3 Cartilage and Chondrocytes
After mesenchymal condensation and chondroprogenitor cell differentiation, the chondrocytes undergo proliferation, terminal differentiation to chondrocyte hypertrophy, and apoptosis through a process termed endochondral ossification, whereby the hypertrophic cartilage is replaced by bone. A similar sequence of events occurs in the postnatal growth plate and leads to rapid growth of the skeleton. Processes that control the different stages of skeletal development are described in Chapter 1.
In adults, the anatomic distribution of cartilage is restricted primarily to the joints, trachea, and nasal septum, where the major function is structural support. In joints, cartilage has the additional function of providing low-friction articulation. Adult articular cartilage comprises a specialized matrix of collagens, proteoglycans, and other cartilage-specific and nonspecific proteins. Adult articular chondrocytes, remnants of the resting, or reserve, chondrocytes that laid down the original cartilage matrix during chondrogenesis, are inactive metabolically, owing partially to the absence of a vascular supply and innervation in the tissue.1 The clinical importance of the adult chondrocyte resides in its capacity to respond to mechanical stimuli, growth factors, and cytokines that may influence normal homeostasis in a positive or negative manner. In rheumatoid arthritis (RA), cartilage destruction occurs primarily in areas contiguous with the proliferating synovial pannus, although evidence indicates that the chondrocyte can respond to the inflammatory milieu and participate in degrading its own matrix.2 In osteoarthritis (OA), the chondrocyte plays a key role by reacting to structural changes in the surrounding cartilage matrix through the production of catabolic cytokines and anabolic factors, which act in an autocrine-paracrine manner.3,4 Nevertheless, the chondrocyte has limited capacity, which declines with age, to regenerate the normal cartilage architecture with zonal variations in the matrix network that was formed originally. This chapter focuses on the structure and function of normal articular cartilage and the role of the chondrocyte in maintaining cartilage homeostasis and responding to adverse environmental insults that may modify cartilage integrity.
Cartilage Structure
Normal articular cartilage is a specialized tissue characterized macroscopically by its milky, shelled-almond (hyaline) appearance. It is an avascular tissue nourished by diffusion from the vasculature of the subchondral bone and from the synovial fluid. Articular cartilage is more than 70% water, and it is hypocellular compared with other tissues; chondrocytes constitute only 1% to 2% of its total volume.5,6 Most of the dry weight of cartilage consists of two components: type II collagen and the large aggregating proteoglycan, aggrecan. Several “minor” collagens and small proteoglycans also seem to play a role in cartilage-matrix organization, however.7,8
Organic constituents represent only about 20% of the wet weight of cartilage. Collagen, primarily type II, accounts for approximately 15% to 25% of the wet weight and about half of the dry weight except in the superficial zone, where it represents most of the dry weight. Proteoglycans, primarily aggrecan, account for 10% of the wet weight and about 25% of the dry weight. The highly cross-linked type II collagen–containing fibrils form a systematically oriented network that traps the highly negatively charged proteoglycan aggregates.9 Histochemical analysis of cartilage shows that proteoglycans can be stained reliably with safranin O, toluidine blue, or Alcian blue, although at low substrate concentrations, these methods are not stoichiometric.10 Collagen also can be stained efficiently, but differentiation of collagen types requires immunostaining with specific antibodies.
Despite its thinness (≤7 mm) and apparent homogeneity, mature articular cartilage is a heterogeneous tissue with four distinct regions: (1) the superficial tangential (or gliding) zone, (2) the middle (or transitional) zone, (3) the deep (or radial) zone, and (4) the calcified cartilage zone, which is located immediately below the tidemark and above the subchondral bone (Figure 3-1).6,9,11,12 In the superficial zone, the chondrocytes are flattened, and the matrix comprises thin collagen fibrils in tangential array, associated with a high concentration of the small proteoglycan decorin and a low concentration of aggrecan. The middle zone, composing 40% to 60% of cartilage weight, consists of rounded chondrocytes surrounded by radial bundles of thick collagen fibrils. In the deep zone, chondrocytes frequently are grouped in columns or clusters (Figure 3-2). In this region, collagen bundles are the thickest and are arranged in a radial fashion.
Cell density progressively decreases from the surface to the deep zone, where it is one-half to one-third the density in the superficial zone13; chondrocytes in the deep and middle zones have a cell volume that is twice that of superficial chondrocytes.14 Water is 75% to 80% of the wet weight in the superficial zone and progressively decreases to 65% to 70% with increasing depth. Greater amounts of collagen relative to proteoglycans are present in the superficial zone, compared with the middle and deep zones, and type I collagen may be synthesized, in addition to type II collagen.15,16 With increasing depth, the proportion of proteoglycan increases to 50% of the dry weight in the deep zone.14,17–19 The calcified zone is formed as a result of endochondral ossification and persists after growth plate closure as the histologically defined tidemark.20 The calcified zone serves as an important mechanical buffer between uncalcified articular cartilage and subchondral bone.
