CHAPTER 5 Exercise therapy
Structure vs function
The aim of this chapter is therefore to develop the theme of ‘functional exercise therapy’, and we will begin by looking at muscle imbalance. Further details of these concepts may be found in Norris (2008).
Muscle imbalance
Basic concepts
Changes in muscle length or strength occur throughout the body in set patterns rather than purely at random. The relationship between the tone and length of muscles around a joint is known as muscle imbalance and has been described by a number of authors (Janda and Schmid, 1980; Sahrmann, 1987; Richardson, 1992; Kendall, McCreary and Provance, 1993; Comerford, 1995; Norris, 1995a). Muscles may be broadly classified into two types for convenience. Those whose actions are mainly to stabilize a joint and approximate the joint surfaces, and those responsible more for movement, which more effectively develop angular rotation. The main differences between the two types of muscles are shown in Table 5.1. As we will see this categorization helps to clarify exercise prescription but is not an exact science. Some muscles or portions of muscles fall into both camps.
Table 5.1 Muscle types (basic classification)
Stability | Movement |
---|---|
Deep | Superficial |
Slow twitch | Fast twitch |
One joint | Two joint |
Weaken and lengthen | Tighten and shorten |
Inhibited | Preferential recruitment |
Keypoint
Stability muscles hold a joint or body part firm and immobile. Movement muscles create body motion.
Classification of a muscle as a stabilizer with predominantly tonic functions refers to its most consistent response. Many muscles are able to exhibit both tonic and phasic contraction depending on requirement at the time. For example transversus abdominis shows tonic activity during gait when it contributes to spinal stability, but phasic activity associated with expiration during rapid breathing (Saunders, Rath and Hodges, 2004). Both the diaphragm (Hodges and Gandevia, 2000) and the pelvic floor muscles (Hodges, Pengel and Sapsford, 2007) exhibit tonic activity during an active arm lifting task and phasic activity during challenged breathing.
Two of the fundamental changes seen in the muscle imbalance process include tightening of the mobilizer (two-joint) muscles and laxity/loss of endurance within the inner range for the stabilizer (single-joint) muscles. These two changes are used as tests for the degree of muscle imbalance. The combination of length and tension changes alter muscle pull around a joint and so pull the joint out of alignment. Changes in body segment alignment and the ability to perform movements which dissociate one body segment from another form the bases of the third type of test used when assessing muscle imbalance (Fig. 5.1).
The mixture of tightness and weakness seen in the muscle imbalance process alters body segment alignment and changes the equilibrium point of a joint. In addition, imbalance leads to lack of accurate segmental control. The combination of stiffness (hypoflexibility) in one body segment and laxity (hyperflexibility) in an adjacent body segment leads to the establishment of relative flexibility (White and Sahrmann, 1994). In a chain of movement the body seems to take the path of least resistance, with the more flexible segment moving first and furthest. If we take as an illustration two pieces of rubber tubing (Fig. 5.2) of unequal strengths. When the movement begins at C and A is fixed, the more flexible area B–C moves more. This will still be the case if C is held still and A moves.
Taking this example into the body, Figure 5.3 shows a toe-touching exercise. The two areas of interest with relation to relative stiffness are the hamstrings and lumbar spine tissues. As we flex forwards, movement should occur through a combination of anterior pelvic tilt and lumbar spine flexion. Subjects often have tight hamstrings and looser lumbar spine tissues due to excessive bending during everyday activities. During this flexion action, greater movement, and therefore greater tissue strain, will always occur at the lumbar spine. Excessive motion at this point can lead initially to pain simply through overstretch of pain sensitive structures. In the short to mid term the tissue insult creates an inflammatory response which both maintains the pain response and causes swelling. Longer term the combination of altered movement and tissue stress may lead to overuse injury. It become apparent in the toe-touching example that relative stiffness makes the toe-touching exercise ineffective as a hamstring stretch unless the trunk muscles are tightened to stabilize the lumbar spine.
