next 40 years in the United States, the institution of effective prevention and treatment strategies to reduce fractures is of great importance.1,4
Pathophysiology of Menopausal and Age-Related Bone Loss
Bone is constantly undergoing remodeling, whereby areas of bone resorption produced by osteoclastic action are replaced by bone laid down by osteoblasts. Osteoporosis results from an imbalance between bone resorption and formation. The initiation of bone remodeling is still being debated; however, the osteocytes, or terminally differentiated osteoblasts, located within the bone matrix and connected to one another and the bone surface may release chemical mediators that attract osteoclasts to the bone surface (Figure 101-1). Osteoclasts originate from the colony-forming unit granulocyte-monocytes, are attracted to the bone surface, attach to bone matrix, and resorb bone tissue. Generally, bone resorption is rapid, and a resorption pit is formed within 10 to 14 days. After resorption is complete, osteoblasts, derived from the bone marrow stromal cells, attach to the resorbed bone surface and produce osteoid, which is then mineralized. Bone formation can take up to 3 or 4 months. Therefore a normal bone remodeling cycle in adults can last 4 to 6 months (see Figure 101-1A). A number of metabolic changes such as estrogen deficiency, immobilization, metabolic acidosis, hyperparathyroidism, and systemic and local inflammatory diseases can increase osteoclast number and activity, uncoupling bone turnover. This results in greater bone resorption than bone formation and a net loss of bone tissue. New data show that a number of local factors in bone affect the regulation of bone formation and resorption and the coupling of these processes. These include insulin-like growth factors (IGFs), interleukins (IL-1, IL-6, and IL-11), tumor necrosis factor (TNF), receptor activator of nuclear factor κB ligand (RANKL), and transforming growth factor (TGF).5 Animal studies have shown that IL-1, IL-6, and TNF knockout mice do not lose bone with estrogen deficiency.6 In addition, inflammatory arthritis animal models find that TNF, IL-1, and IL-6 are all strong stimulators of osteoclastic bone resorption. This link between the immune system and the maintenance of bone mass is intriguing, but additional work is required before we can understand its significance.
A number of mechanisms underlie primary osteoporosis including a low peak bone mass as a young adult and rapid bone loss during menopause. Factors contributing to age-related bone loss include impaired calcium absorption with age, a compensatory rise in parathyroid hormone (PTH) levels, and greater resorption than formation of bone. Estrogen deficiency is associated with the release of cytokines including RANKL, IL-1, IL-6, and TNF, which leads to the recruitment and stimulation of osteoclasts in the marrow and increased production of bone-resorptive cytokines, which may contribute to menopause-related bone loss.5 Estrogen therapy, however, inhibits IL-1 release, and in oophorectomized rats and mice, an inhibitor of IL-1 (the IL-1 receptor antagonist) suppresses bone loss.6 IL-6 levels also increase with age in human marrow cultures7 and in peripheral monocytes. IL-1 and TNF induce the production of IL-6 from osteoblasts and stromal cells. Further evidence supporting a role of IL-6 in bone turnover includes data showing that oophorectomized IL-6 knockout transgenic mice do not lose bone. Two other proteins have been identified that influence osteoclast activity: osteoprotegerin (OPG) and RANKL, which are produced by osteoblasts.8 Estrogen deficiency increases osteoblast production of RANKL, which stimulates maturation and activity of osteoclasts by attaching to RANKL on the surface of immature and mature osteoclasts. Simultaneously, estrogen deficiency decreases osteoblast production of OPG, the decoy receptor that reduces RANKL production and activity. Adenoviral delivery of OPG ameliorates bone resorption in a mouse ovariectomy model of osteoporosis.8 Both preclinical animal models and clinical trials of women with low bone mass have been completed and demonstrate that inhibition of RANKL with a monoclonal antibody (RANKL inhibitor) prevents estrogen-deficiency bone loss.9
In addition, a number of genetic, nutritional, and lifestyle risk factors predispose to the development of osteoporosis. Whites and Asians are at risk for low bone mass and osteoporosis, whereas African-Americans have a higher bone density and one-third to one-half the number of fractures.1,8,9 Some studies show that African-Americans have lower vitamin D and urinary calcium levels, higher PTH levels, and skeletal resistance to the effects of PTH on bone.10–12 Studies in twins and families show that up to 80% of the variance in bone mass is accounted for by genetic factors.13 A maternal history of hip fracture, for example, is associated with a twofold increased risk of a hip fracture.14 Data from Uitterlinden and colleagues15 show that the gene encoding collagen type IA1 is associated with low bone density with increasing age and an increased risk of fracture. In the ss allele group, bone density was 12% lower at the femoral neck and 20% lower at the lumbar spine than that in the SS group, indicating an increased gene-dose effect with increasing