The physical properties of articular cartilage are determined by the unique fibrillar collagen network, which provides tensile strength, interspersed with proteoglycan aggregates that bestow compressive resilience.21–23 Proteoglycans are associated with large quantities of water bound to the hydrophilic glycosaminoglycan chains. This cartilaginous extracellular matrix (ECM), with its tightly bound water, provides a high degree of resistance to deformation by compressive forces. The capacity to resist compressive forces is associated with the ability to extrude water as the cartilage compresses. When compression is released, the proteoglycans (now depleted of balancing counter ions that were removed with the water) contain sufficient fixed charge to reabsorb osmotically the water and small solutes into the matrix, which then rebounds to its original dimensions.24,25
Structure-Function Relationships Of Cartilage Matrix Components
ECM components synthesized by chondrocytes include highly cross-linked fibrils of triple-helical type II collagen molecules that interact with other collagens, aggrecan, small proteoglycans, and other cartilage-specific and nonspecific matrix proteins (Table 3-1).5,7,8,11 The importance of these structural proteins may be observed in heritable disorders, such as chondrodysplasias, or in transgenic animals in which mutations or deficiencies in cartilage genes result in cartilage abnormalities.26–30 Deficiencies or disruptions in genes that encode the cartilage-specific collagens result, in some cases, in premature OA.28 Knowledge of the composition of the cartilage matrix has permitted the development of methods for identifying molecular markers in serum and synovial fluid that can be used to monitor changes in cartilage metabolism and to assess cartilage damage in OA or RA.31–34 Changes in the structural composition of cartilage can markedly affect its biomechanical properties (see Chapter 6).
Table 3-1 Extracellular Matrix Components of Cartilage
Molecule | Structure and Size | Function and Location |
---|---|---|
Collagens | ||
Type II | [α1(II)]3; fibril-forming | Tensile strength; major component of collagen fibrils |
Type IX | [α1(IX)α2(IX)α3(IX)]; single CS or DS chain; α1(II) gene encodes α3(IX); FACIT | Tensile properties, interfibrillar connections; cross-links to surface of collagen fibril, NC4 domain projects into matrix |
Type XI | [α1(XI)α2(XI)α3(XI)]; fibril-forming | Nucleation/control of fibril formation; within collagen fibril |
Type VI | [α1(VI)α2(VI)α3(VI)]; microfibrils | Forms microfibrillar network, binds hyaluronan, biglycan, decorin; pericellular |
Type X | [α1(X)]3; hexagonal network | Support for endochondral ossification; hypertrophic zone and calcified cartilage |
Type XII | [α1(XII)]3; FACIT large cruciform NC3 domain | Associated with type I collagen fibrils in perichondrium and articular surface |
Type XIV | [α1(XIV)]3; FACIT | Associated with type I collagen; superficial zone |
Type XVI | [α1(XVI)]3; FACIT | Integrates with collagen II/XI fibrils |
Type XXVII | Col27a1 gene: 156 kb, 61 exons | Fibril-forming; developing cartilage |
Proteoglycans | ||
Aggrecan | 255 kD core protein; CS/KS side chains; C-terminal EGF and lectin-like domains | Compressive stiffness through hydration of fixed charge density; binding through G1 domain to HA stabilized by link protein |
Versican | 265-370 kD core protein; CS/DS side chains; C-terminal EGF, C-type lectin, and CRP-like domains | Low levels in articular cartilage throughout life; calcium-binding and selectin-like properties |
Perlecan | 400-467 kD core protein; HS/CS side chains; no HA binding | Cell-matrix adhesion; pericellular |
Biglycan | 38 kD; LRR core protein with two DS chains (76 kD) | Binds collagen VI and TGF-β; pericellular |
Decorin | 36.5 kD; LRR core protein with one CS or DS side chain (100 kD) | Controls size/shape of collagen fibrils, binds collagen II and TGF-β; interterritorial |
Asporin | 40 kD; LRR core protein; N-terminal extension of 15 aspartate residues | Binds collagen, modulates TGF-β function |
Fibromodulin | 42 kD; containing KS chains in central LRR region and N-terminal tyrosine sulfate domains | Same as decorin |
Lumican | 38 kD; structure similar to fibromodulin | Same as decorin |
PRELP | 44 kD; LRR core protein; proline-rich and arginine-rich N-terminal binding domain for heparin and HS | Mediates cell binding through HS in syndecan |
Chondroadherin | 45 kD; LRR core protein without N-terminal extension | Binding to cells via α2β1 integrin |
Other Molecules | ||
Hyaluronic acid (HA; hyaluronan) | 1000-3000 kD | Retention of aggrecan within matrix |
