Fig. 22.1
Overview of the assessed radiologic parameters. (a) The acromiohumeral interval – distance from superior humerus to inferior acromion. (b) The acromion index – ratio GA/GH. GA the distance from the glenoid plane to the acromion, and GH the distance from the glenoid plane to the lateral aspect of the humeral head. (c) The critical shoulder angle – angle between a line connecting the inferior and superior borders of the glenoid fossa and a second line connecting the inferior border of the glenoid to the most inferolateral point of the acromion. (d) The lateral acromion angle – angle between a line drawn parallel to the sclerotic line of the acromion undersurface and a second line connecting the superior to the inferior border of the glenoid fossa
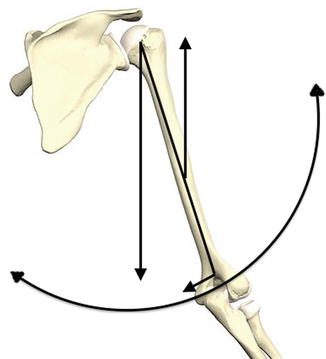
Fig. 22.2
Forces acting on glenohumeral joint. Rotator cuff muscles are predominantly stabilizing glenohumeral joint, but they also significantly contribute to the joint movement as well. They mainly contribute to abduction, external rotation, and internal rotation, and also play important role in joint compression
22.2.2 Scapula
In the resting position, the scapula extends from the 2nd rib to between the 7th or 9th rib at the inferior angle, and is anteriorly rotated 30° in the axial plane to accommodate the thoracic rib cage [5–7]. In the coronal plane, the scapula is rotated upward 3–10°, and when viewed in the sagittal plane, it is anteflexed 10–20° [8].
22.2.3 Glenoid
The orientation of the glenoid may be angled in both the axial and coronal planes. In the axial plane, anterior or posterior angulation is referred to as version. Glenoid version can range from retroversion to anteversion, with one study finding the mean version to be 1.23° of retroversion, with a range of 9.5° of anteversion to 10.5° retroversion [9]. Other sources have found the mean glenoid version to be closer to 7° of retroversion [5]. In the coronal plane, superior or inferior angulation is referred to as inclination. Churchill et al. found the mean glenoid inclination to be 4.2° of superior inclination, with a range of 7° of inferior inclination to 15.8° of superior inclination [9].
22.2.4 Humeral Head
The humeral head and shaft lie roughly in the plane of the scapula, with the articular surface of the humeral head comprising 1/3 of a sphere. The humeral head is oriented superiorly, forming a neck-shaft angle of 45°, and it rests in approximately 30° of retroversion, which is complimented by the anteversion of the scapula [5, 6].
22.3 Tendon Anatomy
22.3.1 Gross Anatomy
The subscapularis muscle alone inserts on the lesser tuberosity of the humerus, and is responsible for internal rotation of the arm (Figs. 22.3 and 22.4). The supraspinatus, infraspinatus, and teres minor all insert on the greater tuberosity (Figs. 22.5, 22.6, 22.7, and 22.8). The supraspinatus tendon passes through the subacromial space beneath the subacromial bursa to its insertion site on the superior facet of the greater tuberosity. This insertion site facilitates abduction of the upper extremity, but also is a component to the frequent impingement of this tendon. Beyond 70° of abduction, the greater tuberosity has a tendency to come into contact with the acromion, resulting in the impingement of both the tendon and the subacromial bursa [10, 11]. The infraspinatus and teres minor tendons insert on the posteroinferior facet of the greater tuberosity and working conjunction to facilitate external rotation.
