8
Cardiovascular System
Bryan W. Smith and Joseph Armen
At the completion of this chapter the reader should be able to do the following:
1. Understand the anatomy and physiology of the cardiovascular system
2. Understand cardiovascular adaptations to exercise
3. Identify various cardiac arrhythmias
4. Identify signs and symptoms of cardiovascular abnormalities
5. Know when to refer an athlete to a physician for further cardiovascular evaluation
Overview of Anatomy and Physiology
On the right side of the heart, the pulmonary valve is connected to the pulmonary artery, whereas on the left side, the aortic valve controls flow to the aorta (Figure 8-1). Blood flow returning to the heart from the body enters the corresponding atrium: pulmonic blood into the left atrium via the pulmonary vein and systemic blood into the right atrium via the superior and inferior vena cava (Figure 8-2).
Understanding how the cardiovascular system responds to exercise requires an understanding of basic cardiovascular physiology at rest. The right and left sides of the heart work together, pumping 5 to 6 L of blood per minute at rest (i.e., cardiac output). The heart pumps in a rhythmic cycle. Both ventricles contract during systole and relax during diastole. During the relaxed stage, they are passively filled with blood by atrial contraction. Pressures are rising and falling during this cardiac cycle, which permits the heart valves to open and close (see Figure 8-2).
Cardiac muscle is unique because it is able to contract within itself and operates on a serial electrical system, free from stimuli external to the heart. An electrical impulse begins at the sinoatrial (SA) node within the walls of the upper right atrium. This impulse travels through both atria to the atrioventricular (AV) node in the atrial septum. After a brief delay, the electrical impulse is promulgated to the bundle of His along the ventricular septum to the Purkinje fibers through the inferior and lateral ventricles1 (Figure 8-3). An electrocardiogram (ECG) records this electrical activity as two phenomena: depolarization (the spread of electricity through the cardiac muscle) and repolarization (the return of the stimulated heart to rest) (Box 8-1).1
As the ventricles relax, the pressure drops and allows the mitral and tricuspid valves to open. The rush of blood into the ventricles can cause a sound in children and young adults that is called S3. There is a fourth heart sound, S4, that marks atrial contraction and immediately precedes S1 of the next cardiac cycle. In older adults, S3 and S4 can be pathological heart sounds. Other pathological sounds, such as snaps and clicks, may also be heard (Figure 8-4).
High blood pressure, or hypertension, is defined as either systolic or diastolic pressure at or above 140/90 mm Hg. Conversely, hypotension, or low blood pressure, is defined as either systolic or diastolic pressure at or below 90/60 mm Hg. Children have lower blood pressure than adults. In fact, a blood pressure of 120/80 in an 8-year-old child would suggest hypertension.1 During dehydration from illness or heat, a drop in blood pressure caused by the decreased plasma volume can occur. A fall in systolic blood pressure of 20 mm Hg or more when accompanied by symptoms such as lightheadedness or fainting is called orthostatic hypotension. When this happens, the patient’s blood pressure should be checked in the supine, sitting, and standing positions.
Blood volume is just one factor that influences blood pressure. Cardiac output, peripheral resistance, blood viscosity, and the elasticity of the large arteries can cause variations in systolic pressure, diastolic pressure, or both. Because of the potential variability in blood pressure, proper measurement is important. Making sure the patient is calm and relaxed, using the proper size cuff, supporting the patient’s arm, and keeping the blood pressure cuff level with the heart are all important points to remember when measuring blood pressure. Chapter 2 gives instructions on how to take blood pressure.
Cardiovascular Adaptations to Exercise
Review of Exercise Physiology
Exercise is usually defined in terms of metabolic characteristics: dynamic or aerobic exercise versus static or anaerobic exercise.2 Most exercise is a composite of both types. Endurance running and swimming are examples of dynamic exercise whereas sprint running and power weight lifting are examples of static exercise.