age. However, COLIA1 is associated with a lower baseline bone density and not an increased rate of bone loss. Further, genetically determined architectural features of bone such as a long hip axis length may contribute to increased fracture risk; conversely, a short hip axis length confers some protective effect.16 Recently, a family has been described whose members have high bone mass but are otherwise phenotypically normal. This family has a mutation (an amino acid change) in the low-density lipoprotein receptor-related protein 5 (LRP5). Using in situ hybridization to a rat tibia, expression of LRP5 was detected in areas of bone involved in remodeling. Additional studies have reported that this LRP5 mutation increases wnt signaling, which may alter bone mass through a primary defect of bone formation. Individuals with this mutation demonstrate normal levels of bone resorption, but specific markers of bone formation are strikingly elevated. The observation that LRP5 is expressed at high levels in osteoblasts is consistent with its having a role in this area. Further work is now required to determine whether other mutations in the chromosome containing the LRP5 segment are associated with a variation in bone density in the general population.17,18
Recently, genome-wide scans have been performed in a number of cohorts and have found a number of single nucleotide polymorphisms (SNPs) that are associated with osteoporosis (fracture or bone mineral density [BMD] associations). They include VDR, ESR1, ESR2, LRP5, LRP4, SOST, GRP177, OPG, RNAK, RNAKL, COL1A, SPP1, ITGAI, SP7, and SOX6, which can be reasonably assigned as confirmed or replicated, and another 30 or so genes as promising candidates. Of note, confirmed and promising genes are clustered in three biologic pathways: the estrogen endocrine pathway, the wnt/beta-catenin signaling pathway, and the RANKL/RANK/OPG pathway. New biologic pathways will certainly emerge when more osteoporosis genes are identified and validated.18a Other risk factors for osteoporosis, as enumerated in Table 101-1, include low body weight and reduced gonadal steroid levels.13 Lifestyle factors that may contribute to the development of osteoporosis include cigarette smoking, excessive alcohol intake, reduced physical activity, and inadequate calcium intake, according to some reports. Cigarette smokers have poorer health than nonsmokers, impaired calcium absorption, lower estrogen levels, earlier menopause, and more fractures, and they exercise less; smoking cessation reverses this risk of osteoporosis.
Table 101-1 Risk Factors for Osteoporosis
Primary |
Secondary |
Nonmodifiable |
Modifiable |
In a large, prospective study of 9516 women older than 65 years, the following lifestyle factors significantly increased the risk of hip fracture: no walking for exercise, intake of more than two cups of coffee daily, current use of long-acting benzodiazepines and anticonvulsant drugs, current weight less than weight at age 25 years, height greater than 5 feet 7 inches, age older than 80 years, fracture since age 50 years, inability to stand from a chair without using arms, poor depth perception, and self-evaluation of health as fair to poor.14 Low bone density in conjunction with a fall or trauma predisposes an individual to a fracture. Poor health and compromise of neuromuscular function increase the risk of osteoporosis and falls, which in turn increase the risk of hip fracture.14 Importantly, elderly white women with both a low bone mass and more than two risk factors have a nearly 20-fold increased risk for fracture.
Secondary causes of bone loss that can affect women and men of all ages and races are listed in Table 101-2. Glucocorticoid therapy is the most common secondary cause of bone loss. Osteoporotic fractures develop in an estimated 30% to 50% of glucocorticoid-treated patients.19 Glucocorticoid therapy causes bone loss through a number of different mechanisms such as producing a negative calcium balance through impaired intestinal calcium absorption, increasing urinary calcium excretion, decreasing bone formation, increasing bone resorption by stimulating osteoclast activity by macrophage colony-stimulating factor, and suppressing endogenous gonadal steroid production.19 Therapy with glucocorticoids leads to an early and, in some instances, dramatic loss of trabecular bone, with less effect on cortical bone. In hyperthyroidism (Graves’ disease or toxic nodule) or supraphysiologic therapy with thyroid hormone, the ensuing accelerated bone turnover may produce a reduction in bone mass when the thyroid-stimulating hormone level is suppressed, even when thyroid hormone levels are within the normal range.20 Athletic amenorrhea, anorexia nervosa, and other hypogonadal states including the use of gonadotropin-releasing hormone agonists21,22 may result in bone loss. In addition to estrogen deficiency, women with anorexia nervosa have low levels of IGF-I and reduced levels of adrenal androgen dehydroepiandrostenedione, which may contribute to the development of osteoporosis.23
Table 101-2 Medical Disorders and Medications Associated with Bone Loss and Osteoporosis
Modified from LeBoff MS: Calcium and metabolic bone disease. In Medical knowledge self-assessment program, Philadelphia, 1995, American College of Physicians.