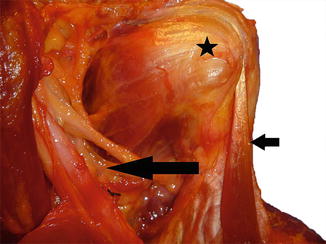
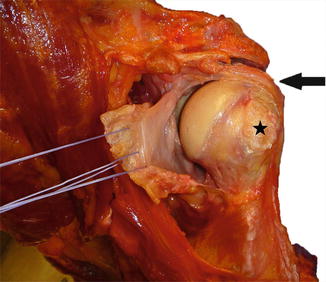
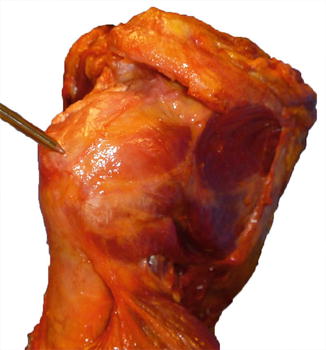
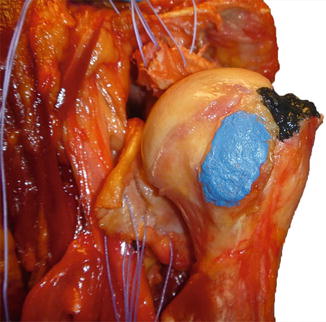
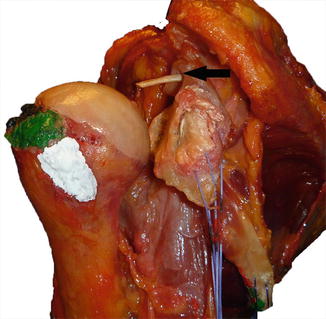
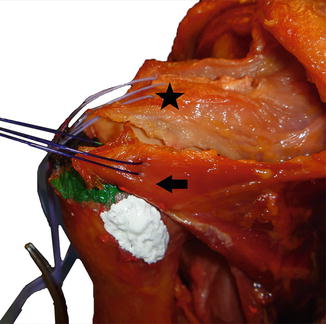
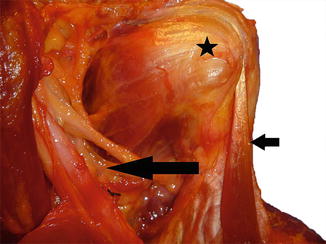
Fig. 22.3
Anterior rotator cuff musculature. Photograph of the anterior aspect of a cadaveric shoulder specimen demonstrating the subscapularis (star), the intact biceps tendon (short arrow), and the nearby presence of the neurovascular plexus (long arrow)
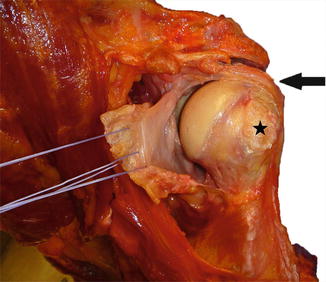
Fig. 22.4
Photograph of the anterior aspect of a cadaveric shoulder specimen after the subscapularis and capsule have been reflected from the lesser tuberosity (star). The supraspinatus remains intact on the greater tuberosity (arrow)
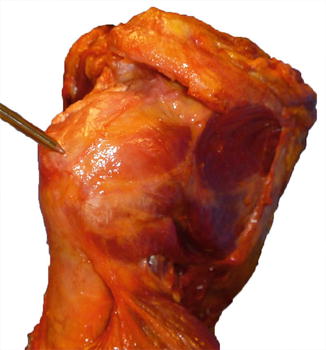
Fig. 22.5
Photograph of the posterior-superior aspect of a cadaveric shoulder specimen with the posterior cuff intact and the pointer resting on the lateral aspect of the greater tuberosity
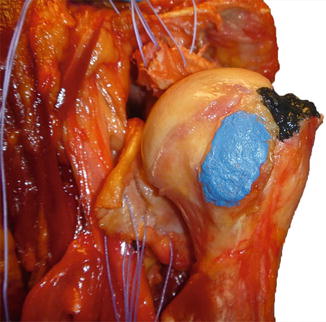
Fig. 22.6
Photograph of the anterior aspect of a cadaveric shoulder specimen after the subscapularis and supraspinatus have been reflected from the lesser tuberosity (painted blue) and the greater tuberosity (painted black)
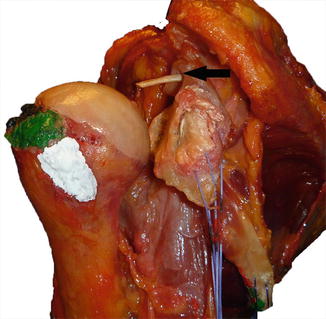
Fig. 22.7
Photograph of the posterior aspect of a cadaveric shoulder specimen with sutures in the reflected posterior rotator cuff. The attachment of the supraspinatus has been painted black (barely visible), the infraspinatus has been painted green, and the teres minor white. The biceps has been tenotomized, and its proximal segment is visible at the superior glenoid tubercle
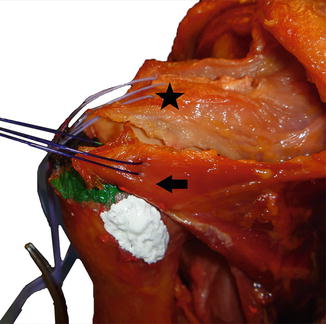
Fig. 22.8
Photograph of the posterior aspect of a cadaveric shoulder specimen with sutures in the posterior rotator cuff, which have been pulled back in-line toward their attachments. The attachment of the supraspinatus (star) has been painted black and the infraspinatus (arrow) has been painted green. The attachment of the teres minor has been painted white and remains reflected
22.3.2 Tendon Composition
22.3.3 Vascularity