Link protein | 38.6 kD | Stabilizes attachment of aggrecan G1 domain to HA |
Cartilage oligomeric matrix protein (COMP) | 550 kD; five 110-kD subunits; thrombospondin-like | Interterritorial in articular cartilage; stabilizes collagen network or promotes collagen fibril assembly; calcium binding |
Cartilage matrix protein (CMP, or matrilin-1); matrilin-3 | Three 50-kD subunits with vWF and EGF domains | Tightly bound to aggrecan in immature cartilage |
Cartilage intermediate layer protein (CILP) | 92 kD; homology with nucleotide pyrophosphohydrolase without active site | Restricted to middle/deep zones of cartilage; increase in early and late osteoarthritis |
Glycoprotein (gp)-39, YKL-40, or chitinase 3-like protein 1 (CH3L1) | 39 kD; chitinase homology | Marker of cartilage turnover; chondrocyte proliferation; superficial zone of cartilage |
Fibronectin | Dimer of 220 kD subunits | Cell attachment and binding to collagen and proteoglycans; increased in osteoarthritis cartilage |
Tenascin-C | Six 200-kD subunits forming hexabrachion structure | Binds syndecan-3 during chondrogenesis; angiogenesis |
Superficial zone protein (SZP), lubricin, or proteoglycan (PRG) 4 | 225 kD, 200 nm length | Joint lubrication; superficial zone only |
Membrane Proteins | ||
CD44 | Integral membrane protein with extracellular HS/CS side chains | Cell-matrix interactions; binds HA |
Syndecan-1, -3, -4 | N-terminal HS attachment site; cytoplasmic tyrosine residues | Syndecan-3 is receptor for tenascin-C during cartilage development; cell-matrix interactions |
Anchorin CII (or annexin V) | 34 kD; homology to calcium-binding proteins calpactin and lipocortin | Cell surface attachment to type II collagen; calcium binding |
Integrins (α1, α2, α3, α5, α6, α10; β1, β3, β5) | Two noncovalently linked transmembrane glycoproteins (α and β subunits) | Cell-matrix binding: α1β1/collagen I or VI, α2β1 or α3β1/collagen II, α5β1/fibronectin; intracellular signaling |
Discoidin domain receptor 2 | Receptor tyrosine kinase | Binds native type II collagen fibrils; Ras/ERK signaling |
CRP, complement regulatory protein; CS, chondroitin sulfate; DS, dermatan sulfate; EGF, epidermal growth factor; FACIT, fibril-associated collagens with interrupted triple helices; HA, hyaluronic acid; HS, heparan sulfate; KS, keratan sulfate; LRR, leucine-rich repeat; NC, noncollagen; PRELP, proline-rich and arginine-rich end leucine-rich repeat protein; TGF, transforming growth factor; vWF, von Willebrand factor.
Cartilage Collagens
The major component of the collagen network in adult articular cartilage is the triple-helical type II collagen molecule, which is composed of three identical α chains (α1[II])3. These molecules are assembled in fibrils in a quarter-stagger array that can be observed by electron microscopy.7,35,36 These fibrils are thinner than type I collagen–containing fibrils in skin because of the higher numbers of hydroxylysine residues that can form cross-links and the presence of other collagen and noncollagen components in the fibril. Type IIB collagen in articular cartilage is a product of alternative splicing and lacks a 69 amino acid, cysteine-rich domain of the amino-terminal propeptide, which is encoded by exon 2 in the human type II collagen gene (COL2A1).37 This domain is found in type IIA procollagen, which is expressed by chondroprogenitor cells during development, and in the amino propeptides of other interstitial collagen types, and may play a feedback-inhibitory role in collagen biosynthesis. The reappearance of type IIA collagen in the midzone pericellular matrix and type X collagen, the hypertrophic chondrocyte marker, in the deep zone of OA cartilage suggests reversion to a developmental phenotype in an attempt to repair the damaged matrix.38,39
Although collagens VI, IX, XI, XII, and XIV are quantitatively minor components, they may have important structural and functional properties. Collagens IX and XI are specific to cartilage, whereas collagens VI, XII, and XIV are widely distributed in other connective tissues.7 Collagen VI, which is present in cartilage as microfibrils in very small quantities in the pericellular matrix, may play a role in cell attachment and interacts with other matrix proteins, such as hyaluronan, decorin, and biglycan. Small amounts of collagen III are found in cartilage, and collagen VI may increase in OA cartilage.39