Osteoporosis in Men
Osteoporosis in men was not recognized 20 years ago but is now a major public health problem owing to men’s longer life spans. The epidemiology of osteoporosis in men is just now being evaluated. Fracture risk occurs in adolescence and young adulthood and then increases after the age of 70. Long bone fractures occur more commonly in young men, while hip and spine fractures are more prevalent in men older than 70 years. The increase in fractures in older men is just as significant as it is in women, but it occurs about 10 years later in life, with an age-adjusted incidence of hip fractures in men of about one-third to one-half that of women.24 Elderly men who sustain hip fractures have a greater risk of dying or being permanently disabled compared with women.24 Risk factors for osteoporosis in men include older age, low BMD, history of a low-trauma fracture as an adult, and a family history of osteoporotic fractures.
There are a number of secondary causes of osteoporosis in men; for instance, hypogonadism causes an increase in bone turnover and rapid bone loss as gonadal function declines with age. At this time, severe hypogonadism from androgen deprivation therapy for prostate cancer is common in elderly men. The exact role of estrogen and androgens in male skeletal health is not yet known. Although estrogen is necessary for the young male skeleton, serum estrogen levels are highly correlated with bone remodeling, BMD, and rate of BMD loss in older men; the associations are stronger than with testosterone. However, serum testosterone levels are also highly correlated with indices of bone resorption and formation. The roles of estrogen and testosterone in the male skeleton need additional investigation.25 Some of the other common causes of osteoporosis in men that are not as frequent in women are alcoholism, gastrointestinal disorders including hepatic disorders, and malabsorption.24
Osteoporosis in Rheumatic Diseases and Other Conditions
Recently, studies have reported significant bone loss in patients with systemic inflammatory diseases such as rheumatoid arthritis, systemic lupus erythematosus (SLE), and ankylosing spondylitis. Patients with rheumatoid arthritis experience periarticular and generalized bone loss, with an increased incidence of fractures compared with the general population.26 T lymphocytes, tissue macrophages, and synovial-like fibroblasts release inflammatory cytokines (IL-1, TNF, IL-6) and inhibitory wnt signaling proteins such as dkk-1 and RANKL, which stimulate preosteoclasts in the bone marrow and synovium to actively resorb bone; in addition, osteoblast maturation is altered.27,28 In an animal model of inflammatory arthritis induced with collagen, animals pretreated with OPG did not have bone loss within the periarticular bone or the presence of erosions.29 Additional factors that may contribute to osteoporosis in patients with rheumatic diseases include decreased mobility, glucocorticoid therapy, and systemic inflammation.30 Some data, however, show that low-dose glucocorticoid therapy in women with rheumatoid arthritis does not have adverse skeletal effects, possibly because of a decrease in disease activity in association with the suppression of inflammatory cytokines and improved physical activity and function.31,32 Ankylosing spondylitis is also associated with fractures and reduced bone density in the spine and proximal femur, even early in the disease.33 Patients with SLE have a high rate of osteoporotic fractures in the presence of low to normal bone mass, suggesting that systemic inflammation alters bone turnover. Increased serum levels of TNF can reduce osteoblast maturation and increase osteoclast maturation and activity; in addition, other inflammatory factors such as oxidized low-density lipoproteins and inflammatory high-density lipoproteins can direct mesenchymal stem cells to differentiate into adipocytes instead of osteoblasts and impair bone mass.34 Infiltrative processes in the marrow such as multiple myeloma, mastocytosis, and Gaucher’s disease may produce osteoporosis. Patients with Gaucher’s disease show an accumulation of glucocerebrosides in macrophages in the spleen, liver, and bone marrow, which causes hepatosplenomegaly, anemia, thrombocytopenia, bone infarcts and infections, fractures, and aseptic necrosis.35
The immunosuppressant drug cyclophosphamide (Cytoxan) induces amenorrhea and hypogonadism, which may increase the risk of bone loss. Women who undergo premature menopause from cyclophosphamide therapy can have estrogen-deficiency bone loss in their 30s. Young women with SLE who try to preserve ovarian function while undergoing cyclophosphamide therapy by taking gonadotropin-releasing hormone agonists may also experience estrogen-deficiency bone loss. In rodent models, the immunosuppressive drug cyclosporine produces a time- and dose-dependent bone loss36; in contrast, azathioprine (Imuran) and rapamycin (sirolimus) do not appear to adversely affect skeletal homeostasis.37 Therapy with both cyclosporine and prednisone in transplant recipients is associated with early accelerated bone loss after the initiation of treatment and the development of osteoporosis and fractures with continued exposure.38
Vitamin D deficiency may also manifest as osteopenia and fractures, but this condition is both preventable and treatable.39 Vitamin D insufficiency is common in older patients and in those with SLE who do not get an adequate amount of sunlight or use potent sunscreens. Also, patients with malabsorption syndromes and liver disease can be vitamin D deficient. Unlike the situation in osteoporosis, very low vitamin D levels are often characterized by a mineralization defect and osteomalacia. Vitamin D deficiency is reported to be present in up to 50% of women with hip fractures.39
Assessment of Bone Density and Osteoporotic Risk
Techniques for evaluating bone mass include dual-energy x-ray absorptiometry (DEXA) and quantitative computed tomography (CT) scanning of the spine.1,2 Bone density evaluations using DEXA incorporate the attenuation of soft tissue and bone by radiographs to calculate the BMD. DEXA is both precise and safe, with a low radiation exposure. With reproducibility errors of approximately 0.6% to 1.5%, this technique can detect small changes over time.2,40,41 Further, newer DEXA techniques measure bone density rapidly, in 0.5 to 2.5 minutes. Quantitative CT scanning allows for the direct measurements of trabecular bone in the central region of the spine, but the procedure entails a comparatively high radiation exposure and time and precision errors are usually higher than those associated with DEXA.