Muscle adaptation
Muscle adaptation to reduced usage has been extensively studied using immobilized limbs (Appell, 1990). The greatest tissue changes occur within the first days of disuse. Strength loss has been shown to be as much as 6% per day for the first 8 days with little further loss after this period (Muller, 1970). Greater reduction in size and loss of numbers is seen in type I fibres, with a parallel increase in type II fibres, demonstrating selective atrophy of type I fibres (Templeton et al., 1984). However, not all muscles show an equal amount of type I fibre atrophy. Atrophy is largely related to change in use relative to normal function, with the initial percentage of type I fibres that a muscle contains being a good indicator of likely atrophy pattern. Those muscles with a predominantly anti-gravity function, which cross one joint and have a large proportion of type I fibres, show greatest selective atrophy (e.g. soleus and vastus medialis). Selective wasting in the calf muscles illustrates this well, with the soleus wasting by 60% and the plantaris by only 17% (Thomason et al., 1987). Those predominantly slow anti-gravity muscles, which cross multiple joints, are next in order of atrophy (e.g. erector spinae); last are phasic, predominantly fast type II muscles which can be immobilized with less loss of strength (e.g. biceps) (Lieber, 1992).
These three categories of muscles have led to stabilizers being subdivided into primary and secondary types (Jull, 1994) as shown in Figure 5.4. Examples of the three types include multifidus, transversus abdominis and vastus medialis oblique as primary stabilizers. The gluteals and oblique abdominals are classified as secondary stabilizers, while rectus femoris and the hamstrings are mobilizers, only acting as stabilizers in conditions of extreme need.
The primary stabilizers have very deep attachments, lying close to the axis of rotation of the joint. In this position they are unable to contribute any significant torque, but will approximate the joint. In addition, many of these smaller muscles have important proprioceptive functions (Bastide, Zadeh and Lefebvre, 1989). The secondary stabilizers are the main torque producers, being large monoarticular muscles attaching via extensive aponeuroses. Their multipennate fibre arrangement makes them powerful and able to absorb large amounts of force through eccentric action. The mobilizers are fusiform in shape, with a less powerful fibre arrangement, but one which is designed for producing large ranges of motion. In addition, the mobilizers are biarticular muscles, which have their own unique biomechanical characteristics.
Selective changes in muscle may also occur as a result of training (Richardson and Bullock, 1986). In the knee, rapid flexion–extension actions have been shown to selectively increase activity in the rectus femoris and hamstrings (biarticular) but not in the vasti (monoarticular). In this study, comparing speeds of 75°/s and 195°/s, mean muscle activity for the rectus femoris increased from 23.0 uV to 69.9 uV. In contrast, muscle activity for the vastus medialis increased from 35.5 uV to only 42.3 uV (Fig. 5.5). The pattern of muscle activity was also noticeably different in this study after training. At the fastest speeds the rectus femoris and hamstrings displayed phasic (on and off) activity while the vastus medialis showed a tonic (continuous) pattern (Fig. 5.6).
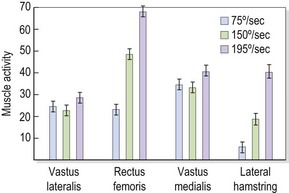
Figure 5.5 Changes in muscle activity with increases in speed.
From Richardson, C.A. and Bullock, M.I. (1986) Changes in muscle activity during fast, alternating flexion–extension movements of the knee. Scandinavian Journal of Rehabilitation Medicine, 18, 51–58. With permission.
Even in the more functional closed kinetic chain position, similar changes have been found (Ng and Richardson, 1990). A 4-week training period of rapid plantarflexion in standing gave significant increases in jump height (gastrocnemius, biarticular) but also significant losses of static function of the soleus (monoarticular).
Changes in muscle length
Chronic muscle lengthening
Stabilizer muscles tend to ‘weaken’ (sag) whereas mobilizers tend to ‘shorten’ (tighten). Taking these responses further, primary stabilizers will react quickly to pain and swelling, by inhibition. Swelling has been shown to cause a reflex inhibition of muscles in the knee (de Andrade, Grant and Dixon, 1965; Stokes and Young, 1984). In addition, marked asymmetry of the multifidus has been shown using real-time ultrasound imaging.
Adaptation of primary stabilizers of the spine
Multifidus
The cross-sectional area (CSA) of the multifidus has been shown to be substantially reduced at the level of lumbar lesion (Hides et al., 1994). The authors suggested that the mechanism for the CSA reduction was inhibition through perceived pain via a long loop reflex.
In addition to changes in muscle bulk, alteration in fibre type has been shown in the multifidus in patients with low back pain (LBP) (Biedermann et al., 1991). A reduced ratio of slow twitch to fast twitch muscle fibres was shown, possibly as an adaptive response by the muscle to changes in functional demand placed upon it. Furthermore, injury may have caused a shift in the recruitment patterns of the motor units of the paraspinal muscles, with the fast twitch motor units being recruited before the slow twitch units.