The muscles of the rotator cuff derive their blood supply from the branches of the axillary artery (Fig. 22.9). The axillary artery is generally divided in to three segments, based on the borders of the pectoralis minor. Proximally, the thoracoacromial artery emerges from the axillary artery at the level of the upper border of the pectoralis minor. The artery pierces the clavipectoral fascia, and then divides into four branches that supply the muscles of the shoulder and proximal humerus. Of these four branches, the deltoid (or humeral) branch and acromial branch are the primary blood suppliers to the scapulohumeral muscles.
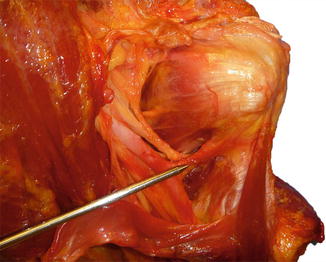
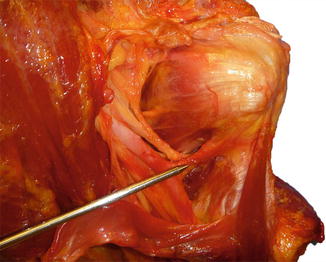
Fig. 22.9
Photograph of the anterior aspect of a cadaveric shoulder specimen with the pointer on the branch of the axillary artery providing the blood supply to the anterior aspect of the rotator cuff
At the lateral border of the pectoralis minor, the subscapular artery emerges to pass between the radial and median nerves and descends caudally to supply the subscapularis muscle. Eventually, the subscapular artery will give rise to the circumflex scapular artery whose branches form an anastomosis with the suprascapular and dorsal scapular arteries. Distally, the anterior and posterior circumflex arteries emerge and encircle the humerus, and provide blood supply to the glenohumeral joint capsule and rotator cuff tendons via its terminal branches [15, 16].
22.3.4 Nerve Supply
The muscles of the rotator cuff are innervated by the brachial plexus, which is formed from the branches of spinal roots C5–T1. In terms of surgical anatomy, the most important nerves are the suprascapular nerve and the axillary nerve. The suprascapular nerve arises from the superior trunk of the brachial plexus and passes through the suprascapular notch to enter into the supraspinatous fossa where it gives off two motor branches to innervate the supraspinatus muscle. It is as it passes under the superior transverse ligament that the nerve becomes most susceptible to injury via compression and shearing forces [5]. The nerve then continues to travel around the lateral border of the scapular spine and into the infraspinatous fossa via the spinoglenoid notch. The subscapular nerve arises from the posterior cord of the brachial plexus, and divides into an upper and lower nerve. The upper nerves insert directly into the subscapularis muscle, while the lower nerve continues to innervate the inferior portion of the subscapularis. The remaining innervation of the teres minor comes from the axillary nerve (Figs. 22.10 and 22.11) [17].