Figure 101-1B and C show the BMD in a postmenopausal patient compared with that of young, healthy controls to determine whether there is reduction in BMD compared with peak bone mass (percentage of young healthy controls expressed as a t score) and with age-matched controls to assess whether BMD is diminished relative to an age-matched cohort (percentage of age-matched controls expressed as a z score). There is an inverse relationship between bone density and the gradient of risk for fracture.42 Prospective studies show that bone densitometry identifies patients with an increased gradient of risk for fracture. In 8134 women, a 1–standard deviation (SD) decrement in the bone density of the spine and the femoral neck compared with age-adjusted controls was associated with a 1.6- and 2.6-fold increased risk of hip fracture, respectively.43 Measurement of bone density in the hip is more predictive of hip fracture than is measurement at another site. Studies show that in women older than 65 years, hip bone density is predictive of spine and hip fracture and that conventional spine density does not add to the diagnostic utility of a single hip bone density test in assessing the risk of fracture.
Although bone densitometry provides a quantitative measure of bone mass, in vitro studies using ultrasonography indicate that this technique also provides information about the mechanical properties of bone including both density and elasticity. These qualities are strong predictors of bone strength. Ultrasound techniques include speed-of-sound and broadband ultrasound attenuation methods; the speed-of-sound technique reflects bone density and elasticity, and broadband ultrasound attenuation is an indicator of bone density, bone structure, and composition. Approved for clinical use by the U.S. Food and Drug Administration (FDA), both ultrasound techniques have been shown to discriminate between normal and osteoporotic patients at increased fracture risk.44 The t score parameters used by some ultrasound machines do not correspond to t score levels as measured by DEXA. Although ultrasonography is a radiation-free technique that may provide information about the risk of fracture and bone quality, the reproducibility of this technique and the measurement sites of mainly cortical bone or low-weight-bearing locations may make it unsuitable for monitoring small changes in bone over time. Therefore ultrasound measurements cannot reliably be used to monitor response to osteoporosis therapies. Further data are necessary to validate the clinical utility of ultrasonography.
On the basis of the guidelines of the Scientific Advisory Board of the National Osteoporosis Foundation, bone densitometry is useful in determining which patients might benefit from therapy to protect the skeleton including patients who have a deficiency of gonadal hormones (postmenopausal women younger than 65 years with one or more risk factors or older than 65 years regardless of risk factors), postmenopausal fracture, evidence of osteopenia or a vertebral abnormality on radiographs, hyperparathyroidism, or exposure to supraphysiologic doses of glucocorticoids (Table 101-3). Bone densitometry is also used to decide when to commence therapy for osteoporosis and to assess the clinical response to therapeutic interventions.40 Screening normal premenopausal women is not cost-effective.
Table 101-3 Indications for Bone Densitometry
The World Health Organization (WHO) has published criteria for osteoporosis on the basis of bone density1,45:
1. Normal bone density if the t score is greater than −1.
2. Osteopenia (low bone mass) is defined as a bone density measurement between 1 and 2.5 SD below the young-adult mean (t score between −1 and −2.5).
3. Osteoporosis is defined as a bone density measurement less than 2.5 SD below that of young, healthy controls (t score < 2.5).