Posture has also been shown to affect the multifidus. In a study of 20 healthy subjects, O’Sullivan et al. (2002) showed a reduction in activity of the multifidus (together with internal oblique and the thoracic erector spinae) in a sway-standing posture, indicating the importance for postural retraining when facilitating the muscle for rehabilitation. Prolonged flexion activities initially result in a reflex spasm of the multifidus, which reduces substantially if the posture is maintained. Williams et al. (2000) used a cat model to investigate sustained moderate flexion stress on seven preparations. They showed a reduction to 5% of this initial value within 3 minutes of taking up the posture, leading to tissue laxity and a loss of reflex protective muscle activity. Prolonged flexion (20 minutes, using cat preparation) has also been shown to result in multifidus spasm (Jackson et al., 2001), with full recovery not seen for 7 hours after initiation of rest.
Injection of hypertonic saline (a chemical irritant that has an effect similar to swelling) into the multifidus has shown that the muscle can be a source of both local and referred pain (Cornwall, John-Harris and Mercer, 2006), and some authors recommend dry needling the muscle in cases of acute low back pain (Gunn, 1996). Retraining of the muscle may be through isolated contraction initially, but successful rehabilitation involves use of the muscle during gross movement (Danneels, Vanderstraeten and Cambier, 2003).
Transversus abdominis
The transversus abdominis shows a similar response in the chronic LBP patient. Normally, this muscle acts as a primary stabilizer of the lumbar spine (Jull, 1994). It is active in both flexion and extension of the lumbar spine (Cresswell, Grundstrom and Thorstensson, 1992) and during action of the upper limb and lower limb in multiple directions (Hodges, Richardson and Jull, 1996). In addition, contraction of transversus abdominis precedes that of the other abdominal and lumbar extensor muscles (Cresswell, Oddsson and Thorstensson, 1994; Hodges and Richardson, 1996). Its primary function would seem to be to contract in response to forces applied to the trunk. In this way it is anticipating the requirement of stability and providing it.
Following LBP, transversus abdominis function changes considerably (Hodges and Richardson, 1995; Hodges, Richardson and Jull, 1996). Timing of onset of transversus contraction is delayed by a mean of 129 ms, while the action of the other abdominals is largely unchanged. When assessed in a hollowing action the transversus muscle shows a smaller increase in thickness measured by real-time ultrasound (between rest and contraction) in patients with low back pain than in normal subjects. Normal subjects showed a mean thickness increase of 49.7% while low back patients showed mean values of 19.15% (Critchley and Coutts, 2002). Re-education of transversus contraction is one important component in low back pain rehabilitation, but reliance on muscle isolation has been shown to be ineffective when compared to more general back stability programmes (see Chapter 14). Even authors who once advocated muscle isolation alone (Hodges and Richardson, 1996) now acknowledge that using this type of action as a primary exercise and then discharging the patient is erroneous (Hodges, 2009).
Quadratus lumborum
The quadratus lumborum (QL) is a muscle which has been described both as a movement muscle tending towards tightness (Janda, 1993) and as an important stabilizer in functional lifting tasks (McGill, Juker and Kropf, 1996). The medial fibres of the muscle connect directly to the lumbar transverse processes, therefore having the potential to stabilize the spine. The lateral fibres run between the ilium and iliolumbar ligament and the 12th rib, spanning the spine and acting as a movement muscle. The muscle is therefore separated into two functional components (Bergmark, 1989). The medial fibres can contribute directly to segmental support of the spine (Richardson et al., 1999), while the lateral portion may tend towards tightness and developing trigger points (Janda, 1993; Gunn, 2000).
The QL has been shown to be an important stabilizer of the spine in side carrying tasks especially (McGill, Juker and Kropf, 1996; McGill, 2000). It is important to note that when the spine fails (buckles) due to compressive loads, it does so laterally in the first instance, so a muscle which resists lateral forces is likely to be an important stabilizer when carrying loads. In addition, the QL functions differently to the erector spinae in forward bending, as it does not demonstrate a flexion relaxation response (Andersson et al., 1996). Whether the muscle reacts to injury and underusage in the same way as the transversus or multifidus is as yet uncertain. Categorization of the medial portion of the muscle as a stabilizer is certain, but sub-categorization into a primary or secondary stabilizer will require more evidence (Bullock-Saxton et al., 2000).