In immediate outcomes, dynamic exercise results in increased cardiac output. Both components of cardiac output, stroke volume and heart rate, are increased. Enhanced cardiac contractility and increased venous return to the heart increase stroke volume. Blood flow is redistributed to the heart and skeletal muscles at the expense of the viscera while remaining constant to the brain. Vascular resistance is decreased because of vasodilation in the skeletal muscle, but blood pressure does not decrease because of the increased cardiac output.2,3 Pulse pressure is widened during dynamic exercise. Maximal dynamic exercise results in a four- to sixfold increase in cardiac output, a threefold increase in heart rate, and a twofold increase in stroke volume.2
Heart rate and blood pressure increase in static exercise. The pressure increase can be dramatic, with systolic pressure exceeding 250 mm Hg.2 High blood pressure is required to maintain blood flow to exercising muscles whose vessels are being
occluded because of the intense muscle contraction. Stroke volume, ejection fraction, and systemic vascular resistance remain unchanged. The higher pressures result in a higher cardiac workload compared with dynamic exercise.
Over the long term, dynamic exercise training results in increased cardiac output. The maximal heart rate cannot change with training, so increased cardiac output is the result of increased stroke volume. The heart adapts to the dynamic work by increasing in size, called hypertrophy. With this hypertrophy comes ventricular cavity dilation caused by the chronic volume loading. The increased diastolic volume permits greater stroke volume for less work. These changes can occur in athletes across the life span, including master-level athletes.2
Blood pressure during ongoing dynamic exercise training in elite athletes has been commonly thought to decrease. This has not been supported in many research studies.2 Scientific evidence, however, supports lowered blood pressure in sedentary adults after engaging in dynamic exercise training.4
Long-term, static exercise training also causes cardiovascular adaptations. In untrained subjects, small decreases are found in heart rate and blood pressure.2 Heavy weight training has been commonly believed to cause hypertension, but this has not been shown in body builders.2 In individuals with hypertension, however, chronic heavy weight training is not recommended. Pressure overload from chronic resistance training can cause cardiac hypertrophy without the chamber enlargement seen with dynamic exercise. Septal and posterior left ventricular wall thickening may also be seen.
Athlete’s Heart
The term athlete’s heart refers to the physiological and morphological adaptations mentioned previously, which an athlete’s cardiovascular system may undergo as a result of ongoing exercise training.5 Some of these adaptations can be confused with pathological cardiac conditions. It is important to allow healthy individuals the privilege of sports participation; it is even more important to distinguish athlete’s heart from pathological disease and minimize the risk of sudden cardiac death.
Both long-term dynamic and static exercise training can result in cardiac hypertrophy; these changes can occur after just a few weeks of training. Because heart wall thickness can be quite variable, sometimes as thick as 16 mm,6 one way to evaluate whether the cardiac changes are pathological is to detrain the athlete. If the wall thickness shrinks, the previous hypertrophy was probably due to the benign effects of athlete’s heart. A hypertrophic ventricle that does not diminish in size with detraining indicates possible cardiac disease or an idiopathic anomaly.
Preparticipation Examination
As discussed in Chapter 1, the preparticipation examination (PPE) sheds light on any medical problems that may affect athletic participation. The American Academy of Family Physicians (AAFP) recommends an initial evaluation for first-time participation in school or college athletics, with annual follow-up questions in certain areas. One of the areas of concern on both the initial and subsequent annual evaluation is cardiac health.7 Figure 1-8 gives the recommended questions for PPEs, including those related to potential cardiac problems. These questions are designed to alert the physician to potential life-threatening anomalies related to the heart and especially sudden death events. Any athlete who complains of symptoms consistent with these questions should be referred to a physician before continuing activity, regardless of whether the athlete has already passed a PPE screening.