The National Osteoporosis Foundation recommends treatment for all individuals who have a lumbar spine, hip, or femoral neck t score of −2.5 or lower. However, for individuals who have a bone density between −1 and −2.5 the National Osteoporosis Foundation recommends performing a Fracture Risk Assessment or FRAX using a computer program that incorporates clinical risk factors for osteoporosis with and without femoral neck BMD. FRAX also provides a 10-year risk of a hip fracture or a major osteoporotic fracture (hip, proximal humerus, and wrist).The clinical risk factors included in the FRAX program include age, weight, height, history of a fracture as an adult, parental history of a hip fracture, current glucocorticoid use, secondary cause of osteoporosis, alcohol intake of more than two drinks a day, and current smoker.45,46 These risk factors are added to femoral neck t score for the 10-year fracture risk. In the United States, a 10-year risk of hip fracture of 3% or more or a major osteoporotic fracture of 20% or more is the threshold to recommend treatment. However, the fracture risk threshold for treatment is individualized by treatment, so it is important to enter the country in which you are practicing medicine. FRAX has advantages that include easily determined risk factors, global validation, application in specific regions or nations, and scores that pertain to both men and women. However, there are a number of limitations including that it can lead to underestimates or overestimates of fracture risk in some patients, because it does not include all known risk factors and some risk factors are superficial (e.g., glucocorticoid use is the question, but dose is not evaluated). Such known or suspected risk factors that contribute to fracture risk but are not included in the FRAX algorithm include immobilization, epilepsy, chronic obstructive pulmonary disease, diabetes, and depression. Lastly, the FRAX score is calibrated only for untreated patients and results can be misleading for patients already taking pharmacologic therapy.45,46
Markers of Bone Turnover
The development of sensitive biochemical markers of bone turnover makes it possible to analyze changes in bone formation and resorption at a given point in time and obtain additional information about a patient’s risk of bone loss and fracture. Only three bone formation markers are currently available. Osteocalcin, a noncollagenous matrix protein in bone, is produced exclusively by osteoblasts; it correlates with histomorphometric bone measurements. In most conditions, bone resorption and formation are tightly coupled and osteocalcin levels reflect bone turnover. The other markers of bone formation are bone-specific alkaline phosphatase (BSAP), an enzyme that is activated as osteoblasts mature, and amino-terminal propeptide of type I procollagen, a protein whose synthesis is high in maturing osteoblasts.47
Sensitive indicators of bone resorption derived from the degradation of mature collagen include the urine and serum markers of type I collagen cross-links including amino-terminal telopeptide of type I collagen (N telopeptides, or NTX) or carboxy-terminal telopeptide of type I collagen (C telopeptides, or CTX). Urinary pyridinoline cross-link, NTX, and CTX levels correlate with histomorphometric determinations of bone resorption; these biomarkers increase with menopause and are high in patients with a variety of disorders characterized by accelerated bone turnover including Paget’s disease, osteoporosis, and rheumatoid arthritis.48,49 Urinary excretion of N telopeptides is inversely related to total hip and spinal bone density and, according to some studies, may be a more specific index of bone resorption than urinary pyridinoline levels.50 Small prospective studies have found high turnover markers, and low BMD has been associated with incident fracture risk but these studies have not been replicated in larger studies.51,52 In clinical studies, antiresorptive agents such as estrogen, bisphosphonates, and inhibitor of RANKL induce a significant decrease (30% to 80%) first in markers of resorption and then in bone formation markers, often within 3 to 6 months. Resorption markers decrease before formation markers and correlate with either maintenance of or increase in BMD. A significant change in bone markers can be observed within months of antiresorptive therapy, before there are changes in BMD.52 Both bone formation and resorption marker changes over 6 to 12 months have been found to predict future fracture risk. In a study of alendronate to reduce osteoporotic fractures, patients who had more than a 30% reduction in bone alkaline phosphatase had the greatest reduction in risk for new vertebral and nonvertebral fractures. Interestingly, studies have found that reductions in markers of bone turnover, either resorption or formation markers, are associated with a reduction in fracture risk. However, long-term, prospective studies of large numbers of women are necessary to determine whether selective biochemical markers of bone turnover can predict changes in BMD or fracture risk and whether these tests should be used in standard clinical practice.
Most bone turnover marker data are derived from large studies of antiresorptive agents. However, a bone-building anabolic agent, PTH, has been approved for the treatment of osteoporosis. PTH’s action is to stimulate osteoblast activity; therefore osteocalcin and other markers of bone formation increase rapidly, within a few weeks of the initiation of treatment. However, activation of the osteoblast over time results in RANKL production, which stimulates osteoclast activity. With continued PTH treatment, markers of osteoclast activity also increase, reaching levels equal to those of formation markers. Because the overall result is an increase in bone mass, the bone turnover markers during PTH therapy reflect significant bone remodeling on both trabecular and cortical bone surfaces. A few small studies have found that increases in both bone formation and resorption markers predict an increase in bone mass with PTH treatment.53–55
Evaluation for Secondary Bone Loss
The workup for osteoporosis is directed toward excluding secondary causes of bone loss and includes a determination of serum calcium, phosphorus, supersensitive thyroid-stimulating hormone, 25-hydroxyvitamin D (25-OHD), and intact PTH, as well as urine calcium and creatinine levels. Also, a complete blood cell count, alkaline phosphatase and liver function tests, erythrocyte sedimentation rate (in some cases), and serum and urine protein electrophoresis for patients older than 50 years may be necessary (Table 101-4). In men, additional testing for secondary causes of osteoporosis includes serum testosterone and luteinizing hormone.
Table 101-4 Evaluation of Osteoporosis
For All Patients |
For Selected Patients* |
CBC, complete blood cell count; ESR, erythrocyte sedimentation rate; PTH, parathyroid hormone; SMA, sequential multiple analysis; TSH, thyroid-stimulating hormone.
* Children, premenopausal women, men younger than 60 yr, black, patients with rapidly progressive disease.