Adaptation of secondary stabilizers
The secondary stabilizer muscles show a tendency to lengthen and weaken. As postural muscles they almost seem to give way to the pull of gravity and ‘sag’. This reaction has been termed stretch weakness (Kendall, McCreary and Provance, 1993). The muscle has remained in an elongated position, beyond its normal resting position, but within its normal range. This is differentiated from overstretch where the muscle is simply elongated or stretched beyond its normal range.
The length–tension relationship of a muscle dictates that a stretched muscle, where the actin and myosin filaments are pulled apart, can exert less force than a muscle at normal resting length. Where the stretch is maintained, however, this short-term response (reduced force output) changes to a long-term adaptation. The muscle tries to move its actin and myosin filaments closer together, and to do this, it must add more sarcomeres to the ends of the muscle (Fig. 5.7). This adaptation, known as an increase in serial sarcomere number (SSN), changes the nature of the length–tension curve.
Long-term elongation of this type causes a muscle to lengthen by the addition of up to 20% more sarcomeres (Gossman, Sahrmann and Rose, 1982). The length–tension curve of an adaptively lengthened muscle moves to the right (Fig. 5.8). The peak tension that such a muscle can produce in the laboratory situation is up to 35% greater than that of a normal length muscle (Williams and Goldspink, 1978). However, this peak tension occurs at approximately the position where the muscle has been immobilized (point A, Fig. 5.8). If the strength of the lengthened muscle is tested with the joint in mid-range or inner-range (point B, Fig. 5.8), as is common clinical practice, the muscle cannot produce its peak tension, and so the muscle appears ‘weak’. For this reason, manual muscle tests have been described as being more accurate indicators of positional (rather than total) strength (Sahrmann, 1987).
In the laboratory situation the lengthened muscle will return to its optimal length within approximately 1 week if once more placed in a shortened position (Goldspink, 1992). Clinically, restoration of optimal length may be achieved by either immobilizing the muscle in its physiological rest position (Kendall, McCreary and Provance, 1993) and/or exercising it in its shortened (inner-range) position (Sahrmann, 1990). Enhancement of strength is not the priority in this situation; indeed, the load on the muscle may need to be reduced to ensure correct alignment of the various body segments and correct performance of the relevant movement pattern.
Immobilization of cat hind limb in a lengthened position (4 weeks) showed a 19% increase in SSN of the soleus muscle but no change in individual sarcomere length. Immobilizing in a shortened position gave a 40% decrease in SSN, again with no change in sarcomere length (Tabary, 1972). It has been argued that this type of adaptation enables the muscle to develop maximum tension when movement of the muscle is limited (Jaspers, Fagan and Tischler, 1985).
SSN may be partly responsible for changes in muscle strength without parallel changes in hypertrophy (Koh, 1995). SSN exhibits marked plasticity and may be influenced by a number of factors. For example, immobilization of rabbit plantarflexors in a lengthened position showed an 8% increase in SSN in only 4 days, while applying electrical stimulation to increase muscle force showed an even greater increase (Williams et al., 1986). Stretching a muscle appears to have a greater effect on SSN than does immobilization in a shortened position. Following immobilization in a shortened position for 2 weeks, the mouse soleus has been shown to decrease SSN by almost 20% (Williams, 1990). However, stretching for just 1 hour per day in this study not only eliminated the SSN reduction but actually produced nearly a 10% increase in SSN.
An eccentric stimulus may cause a greater adaptation of SSN than a concentric stimulus. Morgan and Lynn (1994) subjected rats to uphill or downhill running, and showed SSN in the vastus intermedius to be 12% greater in the eccentric trained rats after 1 week. In contrast to this, however, Koh and Herzog (1998) used the dorsiflexor muscles of the rabbit to assess the effect of eccentric training on SSN. Using a 2-week training session over a total of 12 weeks, they found little effect on SSN or fibre length, but the variation between the studies may well be related to species or training protocol differences.
It has been suggested that if SSN adaptation occurs in humans, strength training might produce this type of change if it is performed at a joint angle different from that at which the maximal force is produced during normal activity (Koh, 1995).