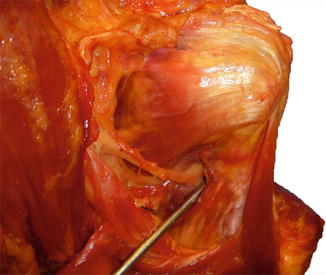
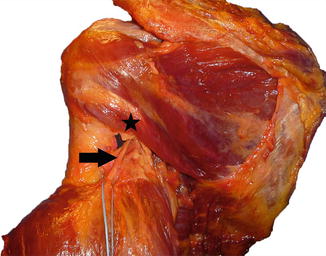
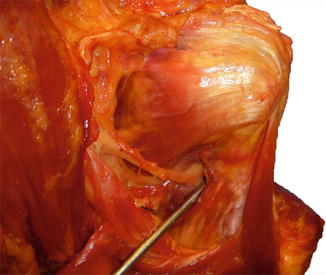
Fig. 22.10
Photograph of the anterior aspect of a cadaveric shoulder specimen with the pointer on the axillary nerve as it travels from anterior to posterior to exit posterior to the humerus (see next figure)
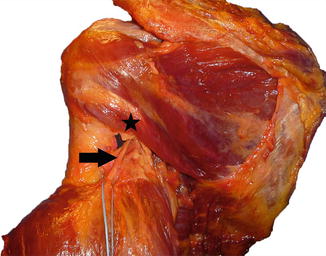
Fig. 22.11
Photograph of the posterior aspect of a cadaveric shoulder specimen with the axillary nerve (arrow), which is tagged with a suture as it exits the quadrilateral space after supplying the teres minor (star) with its innervation
22.4 Shoulder Function
22.4.1 Shoulder Motion
The motion of the shoulder is complex and takes into account motion at the acromioclavicular and sternoclavicular joints, the glenohumeral joint, and the scapulothoracic articulation. There have been numerous methods developed to describe the motion that occurs at each joint. For research purposes, the motion at the glenohumeral joint is often referred to in regard to rotation around three axes to give forward elevation, elevation in the plane of the scapula (or abduction), and humeral rotation. The term “scapulothoracic rhythm” was first used by Codman to describe the complex, coordinated movements of both the scapulothoracic articulation and the glenohumeral joint during arm motion. Further proving that this motion is coupled, pathology of the glenohumeral joint, such as the pain of a full-thickness RC tear, affects the kinematics of the scapulothoracic articulation [18].
During humeral elevation, a greater degree of motion occurs at the glenohumeral joint during the early arc of motion (first 30°). Scapular and clavicular motion are minimal with the arm in less than 90° of elevation. Beyond 90° of humeral elevation, there is upward rotation, posterior tilting, and external rotation of the scapula to allow for the full arc of motion in the upper extremity [19–21]. During this, the clavicle retracts, elevates, and rotates backward to accommodate this motion. The humerus must externally rotate to avoid impingement of the greater tuberosity under the acromion [22].
There is a lack of linearity to the ratio of glenohumeral to scapulothoracic motion with a ratio of 4:1 during the initial 25° of motion, which almost equates at 5:4 after that to average approximately 2:1 for the entire arc of elevation [23–26]. This coupled motion has been further elucidated with dual fluoroscopic imaging systems in an in vitro model [27].
22.4.2 Center of Rotation
When simplified to a single plane, the center of rotation lies within 6 mm of the geometric center of the humeral head [28]. This tight focus regarding the center of rotation is largely due to the small amounts of translation that occur during initial elevation. The center of rotation is also affected by the integrity of the rotator cuff as well as the long head of the biceps tendon [29, 30].
22.4.3 Biomechanics
Forces at the glenohumeral joint depend on multiple factors including the overall condition of the muscle, the size of the muscle as measured by the cross-sectional area, and the position of the joint. In general, a muscle is strongest at the midpoint of its excursion compared to when it is fully contracted or extended.
If the muscle is atrophic due to muscular or neural pathophysiology, it will not function as otherwise expected based on the biomechanics alone. Cross-sectional area of the muscle has been measured to determine a muscle’s volume and subsequent forces, which it is able to generate [31]. The orientation of the muscles surrounding the shoulder to the glenoid creates a joint reaction force with a large component perpendicular to the glenoid, further aiding humeral head compression [32]. The location of the muscle and tendon unit with regard to the joint also becomes important when setting up the testing apparatus during biomechanical testing. Tears of the RC affect the overall amount of force that can be produced in the abducted arm. When a tear was created that involved 1/3 or 2/3 of the supraspinatus, the force decreased only 5 %, and a complete, retracted tear of the supraspinatus caused only a loss of torque of 58 % [33].