Modified from Primer on the metabolic bone diseases and disorders of mineral metabolism, ed 6, Washington, DC, 2006, American Society of Bone and Mineral Research.
Further tests to rule out neoplastic or endocrinologic disorders and a bone biopsy (a decalcified bone specimen is obtained after a double tetracycline label with two different fluorescent labels) should be considered in certain patients with progressive bone loss and in those in whom osteoporosis is unlikely. Identification and appropriate therapy for underlying secondary causes of osteoporosis are important. For example, treatment of vitamin D deficiency is best accomplished with vitamin D supplements. Parathyroidectomy in patients with hyperparathyroidism characterized by hypercalcemia, hypercalciuria, nephrolithiasis, age younger than 50 years, or low cortical BMD (z score ≤2) was associated with a large (4% to 12.8%) increase in bone density over 4 years.56 Bone density was, however, stable for up to 6 years in patients with mild hyperparathyroidism.57 In addition, treatment of hyperthyroidism, hypercortisolism, and a variety of other disorders that may cause osteoporosis can produce increments in bone mass. Reduction in the systemic inflammation associated with rheumatic diseases such as TNF blocking agents for rheumatoid arthritis or ankylosing spondylitis or glucocorticoid-sparing agents for SLE (e.g., azathioprine [Imuran], mycophenolate mofetil [CellCept]) can also produce increments in bone mass.
Treatment
Calcium
The goals of therapy for osteoporosis are to reduce bone resorption and enhance bone formation, if possible. Bone loss occurs when the calcium intake and absorption are insufficient to balance the daily calcium losses. Prospective data show that calcium stabilizes bone mass.58
Table 101-5 shows the current recommendations for optimal calcium intake for women and men from the 1997 report of the Institute of Medicine to the National Academy of Sciences.59 In the absence of kidney stones or an underlying disorder of calcium metabolism, these calcium intakes are safe. To prevent negative calcium balance, premenopausal women require 1000 mg and postmenopausal women 1200 mg of total elemental calcium daily.60 Children have increasing calcium requirements during adolescence, and data show increased bone accretion with increased calcium intake in prepubescent and pubertal children. Calcium carbonate contains 40% elemental calcium and should be taken with meals because of poor absorption in achlorhydric patients in the absence of food. Calcium citrate, which contains 24% elemental calcium, has better bioavailability and is more readily absorbed.61 It is also absorbed well on an empty stomach in patients with achlorhydria. Recent studies underscore the fact that only recommended daily allowances of calcium and vitamin D are useful for bone health in postmenopausal women and elderly men. Additional supplementation over the recommended daily allowance has resulted in reports of increased cardiovascular disease.61a–61c
Table 101-5 Calcium Requirements Recommended by the National Academy of Sciences (USA)
Age Group | Optimal Daily Calcium Intake (mg) |
---|---|
Infants | |
Birth-6 mo | 400 |
6 mo-1 yr | 600 |
Children 1-8 yr | 500-800 |
Adolescents 9-18 yr | |
9-10 yr | 800-1200 |
11-18 yr | 1200-1500 |
Pregnant and nursing females | 1300 |
Men and women | |
19-50 yr | 1000 |
>50 yr (± hormone replacement therapy) | 1200-1500 |
Modified from Atkinson SA, Abrams SA, Dawson-Hughes B, et al: Calcium. In Young V, editor: Dietary reference intake for calcium, phosphorus, magnesium, vitamin D and fluoride, Washington, DC, 1997, National Academy Press, pp 91–143.