Type IX collagen is a proteoglycan and a collagen because it contains a chondroitin sulfate chain attachment site in one of the noncollagen domains. The helical domains of the type IX collagen molecule form covalent cross-links with type II collagen telopeptides and are attached to the fibrillar surface, as observed by the electron microscope. Type IX collagen may function as a structural intermediate between type II collagen fibrils and the proteoglycan aggregates, serving to enhance the mechanical stability of the fibril network and resist the swelling pressure of the trapped proteoglycans. Destruction of type IX collagen accelerates cartilage degradation and loss of function.7,35,36
The α3 chain of type XI collagen has the same primary sequence as the α1(II) chain, and the heterotrimeric type XI collagen molecule is buried in the same fibril as type II collagen. Type XI collagen may have a role in regulating fibril diameter. The more recently discovered nonfibrillar fibril-associated collagens with interrupted triple helices (FACIT), XII and XIV, which are structurally related to type IX collagen, do not form fibrils by themselves but co-aggregate with fibril-forming collagens and modulate the packing of collagen fibers through domains projecting from their surfaces.7,35,36
Cartilage Proteoglycans
The major proteoglycan in articular cartilage is the large aggregating proteoglycan, or aggrecan, which consists of a core protein of 225 to 250 kD with covalently attached side chains of glycosaminoglycans, including approximately 100 chondroitin sulfate chains, 30 keratan sulfate chains, and shorter N-linked and O-linked oligosaccharides.5,8,40,41 Link protein, a small glycoprotein, stabilizes the noncovalent linkage between aggrecan and hyaluronic acid (also called hyaluronan) to form the proteoglycan aggregate that may contain 100 aggrecan monomers. The G1 and G2 N-terminal globular domains of aggrecan and its C-terminal G3 domain have distinct structural properties that function as integral parts of the aggrecan core protein and contribute cleavage products that accumulate with age or in OA. The G2 domain is separated from G1 by a linear interglobular domain and has two proteoglycan tandem repeats. The G3 domain contains sequence homologies to epidermal growth factor, lectin, and complement regulatory protein, and participates in growth regulation, cell recognition, intracellular trafficking, and recognition, assembly, and stabilization of the ECM. About half of the aggrecan molecules in adult cartilage lack the G3 domain, probably as a result of proteolytic cleavage during matrix turnover. Small quantities of other large proteoglycans are found in cartilage, including versican, which forms aggregates with hyaluronic acid, and perlecan, which is nonaggregating; however, these proteoglycans function primarily during skeletal development, where versican is expressed in prechondrogenic condensations, and perlecan is expressed in the cartilage anlagen after expression of type II collagen and aggrecan.41
The nonaggregating small proteoglycans are not specific to cartilage, but in cartilage they serve specific roles in matrix structure and function, primarily by modulating collagen-fibril formation.42–44 Of the more than 10 leucine-rich repeat (LRR) proteoglycans discovered so far, only osteoadherin is not present in cartilage. The 24 amino acid central LRR domain is conserved, but the N-terminal and C-terminal domains have patterns of cysteine residues involved in intrachain disulfide bonds that distinguish the four subfamilies: (1) biglycan, decorin, fibromodulin, and lumican; (2) keratocan and proline and arginine-rich end leucine-rich repeat protein (PRELP); (3) chondroadherin; and (4) epiphycan/PG-Lb and mimecan/osteoglycin. Biglycan may have two glycosaminoglycan chains—chondroitin sulfate or dermatan sulfate, or both—attached near the N-terminus through two closely spaced serine-glycine dipeptides. Decorin contains only one chondroitin sulfate or dermatan sulfate chain. Fibromodulin and lumican contain keratan sulfate chains linked to the central domain of the core protein and several sulfated tyrosine residues in the N-terminus. Negatively charged glycosaminoglycan side chains contribute to the fixed charge density of the matrix and, together with the highly anionic tyrosine-sulfation sites, permit multiple-site linkage between adjacent collagen fibrils, stabilizing the network. Decorin, the most extensively studied LRR proteoglycan, binds to collagens II, VI, XII, and XIV, and to fibronectin and thrombospondin. Biglycan, decorin, and fibromodulin bind transforming growth factor (TGF)-β and the epidermal growth factor receptor and may modulate growth, remodeling, and repair. PRELP and chondroadherin may regulate cell-matrix interactions through binding to syndecan and α2β1 integrin.