Shortening posturally lengthened muscle
Inner-range holding may or may not represent a reduction in SSN, but is a required functional improvement in postural control. Muscle shortening may certainly be achieved through splinting. Muscles immobilized in a shortened position in this way show loss of sarcomeres in series within 14–28 days (Tabary, 1972; Williams and Goldspink, 1978). With training, there is less evidence for reduction in SSN in humans. Muscle shortening has been shown in the dorsiflexors of horse riders. Clearly, this position is not held permanently as with splinting, but rather shows a training response. Following pregnancy, SSN increases in the rectus abdominis in combination with diastasis. Again, the length of the muscle gradually reduces in the months following birth. It is generally thought that inner-range training is likely to shorten a lengthened muscle, although the precise method through which this adaptation is achieved in humans is not certain (Goldspink, 1996). The treatment aim for a posturally lengthened muscle must ultimately be to change its resting length and therefore correct segmental alignment. In so doing the joints within a body region will be able once more to move through an optimal movement range (Sahrmann, 2002).
Inner-range holding ability
The ability of a stabilizer muscle to maintain an isometric contraction at low load over a period of time is vital to its anti-gravity function (Richardson, 1992). This may be assessed by using the classic muscle test position (see Appendix) and asking the subject to maintain a contraction in full inner range (Richardson and Sims, 1991). The important factor in the assessment is the length of time a static hold can be maintained without jerky (phasic) movements occurring. In each case the limb is placed passively into full inner range. When released, if the limb drops, the passive range of motion differs from the active range, which is an important indicator of poor stabilizer function. Full stabilizing function is achieved when a subject can maintain the inner-range position for 10 repetitions, each of 10 seconds duration (Jull, 1994). Often the first two or three repetitions are performed normally, and it is only with further repetition that the deficit becomes apparent.
Tests for the major stabilizing muscles
Lower limb
Iliopsoas
The iliopsoas (IP) is assessed in the sitting position. The patient flexes the hip while maintaining a 90° knee flexion so that the foot is lifted clear of the ground (Fig. 5.9A). Where the IP is lengthened the thigh may drop down from the inner range position, or more commonly the pelvis is tilted backwards while the knee position is maintained. Backward tilting of the pelvis accompanied by flattening of the lumbar lordosis moves the origin of the muscle away from its insertion and lengthens the IP.
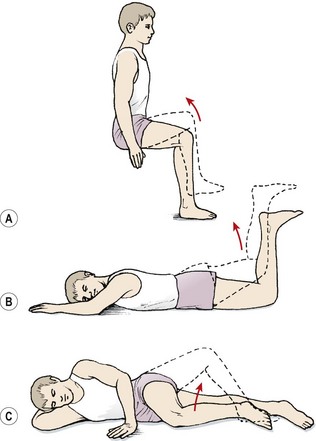
Figure 5.9 Inner-range holding of the lower limb. (A) Iliopsoas. (B) Gluteus maximus. (C) Gluteus medius.
The stabilizing function of psoas major (Gibbons, 2007) may be rehabilitated using a leg shortening action, asking the patient to imagine ‘sucking the hip back into its socket’ (see Chapter 14).
Gluteus maximus
The gluteus maximus is assessed in prone lying with the knee flexed to 90°. Flexing the knee shortens the hamstring muscles, placing them at a physiological disadvantage and making hamstring substitution less likely. The hip is lifted to inner-range extension and held (Fig. 5.9B). In this position, the gluteus maximus may be palpated; often, with a chronic low back pain patient, little activity is noted. Where the muscle is working, it may still not be able to maintain the inner range position over time. Here, the inner range position is held for 1–2 seconds and the limb slowly sinks to a lower position and is held at this mid-range angle. As endurance of the muscle fails, muscle shaking is evident as the large diameter fast twitch fibres of the muscle are used.
Gluteus medius
The gluteus medius is tested in side lying with the knee flexed. The action is combined with hip abduction, with slight lateral rotation to emphasize the posterior fibres of the muscle (Fig. 5.9C). Two starting positions may be used, both with the foot supported to work the limb in closed chain format. In the first the feet are placed together; in the second the foot of the upper leg is placed on the couch at mid-shin level. The action is to keep the foot still and lift the knee while keeping the trunk still. Rotation of the trunk at the pelvis must be avoided. The therapist should monitor the position of the greater trochanter of the upper leg and ensure that it points to the ceiling and does not move forwards or backwards.