Estrogen
Hormone replacement therapy (HRT) was once the mainstay of treatment in osteoporosis because estrogen inhibits bone resorption, produces a small rise in bone density, and reduces the risk of fracture by approximately 50% in retrospective observational studies. Cardiovascular disease is the leading cause of death in postmenopausal women. Previous data from longitudinal observational studies suggested that estrogen replacement had a beneficial effect on reducing primary and secondary cardiac events in postmenopausal women. However, in 1998, data from the 4-year Heart and Estrogen/Progestin Replacement Study were published.62 In this study, 2763 postmenopausal women with a previous history of heart disease were randomized to receive estrogen (0.625 mg) plus progestin (2.5 mg) or placebo alone. Results showed no reduction in the overall rate of coronary heart disease or cardiac events in the treatment group; in fact, an early increase in risk for cardiac events was noted, possibly related to increased coagulability.63
In addition, a large, multicenter, longitudinal study by the Women’s Health Initiative (WHI)—in which 162,000 women aged 50 to 79 years were randomized into a placebo group, an HRT group (if the uterus was intact), or an estrogen-only group (if the uterus was absent)—was terminated early due to an increased risk of breast and cardiovascular events. The research goals for the WHI study were to determine the effects of HRT, diet modification, and calcium and vitamin D supplements on heart disease, osteoporosis, and colorectal cancer risk. After a mean follow-up of 5.3 years in an 8.5-year study, the HRT group had an increased risk of seven more cardiac events per 10,000 women taking the drug for a year, eight more invasive breast cancers, eight more strokes, and eight more pulmonary emboli, but six fewer colorectal cancers and five fewer hip fractures.64
Selective Estrogen Receptor Modulators
The ideal estrogen replacement therapy would confer the beneficial effects of estrogen on bone and cardiovascular disease without increasing the risk of breast or uterine cancer. Selective estrogen receptor modulators (SERMs) are a nonsteroidal class of drugs that bind to the estrogen receptor and differ from one another in their actions on estrogen-responsive tissues, acting selectively as agonists or antagonists. Tamoxifen, the first available SERM, is an estrogen antagonist that binds to the estrogen receptor and also has estrogen-agonist effects on bone, lipids, clotting factors, and endometrium. Tamoxifen therapy in women with breast cancer produced a small increase in bone density of the spine over 2 years, with no effect on radial bone density, in association with reductions in both low-density lipoprotein and total cholesterol.65 The Breast Cancer Prevention Trial studied 13,388 women at increased risk for breast cancer, comparing treatment with tamoxifen (20 mg daily) to placebo for 5 years.66 Tamoxifen reduced the risk of invasive and noninvasive breast cancer by 50%, and a decreased risk of fracture was observed as well: 45% reduction at the hip and 29% at the spine. An increased incidence of low-grade endometrial cancer was noted, but there was no change in the risk of ischemic heart disease.66
Raloxifene,67 now FDA approved for the prevention and treatment of osteoporosis, is a SERM that acts as an estrogen agonist on bone, with antagonist effects on the breast and uterus.68 Raloxifene (60 mg/day over a 2-year study period) increased BMD in the lumbar spine by 2.4%, in the total hip by 2.4%, and in the total body by 2%, with a reduction in fracture risk at 2 years similar to that seen with estrogen or alendronate (5 mg) treatment. Over the 2-year study period, raloxifene produced a significant reduction in vertebral fractures: Fractures were present in 1.6% of raloxifene-treated women, compared with 2.9% of those in the placebo group; fractures recurred in 7.6% of treated women with a previous fracture, compared with 14.3% of those in the placebo group.69 Endometrial thickness is not increased by raloxifene, but menopausal symptoms may be made worse. Raloxifene has been shown to decrease low-density lipoprotein cholesterol by 12%, with a nonsignificant increase in high-density lipoprotein cholesterol; cardiovascular protection has not yet been determined.70 However, raloxifene, unlike estrogen, does not affect C-reactive protein, which is associated with a risk of cardiovascular disease.71,72 Raloxifene also decreased the incidence of breast cancer by 76% in patients enrolled in a clinical study of osteoporosis, with breast cancer incidence studied as a secondary endpoint.67 A study that evaluated the effects of raloxifene on cardiovascular disease found no effect.73 One study compared tamoxifen and raloxifene, and another study evaluated raloxifene versus placebo, in the prevention of breast cancer. The first study reported that both tamoxifen and raloxifene reduced the risk of developing breast cancer, and the second found that raloxifene reduced the risk of estrogen receptor–positive breast cancer compared with placebo in postmenopausal women.74,75 At this time, there is little information on the use of raloxifene in men, so it is not recommended for male patients.
Testosterone
Men with osteoporosis, hypogonadism, and symptoms of low libido may benefit from testosterone replacement therapy. This can be administered as testosterone cypionate or enanthate (50 to 400 mg intramuscularly every 2 to 4 weeks) or as a transdermal testosterone replacement patch that is applied to the scrotal area (Testoderm, 4 to 6 mg/day) or elsewhere (Androderm, 2.5 or 5 mg/day).76 Most studies find that bone mass increases with testosterone replacement when levels of testosterone were low at the initiation of therapy.