General Evaluation of the Cardiovascular System
specific areas of the chest (Figure 8-5) while the athlete is in one position (e.g., sitting) and then repeat the sequence of auscultation while the athlete is supine and again with the athlete lying in a lateral recumbent position (Figure 8-6). The five auscultatory areas are as follows1:
1. Aortic valve: second right intercostal space at right sternal border
2. Pulmonic valve: second left intercostal space at left sternal border
3. Second pulmonic valve: third intercostal space at left sternal border
4. Tricuspid valve: fourth intercostal space along lower left sternal border
Accurate cardiac auscultation takes time. The examiner pauses at each auscultatory area to completely hear and isolate the sounds of each valve opening and closing. This skill requires patience, practice, and a quiet area. The examiner listens for the normal rate and rhythm of the heart in each auscultatory area and then specifically listens for the sounds associated with systole (i.e., contraction of the ventricles) followed by diastole (i.e., relaxation of the ventricles). Diastole is a longer interval than systole.1 When auscultating in each area, the examiner should also make sure to listen for adventitious or extra sounds or noises. The website that accompanies this text includes both normal and abnormal cardiac sounds for instructional purposes. The examiner should be aware that if athletes are asked to hold their breath during expiration, S1 may be more predominant; holding their breath on inspiration will cause S2 to be more distinct.
The physical examination of the athlete can be variable and nonspecific. In many high-performance athletes, particularly those who practice endurance sports, an increase in parasympathetic tone may cause a resting bradycardia. Resting heart rates have been recorded as low as 25 beats/minute in elite endurance athletes. Resting blood pressure usually is not changed, but it may be lowered. Third and fourth heart sounds may be present and are of no clinical significance as an isolated finding. Palpation may reveal a left ventricular impulse that is displaced to the left and prolonged. Because of the cardiac hypertrophy, 3% to 50% of high-performance athletes may have a mild mid-systolic heart murmur.6 These benign flow murmurs are best heard in the supine position and often disappear on standing.
The ECG in an individual with athlete’s heart can mimic many pathological conditions. The increased vagal tone and resultant bradycardia seen in trained athletes are associated with a greater incidence of benign arrhythmias, such as premature atrial contraction (PAC) and premature ventricular contraction (PVC), than in the general population.5 Conduction blocks and junctional rhythms also are more common.8 Complete, or third-degree, atrioventricular (AV) blocks, however, are rare and need to be investigated for pathology. High voltage on the ECG is common and can skew determining hypertrophy. The most common change seen in the athletic heart is early repolarization of the ventricles. On the ECG, this is evidenced by characteristic ST- and T-wave changes.8
Pathological Conditions of the Cardiac System
This section reviews various pathological cardiac conditions that may be seen in athletes. Rare congenital cardiac conditions are beyond the scope of this chapter. The most recent recommendations for determining eligibility for competition by athletes with cardiovascular abnormalities were published from the 36th Bethesda Conference in April of 2005.9,10
Sudden Cardiac Death
Sudden cardiac death is a rare event in the young athlete. In U.S. society, athletes are viewed as physically invincible because of the incredible physical feats displayed in the athletic arena. Any time a tragedy of this proportion occurs, the public’s reaction is one of disbelief and medical knowledge is called into question. Studies that have tracked these deaths during sports used the National Federation of State High School Associations (NFHS) and National Collegiate Athletic Association (NCAA) governing organizations as well as state and federally funded research groups for data collection. Approximately 90% of the data collected involved male athletes, with an equal distribution between Caucasians and African-Americans.11,12 Sudden death cases in young women are rare, and the most common cause of sudden cardiac death was congenital cardiac disease.
The prevalence of sudden cardiac death in young athletes (those less than 35 yr old) is estimated to be between 1 and 3 in 100,000.11–13 This is much higher than statistics reported in a more general population of active individuals, which show 1 in 735,000 U.S. Air Force recruits experiencing sudden cardiac death over a 20-year period.14 In contrast, Thompson and colleagues found the incidence of sudden death in joggers ranging from 30 to 65 years of age to be 1 in 7620.15 These data indicate that active people are not immune to cardiovascular events that may result in death. The National Registry of Sudden Death in Athletes (see Web Resources), hosted by the Minneapolis Heart Institute Foundation, provides information about cardiovascular mortality in athletes. It not only provides epidemiological data, but has educational references as well.