Cervical spine
For the cervical spine the essential stabilizers are the deep neck flexors. These are retrained in the supine position using pressure biofeedback, with the cuff placed behind the head or upper cervical spine (Jull, 1994). The aim is to achieve suboccipital rather than lower cervical flexion. The action is a minimal flexion or ‘nodding’ action of the head alone, avoiding forceful actions or lifting the head from the couch.
Overactivity of the superficial muscles with trigger point development is common. The sternomastoid and anterior scalene especially may be shortened in a head held forward (HHF) posture. In addition, tightness and thickening are seen in the splenius capitis and splenius cervicis, as well as the semispinalis capitis and levator scapuli (Gunn, 1996).
Upper trunk
A blanket assessment of scapular stability may be usefully made in prone falling (Fig. 5.10). Slowly lowering (eccentric push-up) will often reveal inappropriate movement of the scapulae in the first phase of the action. The scapulae should remain apart, and firmly fixed to the thoracic wall throughout the movement. Scapular movement outward and upward indicates lack of stability (Janda, 1994). If the scapulae fall together, the rhomboids and levator scapulae are dominant (Kendall, McCreary and Provance, 1993). True winging, apparent through lifting of the medial edge of the scapula, represents serratus anterior inactivity. Retraining scapular stability is covered in Chapter 17 Treatment note 17.1.
Lower trunk
Inner range holding of the lower trunk forms the basis of stabilization training and is covered in Chapter 13. A blanket assessment may be made (i) by using an isolation action such as the heel slide, or (ii) by assessing lumbopelvic alignment during whole body tasks. An inability to maintain the optimal alignment of the lumbar spine during whole body movements suggests instability and warrants closer examination. Spinal stabilization is covered in Chapter 13.
Muscle shortening
Mobilizer muscles have long fusiform shapes and, as such, show a tendency to tighten. Tightness in the hamstrings, for example (mobilizers, biarticular), is often seen, while tightness in the gluteals (stabilizers, monoarticular) is rare. As well as reducing range of motion, the tightened muscle is more likely to develop painful trigger points (Travell and Simons, 1983) These are small hypersensitive regions within a muscle which stimulate afferent nerve fibres, causing pain. The sensation created is a deep tenderness with an overlying increase in tone creating a palpably tender band of muscle. When palpated deeply, the trigger point creates a local muscle spasm, giving the ‘jump sign’ (Janda, 1993). The irritability threshold of a tight muscle is lowered, causing it to be activated earlier than normal in a movement sequence. One of the reasons for this is that, being tight, there is less ‘slack’ to take up in the muscle before contraction begins. In addition, the muscle shows an increased afferent input via the stretch receptors (Sahrmann, 1990).
Increased tone within the mobilizer muscle can close down the local capillary bed disrupting blood flow into and out of the working muscle tissue. The result is ischaemic pain which is dull, aching and diffuse in nature, often seen at rest. This is overlaid by the acute trigger point pain which is highly localized and may occur at the initiation of movement and may show a referral pattern (see Fig. 5.35).
The lower limb
Thomas test
The Thomas test used here is modified from the original test first described by Jones and Lovett (1929). With the modified Thomas test (Fig. 5.11), the patient begins in crook lying at the end of the couch. The knees are brought to the chest and the back flattened to a point where the sacrum just begins to lift away from the couch surface but no further. One leg is held close to the chest to maintain the pelvic position and the other leg is straightened over the couch end. An optimal alignment exists when the femur lies horizontally, and aligned to the sagittal plane (no abduction). The tibia should lie vertically (90° knee flexion) and be aligned with the sagittal plane (no hip rotation), as shown in Fig. 5.11. If the femur rests above the horizontal and the knee is flexed less than 90°, tightness may be present in either the iliopsoas or rectus femoris. If the rectus is tight, straightening the knee will take the stretch off the muscle and the leg will drop down. If the knee is straightened and the leg stays in place, it indicates tightness in the iliopsoas. Deviation of the knee laterally (the ‘J’ sign) indicates a possible tightness in the iliotibial band (ITB), indicating that the Ober test should be performed to confirm the tightness. The Thomas test has been shown to be a repeatable measure using 11 subjects over a sequence of 10 trials (Bullock-Saxton and Bullock, 1994).