Calcitonin
Calcitonin, a 32–amino acid peptide synthesized by the C cells of the thyroid gland, is a potent inhibitor of osteoclast-mediated bone resorption. Although human and salmon calcitonin are commercially available, salmon calcitonin is most commonly used because of its greater potency. On the basis of data showing an increase in total body calcium, parenteral calcitonin was approved by the FDA for the treatment of osteoporosis in 1984, and calcitonin in a nasal spray was approved for the treatment of postmenopausal osteoporosis in 1995. Parenteral calcitonin (100 IU subcutaneously or intramuscularly three times a week or daily) can maintain bone density or produce a small increase in bone mass in the spine and, in some instances, the forearm, particularly in patients with a high bone turnover.77 Nasal spray calcitonin is absorbed through the nasal mucosa and is approximately 40% as potent as the parenterally administered drug (e.g., 50 to 100 IU of injectable calcitonin is comparable with 200 IU of nasal spray calcitonin).78 In osteoporotic women more than 5 years past menopause, nasal calcitonin (200 IU/day) increases spinal bone density 2% to 3% compared with placebo, with no effect on proximal femur bone mass; higher doses are necessary in the early menopausal period.78,79 Nasal spray calcitonin therapy in patients with osteoporosis is associated with a 36% reduction in vertebral fractures over 5 years.79
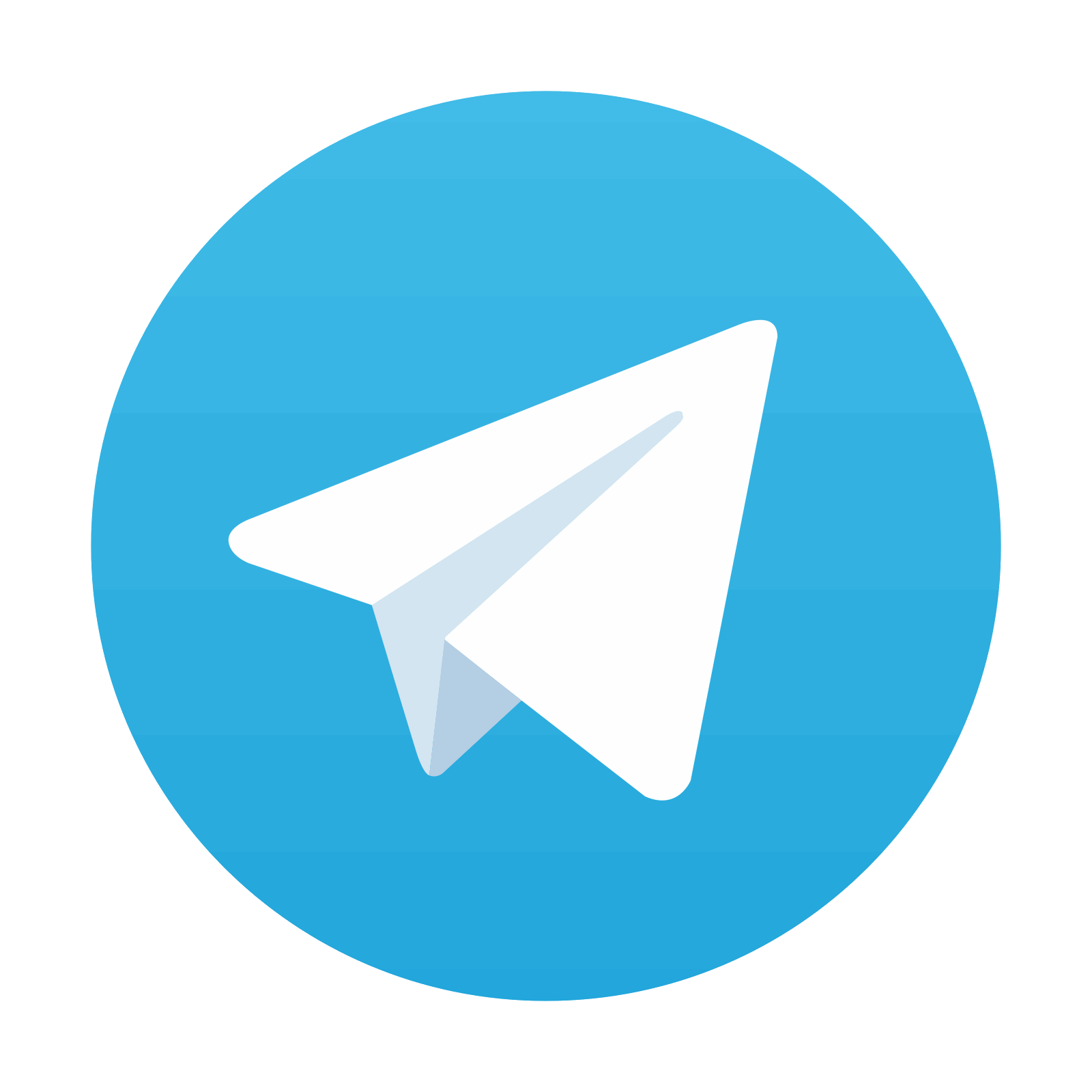
Stay updated, free articles. Join our Telegram channel

Full access? Get Clinical Tree
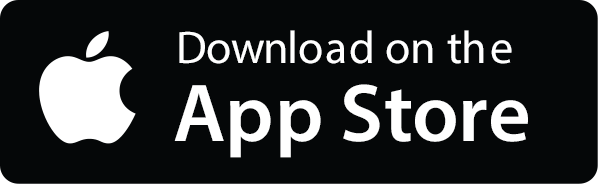
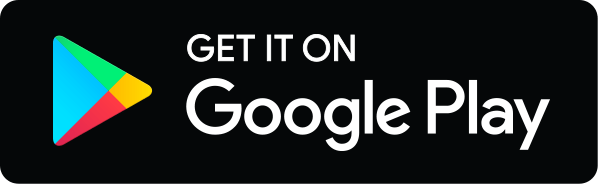