Causes
The most common cause of sudden cardiac death in the young athlete is hypertrophic cardiomyopathy, which accounts for up to 50% of the cases. Other significant causes of sudden cardiac death in a young athlete are coronary artery anomalies, increased cardiac mass, aortic rupture, myocarditis, and aortic stenosis.11–16 Rare causes include dilated cardiomyopathy, atherosclerotic coronary artery disease, mitral valve prolapse, isolated arrhythmias such as long QT syndrome and Wolff-Parkinson-White syndrome, and arrhythmogenic right ventricular dysplasia (ARVD).11–13 In the Veneto region of Italy, researchers have found ARVD to be the most common cause of sudden cardiac death in the athlete.17,18 This research suggests that a specific population may have different genetic subtraits.
In the older athlete, coronary artery disease is by far the most common cause of sudden death. Rarely is sudden death in the older athlete caused by hypertrophic cardiomyopathy, mitral valve prolapse, or acquired valvular conditions.18
Traumatic sudden cardiac death has not captured as much attention because its epidemiology is more difficult to track. However, it is a growing problem that strikes without warning. In 2009, the U.S. Commotio Cordis Registry within the Minneapolis Heart Institute reported 200 cases of traumatic sudden cardiac death,19–21 and most of the cases involved youngsters with a mean age of 13 years. Ninety-five percent of the cases occurred in males.
Commotio cordis refers to trauma to the chest wall that interrupts the electrical impulse in the heart. If the cardiac rhythm is not promptly normalized, the individual dies. Typically the ribs or sternum is not broken, although some contusions may be found. Research has found that a chest blow occurring during the vulnerable phase of repolarization, just prior to the T-wave peak in the cardiac cycle, can induce ventricular fibrillation.22,23
Although children and teenagers with thin chest walls are most vulnerable to commotio cordis (Figure 8-7), deaths have been reported in adults. Sports such as baseball, hockey, lacrosse, and softball, which have hard projectiles that can strike the chest, have been associated with the greatest number of deaths. Commotio cordis also has occurred in sports such as soccer, football, and karate, in which the blow came from a soft projectile or a collision. It appears that the timing of the incident, rather than the degree of impact of the object, is the causative factor. Commotio cordis is the only significant cause of traumatic sudden cardiac death in athletes.
Prevention
The American Heart Association estimates that 100,000 deaths could be prevented each year with prompt defibrillation.24 If defibrillation is performed within 3 minutes, the likelihood of survival is high. For every minute of delay the chance of survival drops by as much as 10%.
The advent of the automated external defibrillator (AED) has provided greater public access to life-saving technology (Figure 8-8). These devices can be operated by trained laypeople and are increasingly affordable in all sectors. The AED is portable, rechargeable, simple to operate, and easy to maintain. The American Red Cross, American Heart Association, and National Safety Council offer AED certification courses in addition to CPR courses for the lay public. Athletic trainers are required to be certified in AED use and need to have ready access to an AED in order to provide rapid cardiac assessment and care to those in fibrillation. When working with adolescents, athletic trainers need to consult the team physicians to make sure AED usage is appropriate, because the standard AED currently is limited to children over 8 years of age or those who weigh more than 55 pounds.