Other Extracellular Matrix and Cell Surface Proteins
Several other noncollagenous matrix proteins may play important roles in determining cartilage matrix integrity. Cartilage oligomeric protein (COMP), a member of the thrombospondin family, is a disulfide-bonded, pentameric, 550 kD, calcium-binding protein that constitutes approximately 10% of the noncollagenous, nonproteoglycan protein in normal adult cartilage. COMP is located in the interterritorial matrix of adult articular cartilage, where it interacts with the COL3 and NC4 domains of type IX collagen that protrude from the fibril, stabilizing the collagen network. COMP is pericellular in the proliferating region of the growth plate, where it may have a role in cell-matrix interactions.45 The cartilage matrix protein (or matrilin-1) and matrilin-3 are expressed in cartilage at certain stages of development and are present in tracheal cartilage. Matrilin-1 is present in the pericellular matrix of adult articular cartilage.46,47
Tenascin-C, a glycoprotein that is regulated in development, is characteristic of nonossifying cartilage.48 Similar to fibronectin, alternative splicing of tenascin-C mRNA gives rise to different protein products at different stages of chondrocyte differentiation. Both proteins are increased in OA cartilage and may serve specific functions in remodeling and repair. A splice variant of tenascin-C mRNA is found in chondrosarcomas.49 The cartilage intermediate-layer protein (CILP) is expressed by chondrocytes in the middle to deep zones of articular cartilage as a precursor protein. When cleaved during secretion, CILP has structural similarities with nucleotide pyrophosphohydrolase, although it lacks the catalytic site, and it may play a role in pyrophosphate metabolism and calcification.50,51 Asporin is related to decorin and biglycan and, similar to those other LRR proteins, may interact with and sequester growth factors such as TGF-β.52–54 YKL-40/HC-gp39, also known as chitinase 3-like protein 1, is found only in the superficial zone of normal cartilage and stimulates proliferation of chondrocytes and synovial cells.55 Chitinase 3-like protein 1 is induced by inflammatory cytokines and may function as a feedback regulator because it inhibits cytokine-induced cellular responses.56,57 Synthesis or release of these proteins or fragments is often increased in cartilage that is undergoing repair or remodeling, and they have been investigated as markers of cartilage damage in arthritis.31,32 A related member of the chitinase family, YKL-39, may be a more specific serum marker as a cartilage-derived autoantigen.58,59
Morphology, Classification, And Normal Function Of Chondrocytes
Morphology
The characteristic feature of the chondrocyte embedded in cartilage matrix is its rounded or polygonal morphology. The exception occurs at tissue boundaries, such as the articular surfaces of joints, where chondrocytes may be flattened or discoid. Intracellular features, including a rough endoplasmic reticulum, a juxtanuclear Golgi apparatus, and deposition of glycogen, are characteristic of a synthetically active cell. Stockwell and Meachim60 calculated that the cell density of full-thickness, human, adult, femoral condyle cartilage is maintained at 14.5 (±3.0) × 103 cells/mm2 from age 20 to 30 years. Because senescence of chondrocytes is known to occur with aging, it is logical to suppose that dead chondrocytes are replaced by mitosis. Mitotic figures are not observed, however, in normal adult articular cartilage.
The morphology, density, and synthetic activity of an adult chondrocyte vary according to its position within the different zones of articular cartilage.61–63 In the region of highest cell density, the superficial zone, cells are flattened and are oriented parallel to the surface, along with the collagen fibers. Chondrocytes within the middle zone appear larger and more rounded and display a random distribution within the matrix, where the collagen fibers also are more randomly arranged. Chondrocytes in the deeper zones form columns that, along with the collagen fibers, are oriented perpendicular to the cartilage surface. Chondrocytes may exhibit different behaviors depending on their position within the different layers, and these zonal differences in synthetic properties may persist in primary chondrocyte cultures.61,62 Chondrocyte volume in situ increases from the superficial through the deep zones and with the degree of cartilage degeneration.6 A study using confocal scanning laser microscopy of live, unfixed cartilage has revealed fine cytoplasmic processes extending from the cell bodies of 40% of chondrocytes.64 These processes are proposed to permit interactions among chondrocytes and the cartilage matrix at near and remote sites. They are distinct from the cilia, which are observed by electron microscopy65 but not by confocal scanning laser microscopy.64
Classification: Cell Origin and Differentiation
Chondrocyte arises in the embryo from mesenchymal origin during chondrogenesis, which is the earliest phase of skeletal development involving mesenchymal cell recruitment, migration, and condensation and differentiation of mesenchymal chondroprogenitor cells.66 As described in detail in Chapter 1, chondrogenesis results in the formation of cartilage anlagen, or templates, at sites where skeletal elements form. This process is controlled by cell-cell and cell-matrix interactions and by growth and differentiation factors that initiate or suppress cellular signaling pathways and transcription of specific genes in a temporospatial manner.