The position of the arm also effects the direction of pull of the muscle, with the most obvious example being the supraspinatus, which can perform abduction or external rotation based on the arm’s position (Fig. 22.2). The position of the arm also affects the morphology of the rotator cuff, as was shown in an MRI study evaluating supraspinatus tendon during different positions of rotation and abduction of the humerus. Abduction over 30° was found to shorten the tendon, while external and internal rotations caused elongation of the anterior and posterior portions of the tendon, respectively [34]. The size of the individual rotator cuff muscle can be compensated for by positioning that creates a more effective moment arm. The subscapularis and infraspinatus muscles generate forces of two to three times more than the supraspinatus; however, a more effective moment arm causes the supraspinatus to be a much more effective abductor [35].
22.4.4 Function
The rotator cuff complex functions overall in three broad categories: rotation of the humerus about the scapula, compression of the humeral head into the glenoid, and providing muscle balance to the glenohumeral joint. The subscapularis functions to internally rotate the humerus, while the infraspinatus and teres minor are external rotators. The supraspinatus functions to abduct as well as provide weak external rotation with the arm in adduction [36].
Compression of the humeral head into the glenoid has been researched by determining reaction force testing. This is accomplished by using a dynamic shoulder testing apparatus that has determined that joint forces increase throughout abduction and peak at approximately 90° of motion. Firing of the supraspinatus increases the joint forces, and simulated paralysis of the supraspinatus results in a significant decrease in compression [37].
There remains controversy on how much muscle balance and stability to the glenohumeral joint is provided by the rotator cuff complex. Different in vivo studies have been performed in an effort to gain more realistic information on the stabilizing effects of the rotator cuff. Loads to the rotator cuff were given, and the strain of the inferior glenohumeral ligament was measured, showing that the infraspinatus and teres minor were most responsible for aiding to the stability of the GH joint [38]. It was also shown that the subscapularis stabilized anteriorly with the arm in abduction; however, with external rotation, the humerus became less important as the posterior musculature became of primary importance. The rotator cuff musculature has been shown to have a greatest effect on glenohumeral stability in the midrange of motion when the capsule-labral complex is lax [39].
The moment arm and orientations of the rotator cuff muscles change with the abduction angle of the arm. Different authors have measured this through radiographic studies [40, 41]. Not surprisingly, the anterior and middle deltoids as well as the supraspinatus have the largest moment arm.
Much of the work done to corroborate the biomechanical studies of the rotator cuff has utilized electromyography and selective nerve blocks to include or exclude certain muscles and record their activity. This type of research has led to a greater understanding of the proportion of individual rotator cuff muscle involvement with certain motions. The percentage of involvement of the supraspinatus with external rotation was quantified with this pattern of research, which has led to an increased recognition of physical exam tests to detect RC tears [42].
22.5 Pathology
22.5.1 Vascularity
It has been proposed that the rotator cuff tendon tears are related to hypoperfusion. A study utilizing Doppler flowmetry to analyze blood flow to the rotator cuff failed to identify any “critical” zone of hypoperfusion in a normal rotator cuff. Blood flow was found to be highest at the edges of torn rotator cuff tendons and lowest in tendons suffering from chronic impingement [15].
22.5.2 Effect of Trauma
In cadaveric studies utilizing electromagnetic tracking devices, a 2 cm rotator cuff tear was created to determine the effect on kinematics in vitro. EMG data was used to apply force through cables sewn into the muscles. The defect resulted in posterior angulation of the plane of elevation, most notably throughout the midpoint of abduction [43].