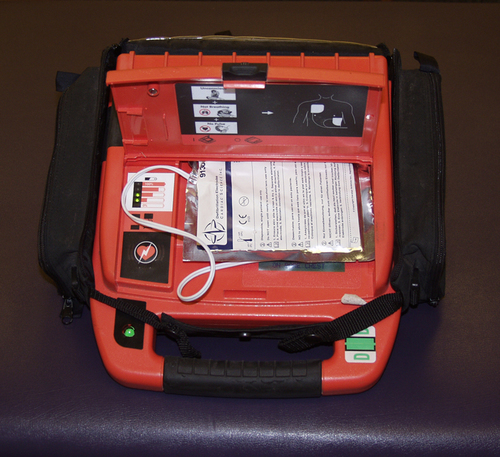
Hypertrophic Cardiomyopathy
Hypertrophic cardiomyopathy (HCM) is the leading cause of cardiac sudden death in athletes in the United States under the age of 35 years (Figure 8-9). The disorder is characterized by an abnormally hypertrophied but nondilated left ventricle in the absence of physiological conditions such as physical training or pathological conditions such as aortic stenosis or hypertension that would result in left ventricular hypertrophy.25 Numerous genetic mutations have been identified, and an autosomal dominant transmission of this disorder has been described.25
The prevalence of HCM is estimated at 1 in 500 in the general population, but no consensus exists about whether this disorder is more prevalent in males or females or in certain racial groups.26 The incidence of sudden death from HCM is two to three times higher in children and adolescents compared with adults.27
The walls of the left ventricle thicken in a variable pattern in HCM. As often as 28% of the time, physical obstruction of blood flow occurs during systole. Up to 80% of individuals with HCM have abnormally small coronary arteries that may cause myocardial ischemia.25 Cellular abnormalities include myofibrillar disorganization and death with resultant fibrotic scarring.
Although outflow obstruction can occur, the presence of left ventricular diastolic dysfunction is more common. Either outflow obstruction or diastolic dysfunction can impair exercise performance even in the least symptomatic individual.28,29 Both decreased wall distensibility and incomplete myocardial relaxation contribute to altered left ventricular filling,25 which leads to left atrial dilation and potential development of emboli. Regional myocardial ischemia likely occurs because of the abnormally small coronary arteries and inadequate capillary density.25 Adding random fibrosis of the cardiac musculature produces a combination of ischemia, fibrosis, and impaired vasodilator reserve that can lead to arrhythmia and sudden death.
Signs and Symptoms
Symptoms of HCM are fatigue, dyspnea, exertional angina, and syncope or near syncope. These symptoms may not correlate with the degree of ventricular hypertrophy or be predictive for sudden death.12,13 Physical examination can provide valuable information, but the findings are not consistent. On palpation, an increased left ventricular impulse may be felt. Pulses may be bifid in character and exhibit a brisk upstroke. On cardiac auscultation, a classic, harsh precordial ejection murmur may be heard at the left lower sternal border toward the apex. An example of this can be found on the accompanying website. The murmur increases with standing or Valsalva maneuver and diminishes with squatting; however, a murmur is not always present.
Referral and Diagnostic Tests
ventricular wall thickness (>15 mm) is the most helpful diagnostic parameter. The majority of male athletes and all female athletes have a ventricular wall thickness of 12 mm or less.6,30 Therefore the range from 13 to 15 mm may pose a diagnostic dilemma because it is possible to have physiological hypertrophy in this wall thickness range; however, this hypertrophy has been reported only in male cyclists and rowers.6 Another sign of HCM that can be seen on an echocardiogram is a ventricular septum/free wall thickness ratio greater than 1:3. Asymmetric wall thickening and decreased left ventricular diastolic cavity dimension (<45 mm) may also be present. In addition, the echo may reveal abnormal diastolic filling with decreased early filling and increased late filling and abnormal ultrasonic myocardial reflex activity in the athlete with HCM.31
Discontinuing athletic activities over a few weeks to 1 month for the athlete with these symptoms may distinguish whether the problem is HCM, or simply athlete’s heart.32,33 Hypertrophy of the ventricular wall will not resolve with detraining in an individual with HCM.
As a diagnostic tool for HCM, echocardiography is the “gold standard.” Its value as a screening tool, however, is limited by its relative expense. The cost to prevent one death from HCM by using echocardiography as a preparticipation screening tool would exceed $100 million.34 In the future, genetic markers may hold the greatest promise as effective screening tests, with 12 mutant genes and more than 400 specific mutations presently implicated in the pathogenesis of clinically diagnosed HCM.33
Prognosis and Implications for Participation
On the basis of the 36th Bethesda Conference recommendations, athletes with hypertrophic cardiomyopathy should be restricted from participation in all competitive sports with the possible exception of low-intensity (class IA) sports such as golf, bowling, and billiards (Table 8-1).35,36 Furthermore, the placement of an implantable cardioverter-defibrillator (ICD) in a patient with HCM does not change the competitive sports recommendations for this disease.