Vertebrate limb development is controlled by interacting patterning systems involving fibroblast growth factor (FGF), hedgehog, bone morphogenetic protein (BMP), and Wnt pathways. Wnt signaling, via the canonical β-catenin pathway and activation of TCF/Lef transcription factors, functions in a cell-autonomous manner to induce osteoblast differentiation and suppress chondrocyte differentiation in early chondroprogenitors.67 During chondrogenesis, Wnt/β-catenin acts at two stages: at low levels to promote chondroprogenitor differentiation, and later at high levels to promote chondrocyte hypertrophic differentiation and subsequent endochondral ossification.68,69 The transcription factor, Sry-type high-mobility group box 9 (Sox9), is an early marker of the differentiating chondrocyte that is required for the onset of expression of type II collagen, aggrecan, and other cartilage-specific matrix proteins, such as type IX collagen.70 Two other members of the SOX family, L-Sox5 and Sox6, are not present in early mesenchymal condensations but are required during overt chondrocyte differentiation, forming heterodimers that induce transcription more efficiently than Sox9 by itself.70 Expression of SOX proteins depends on signaling through mammalian homologs of Drosophila mothers against decapentaplegic (SMADs), which are functionally redundant and active in differentiating chondrocytes.71 A long form of c-Maf interacts with Sox9 at early stages to upregulate COL2A1 gene expression,72 whereas C/EBPβ and C/EBPδ and AP-2α may inhibit chondrocyte differentiation by blocking transcription of COL2A1, aggrecan (ACAN), and other cartilage-specific genes through direct or indirect mechanisms.73–75
In the embryonic or postnatal epiphyseal growth plates, upregulation of molecules that promote matrix remodeling and angiogenesis facilitates endochondral ossification, whereby bone replaces the calcified cartilaginous matrix in the hypertrophic zone (see Chapter 1). Differentiated chondrocytes that remain in the reserve, or resting, zone become the cartilage elements in articular joints, or they can proliferate and undergo the complex process of terminal differentiation to hypertrophy marked by type X collagen. Indian hedgehog and parathyroid hormone–related protein transiently induce proliferation and repress differentiation, determining the number of cells that enter the hypertrophic maturation pathway.76 The runt domain transcription factor, Runx2 (also known as core binding factor or Cbfa1), serves as a positive regulatory factor in chondrocyte maturation to the hypertrophic phenotype and subsequent osteogenesis.77 Runx2 is expressed in the adjacent perichondrium and in prehypertrophic chondrocytes, but less in late hypertrophic chondrocytes, and is required for expression of type X collagen and other markers of terminal differentiation.78
Numerous other transcription factors positively or negatively regulate chondrocyte terminal differentiation by controlling the expression or activity of Runx2.79 BMP-induced Smad 1 and interactions between Smad 1 and Runx2 are required for the induction of chondrocyte hypertrophy. Because no SMAD site is present on the Runx2 promoter, it has been proposed that homeodomain proteins such as Dlx3 may activate Runx2 signaling in response to BMP-2 during endochondral ossification, whereas Dlx5, Dlx6, and Msx2 are known to inhibit Runx2-mediated activation of genes such as osteocalcin at later stages.80 The homeodomain protein Nkx3.2, which is an early BMP-induced signal required at the onset of chondrogenesis, is a direct transcriptional repressor of Runx2 promoter activity.81 The bHLH factor Twist transiently inhibits Runx2 function and prevents premature osteoblast differentiation,82 whereas cooperation of the Groucho homolog Grg5 or the leucine zipper protein ATF4 with Runx2 promotes chondrocyte maturation.83 Histone deacetylase 4 (HDAC4), which is expressed later in prehypertrophic chondrocytes, prevents premature chondrocyte hypertrophy by interacting with Runx2 and inhibiting its activity.84 The hypoxia-inducible factor (HIF)-1α is required for chondrocyte survival during hypertrophic differentiation, owing partially to its regulation of vascular endothelial cell growth factor (VEGF) expression.85
The leucine zipper proteins Fra2, ATF2, and c-Maf are required for gene expression during late-stage hypertrophy (see for review, Goldring and Sandell75). One major function of the chondrocyte is growth of the skeleton through increased cell proliferation, production of ECM, and cell volume through hypertrophy. After cessation of growth, the resting chondrocyte remains as part of the supporting structures in articular, tracheal, and nasal cartilages, indicating that the fate of a chondrocyte depends on origin and location (Figure 3-3).
Normal Function of the Adult Articular Chondrocyte
The mature articular chondrocyte embedded in its ECM is a resting cell with no detectable mitotic activity and a low rate of synthetic activity. Because articular cartilage is not vascularized, the chondrocyte must rely on diffusion from the articular surface or subchondral bone for exchange of nutrients and metabolites. Chondrocytes maintain active membrane transport systems for exchange of cations, including Na+, K+, Ca2+, and H+, whose intracellular concentrations fluctuate with load and changes in the composition of the cartilage matrix.86 The chondrocyte cytoskeleton is composed of actin, tubulin, and vimentin filaments, and the composition of these filament systems varies in the different cartilage zones.87,88
Chondrocyte metabolism operates at low oxygen tension within the cartilage matrix, ranging from 10% at the surface to less than 1% in the deep zone. The consumption of oxygen by cartilage on a per-cell basis is only 2% to 5% of that in liver or kidney, although the amounts of lactate produced are comparable. Chondrocytes do not normally contain abundant mitochondria, energy metabolism depends strongly on the glucose supply, and the energy requirements may be modulated by mechanical stress.89 Glucose serves as the major energy source for chondrocytes and as an essential precursor for glycosaminoglycan synthesis.90 Facilitated glucose transport in chondrocytes is mediated by several distinct glucose transporter proteins (GLUTs) that may be constitutively expressed (GLUT3 and GLUT8) or cytokine inducible (GLUT1 and GLUT6).91,92 A proteomic study of chondrocytes identified 93 different intracellular proteins known to be involved in cell organization (26%), energy (16%), protein fate (14%), metabolism (12%), and cell stress (12%).93 The relative expression of these proteins may determine the capacity of chondrocytes to survive in cartilage matrix and to modulate metabolic activity in response to environmental changes.