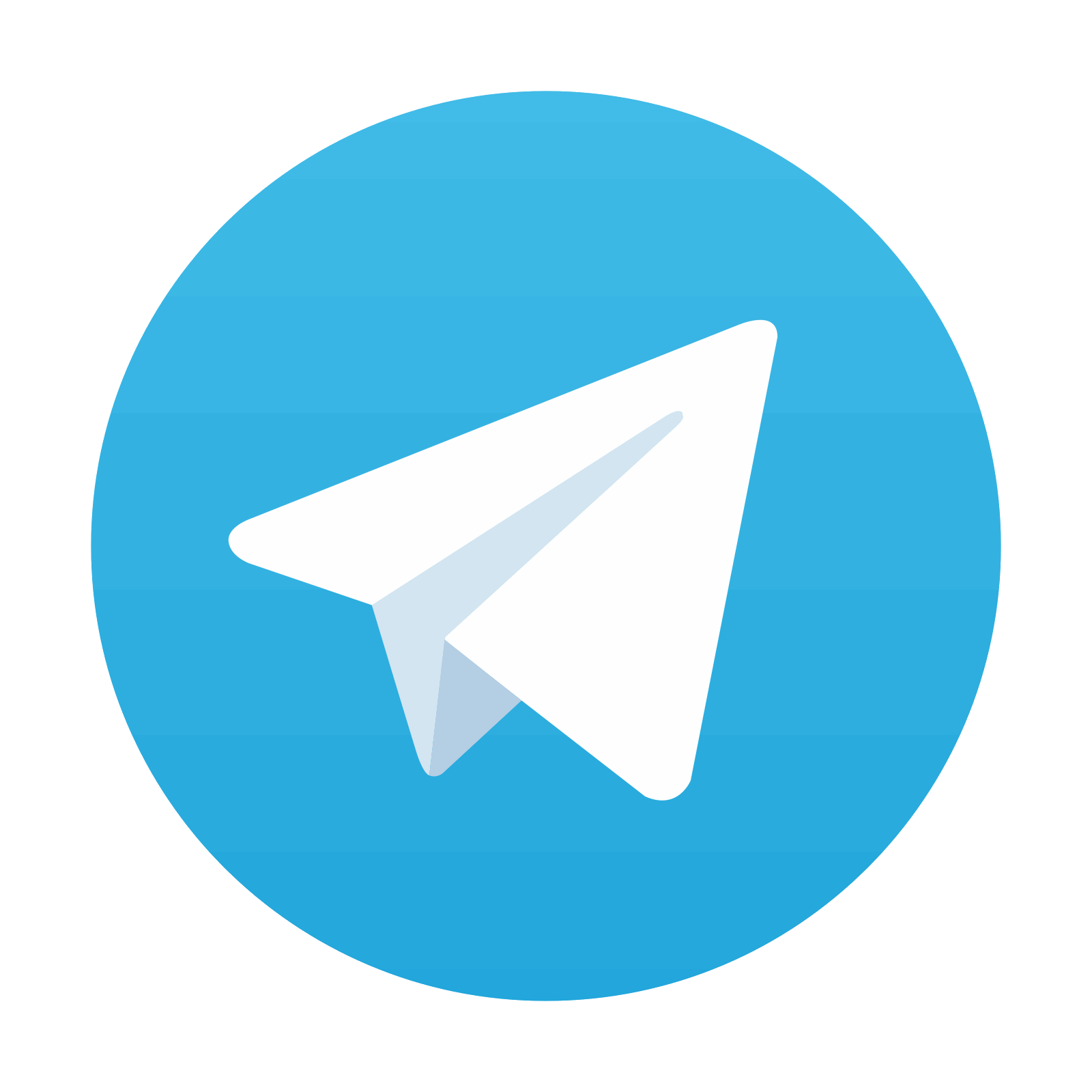
Stay updated, free articles. Join our Telegram channel

Full access? Get Clinical Tree
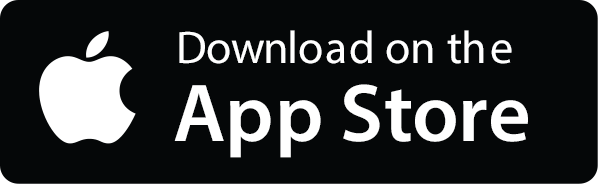
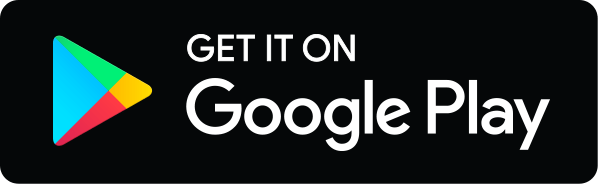