TABLE 8-1
Classification of Sports Based on Peak Static and Dynamic Components During Competition
Classification | |||
Classification | Low Dynamic (A) | Moderate Dynamic (B) | High Dynamic (C) |
Low static (I) | Billiards | Baseball∗ | Badminton |
Bowling | Softball∗ | Cross-country skiing (classic technique) | |
Cricket | Fencing | Tennis (singles) | |
Curling | Table tennis | Field hockey∗ | |
Golf | Volleyball | Orienteering | |
Racquetball/squash | |||
Race walking | |||
Distance running | |||
Soccer∗ | |||
Tennis | |||
Moderate static (II) | Archery | American football∗† | Basketball∗ |
Auto racing∗† | Field events (jumping) | Ice hockey∗ | |
Diving∗† | Figure skating∗ | Cross-country skiing (skating technique) | |
Equestrian∗† | Rodeo∗† | Lacrosse∗ | |
Motorcycling∗† | Sprint running | Mid-distance running | |
Surfing | Swimming† | ||
Synchronized swimming | Team handball | ||
Rugby∗ | |||
High static (III) | Bobsledding/luge∗† | Body building∗† | Boxing∗ |
Field events (throw) | Downhill skiing∗† | Canoeing/kayaking | |
Gymnastics∗† | Wrestling∗ | Cycling∗† | |
Karate/judo∗ | Decathlon | ||
Sailing | Rowing | ||
Rock climbing | Speed skating∗† | ||
Water skiing∗† | Triathlon∗† | ||
Weight lifting∗† | |||
Wind surfing∗† |
†Increased risk if syncope occurs.
From Mitchell JH, Haskell W, Snell P, et al: Task Force 8: classification of sports, Journal of the American College of Cardiology 45(8):1366, 2005.
Coronary Artery Abnormalities
Congenital coronary anomalies are a much less frequent cause of sudden death than HCM in athletes. They are characterized by either an aberrant (i.e., deviating or abnormal) coronary artery takeoff or the complete absence of a coronary artery. Most reports rank coronary anomalies as the second leading cause of sudden death in athletes,11–13 with the majority of events happening during or just after strenuous exercise.
Myocardial bridging refers to a coronary artery that is surrounded by myocardium for a portion of its course. This tunneling is seen in up to 25% of hearts at the time of autopsy after sudden death and usually involves the left anterior descending artery. Rarely does a congenital aberration result in clinical pathology, but the vascular compression from the ventricle during systole has been reported as an exercise-related cause of sudden death.37
Referral and Diagnostic Tests
Any athlete presenting with unexplained exertional chest pain or an exertional syncopal episode must be referred immediately to a physician. An echocardiogram may demonstrate the anomalous takeoff. However, cardioangiography is usually required for a conclusive diagnosis (Figure 8-10).