When cultured in a range of oxygen tensions between severe hypoxia (0.1% oxygen) and normoxia (21% oxygen), chondrocytes adapt to low oxygen tension by upregulating HIF-1α. Hypoxia via HIF-1α can stimulate chondrocytes to express GLUTs92 and angiogenic factors such as VEGF94,95 and numerous genes associated with cartilage anabolism and chondrocyte differentiation, including Sox9, TGF-β, and connective tissue growth factor.96,97 In the growth plate, hypoxia and HIF-1α are associated with type II collagen production.98 HIF-1α is expressed in normal and OA articular cartilage, where it maintains tonic activity during physiologic hypoxia in the deeper layers associated with increased proteoglycan synthesis.99 It is not completely degraded, however, as it is in other tissues, when normoxic conditions are applied.100 Long-term systemic hypoxia (13%) may downregulate collagen and aggrecan gene expression in articular cartilage,101 whereas hyperoxia (55% oxygen) may increase the breakdown of cartilage collagens in articular cartilage in the presence of vascularized rheumatoid synovium.102 By modulating the intracellular expression of survival factors such as HIF-1α, chondrocytes have a high capacity to survive in the avascular cartilage matrix and to respond to environmental changes. Findings that catabolic stress and inflammatory cytokines upregulate HIF-1α suggest that it may serve as a survival factor in OA cartilage.99,103,104 More recent studies indicate that another hypoxia-inducible factor, HIF-2α, can become activated by proinflammatory cytokines and stress in the avascular, hypoxic environment of articular cartilage.105–107
The chondrocyte maintains a steady-state metabolism secondary to equilibrium between anabolic processes and catabolic processes that results in the normal turnover of matrix molecules. In normal adult articular cartilage, the turnover of matrix components is low. The turnover of collagen has been estimated to occur with a half-life greater than 100 years.108,109 In contrast, glycosaminoglycan constituents on the aggrecan core protein are more readily replaced; the half-life of aggrecan subfractions has been estimated to range from 3 to 24 years.110 Other cartilage ECM components, including biglycan, decorin, COMP, tenascins, and matrilins, incorporated previously into the matrix during development also may be synthesized by chondrocytes under low-turnover conditions. Regional differences in the remodeling activities of chondrocytes have been noted, however, and matrix turnover may be more rapid in the immediate pericellular zones.111 The metabolic potential of these cells is indicated by their capacity to proliferate in culture and to synthesize matrix proteins after enzymatic release from the cartilage of even elderly individuals.
The complex composition of the articular cartilage matrix is more difficult for the chondrocyte to replicate if severe damage to the collagen network occurs. During initial stages of OA, chondrocytes in vivo respond to structural changes in the surrounding cartilage matrix by increasing cell proliferation and synthesis of matrix proteins, proteinases, and anabolic and catabolic factors. The aberrant behavior of OA chondrocytes is reflected in the appearance of fibrillations; matrix depletion; cell clusters; and changes in quantity, distribution, or composition of matrix proteins.112,113 Evidence of phenotypic modulation is reflected in increases in collagens I and III114 and the appearance of the hypertrophic chondrocyte marker, type X collagen, and other chondrocyte differentiation genes, suggesting recapitulation of a developmental program.38,115 However, evidence reveals compensatory increases in type II collagen synthesis in deeper regions of the articular cartilage.112
Genomic and proteomic analyses of global gene expression in cartilage have confirmed increased COL2A1 mRNA levels in early OA cartilage.116–118 Increased levels of factors such as BMP-2 and inhibin βA/activin, members of the TGF-β superfamily,117,119,120 and prostaglandins121 suggest the presence of anabolic responses. Nevertheless, Aigner and co-workers118 have shown that COL2A1 expression is suppressed in upper zones of OA cartilage with progressing matrix destruction, whereas global COL2A1 gene expression is increased in late-stage OA cartilage compared with normal and early degenerative cartilage. The capacity of the adult articular chondrocyte to regenerate the normal cartilage matrix architecture is limited; the damage becomes irreversible, unless the destructive process is interrupted.
Culture Models For Studying Chondrocyte Metabolism
Primary cultures of articular chondrocytes isolated from various animal and human sources have served as useful models for studying the mechanisms controlling responses to growth factors and cytokines.122–125 A challenge to researchers in cartilage biology is the maintenance of chondrocyte morphology and cartilage-specific gene expression during in vitro studies. In confluent primary monolayer cultures, chondrocytes maintain a rounded, polygonal morphology (Figure 3-4), but progressive loss of cartilage phenotype is seen with passage of time and after subculture. High-density cultures maintain the gene expression and synthesis of cartilage-specific matrix proteins until they are subcultured, although gene expression of type II collagen is generally more labile than that of aggrecan. During this loss of phenotype or dedifferentiation, chondrocytes lose the rounded, polygonal morphology and express some, but not all, characteristics of the fibroblast phenotype, such as type I collagen. It is possible to expand cultures through a limited number of subcultures and to “redifferentiate” the cells in three-dimensional culture systems, in which chondrocytes regain morphology; cessation of proliferation is associated with increased expression of cartilage-specific matrix proteins. Alternatively, explant cultures of articular cartilage in which chondrocytes remain encased within their own ECM have been used as in vitro models to study cartilage biochemistry and metabolism, as described in the following section.