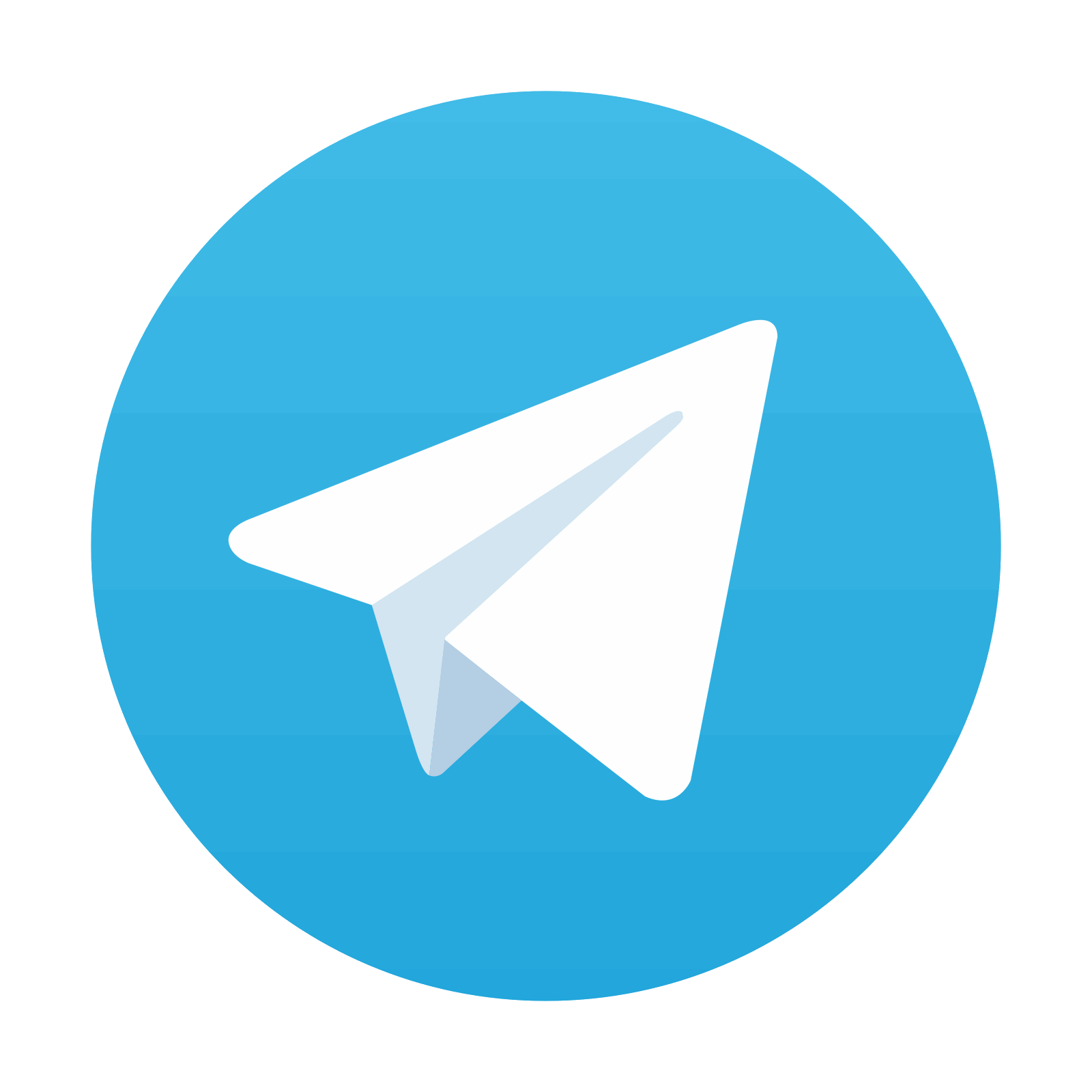
Stay updated, free articles. Join our Telegram channel

Full access? Get Clinical Tree
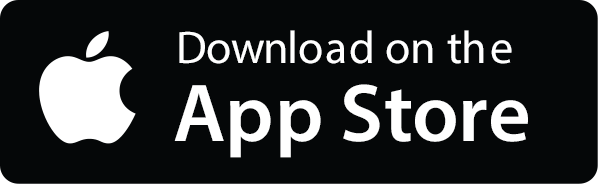
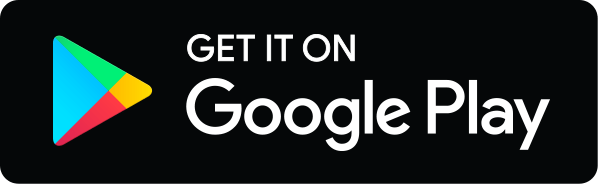