Treatment
At present no medical treatment exists that can permit continued athletic competition, although the individual may benefit from β-adrenergic or calcium-blocking agents. In myocardial bridging, surgical resection may resolve the ischemia and allow a safe return to play. Coronary stenting has been associated with restenosis and periprocedural complications in 50% of cases.39
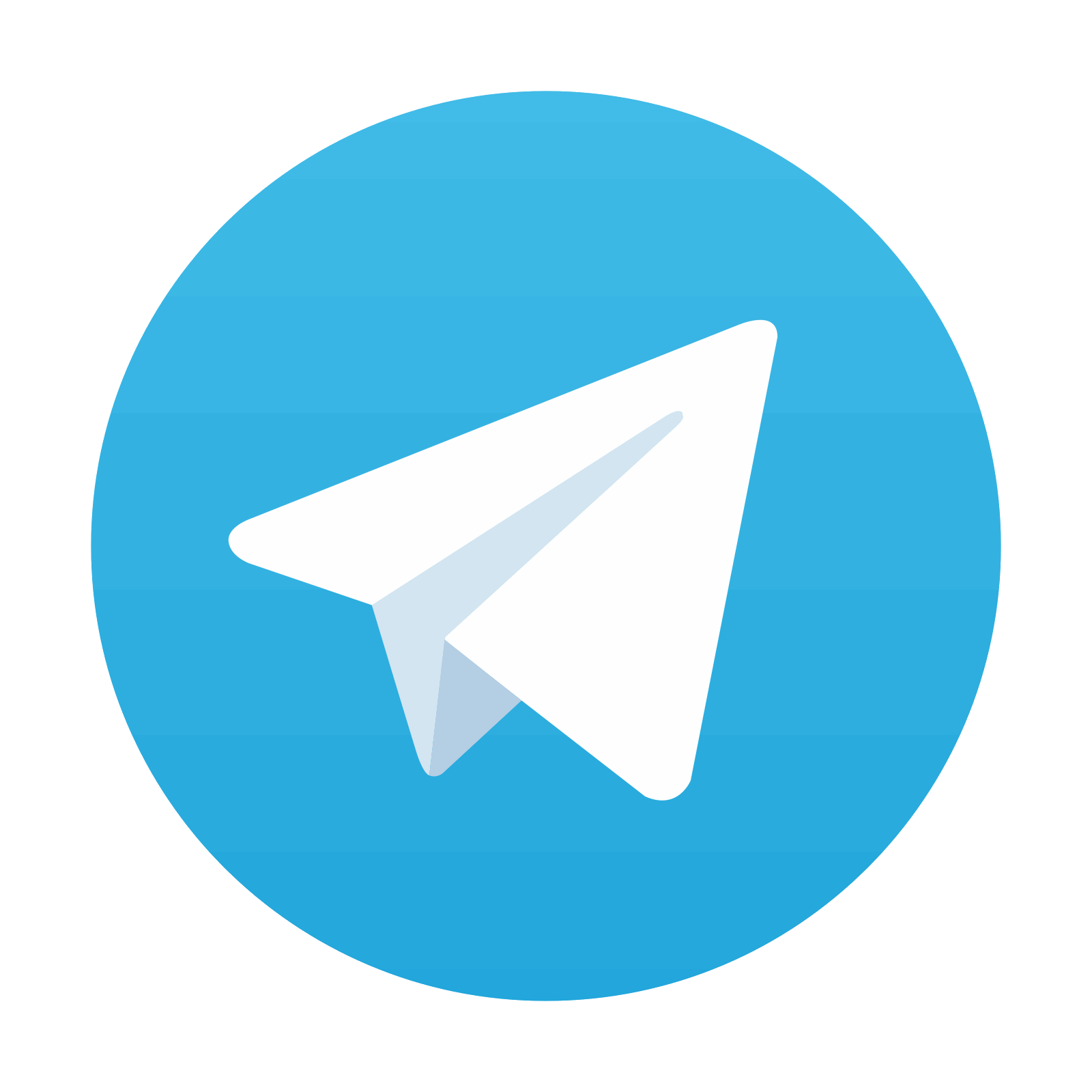
Stay updated, free articles. Join our Telegram channel

Full access? Get Clinical Tree
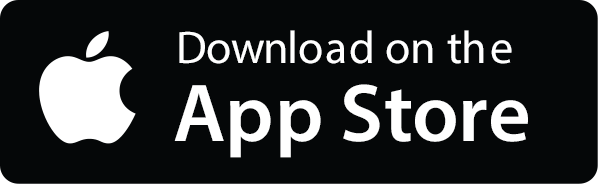
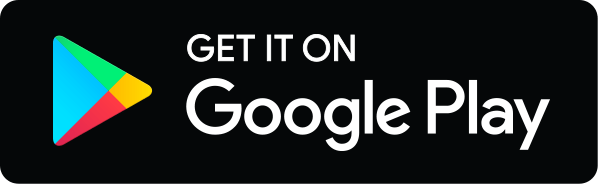