< div class='tao-gold-member'>
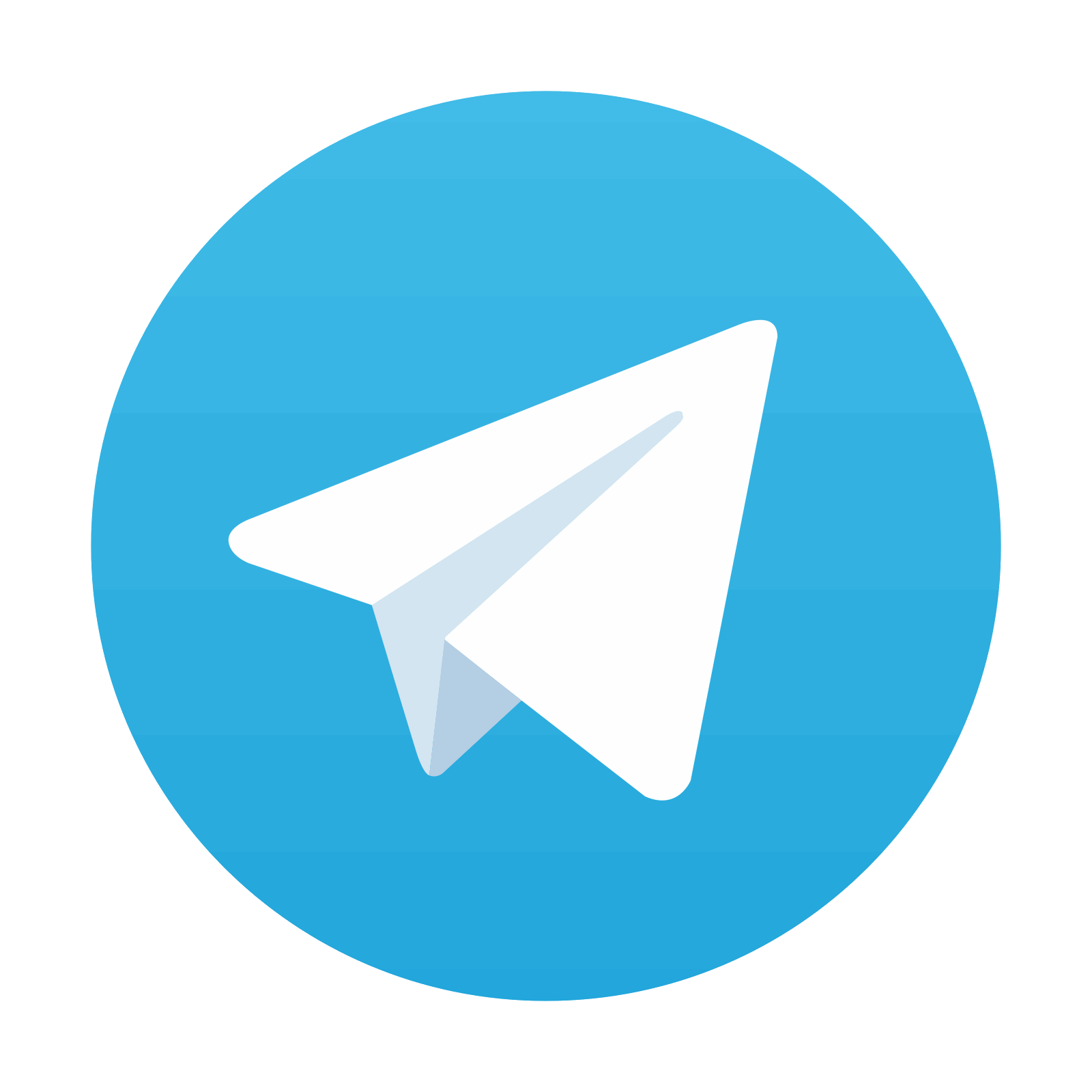
Stay updated, free articles. Join our Telegram channel

Full access? Get Clinical Tree
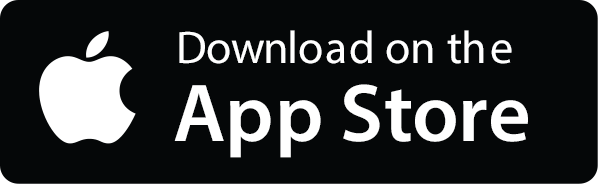
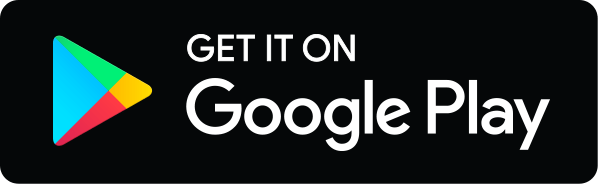