Fig. 1.1
A portrait of Sir Isaac Newton during his time as President of the Royal Society (Ref: www.parrswood.manchester.sch.uk)
From the Original Latin of Newton’s Principia
Lex I: Corpus omneperseverare in statusuoquiescendivelmovendiuniformiter in directum, nisi quatenus a viribusimpressiscogiturstatumillummutare.
Lex II: Mutationemmotusproportionalemesse vi motriciimpressae, et fierisecundumlineamrectam qua vis illaimprimitur.
Lex III: Actionicontrariam semper et æqualemessereactionem: sivecorporumduorumactiones in se mutuo semper esseæquales et in partescontrariasdirigi.
Translated to English
Law I: Every body persists in its state of being at rest or of moving uniformly straight forward, except insofar as it is compelled to change its state by force impressed.
Law II: The change of momentum of a body is proportional to the impulse impressed on the body and happens along the straight line on which that impulse is impressed.
Law III: To every action, there is always opposed an equal reaction, or the mutual actions of two bodies upon each other are always equal and directed to contrary parts.
In developing his laws of motion, Sir Isaac Newton credits Galileo Galilei for the first law. Initially, a Greek philosopher Aristotle observed and believed that for the body at rest to move in a straight line at a constant speed, an external force was necessary to maintain the constant speed. Centuries later, Galileo Galilei realized that an external force is necessary to change the velocity of a body, which is defined as acceleration, but no force was required to maintain its velocity. This tendency of objects to resist changes in motion was what Galileo called “inertia.” This was then refined by Newton who made it into “Newton’s first law,” also known as the “law of inertia.” Therefore, Newton’s first law is a restatement of the “law of inertia” which Galileo described. Although not widely publicized, Newton appropriately gave credit to Galileo for the first law.
For shoulder biomechanics, it is very important to have a good understanding of Newton’s laws of motion to understand shoulder function and the intricate biomechanical characteristics of each tissue structure and their complex interplay that occurs to provide stability and function.
1.2 Shoulder Biomechanics
1.2.1 Stability and Kinematics of Shoulder Motion
The shoulder provides the greatest range of motion of any joint in the body, but the trade-off is the propensity for instability. This unique function is essential at the shoulder to enable the hand to be placed in the multitude of positions required in everyday life. This is provided by the intricate and complex coordinated interplay of the active and passive stabilizers of the shoulder. Shoulder motion is comprised of many joints, primarily the glenohumeral and scapulothoracic joints. Abduction in the scapular plane is created by both joints in a movement termed “scapulohumeral rhythm.” Shoulder abduction in the scapular plane is described in a 2:1 ratio between glenohumeral and scapulothoracic motion [2, 3], with some variation in the first 30° of abduction (Fig. 1.2) [2, 4, 5]. The sternoclavicular and acromioclavicular joints move at the extremes of motion. Shoulder motion can be broken down into three planes of motion: abduction and adduction in the coronal plane, flexion and extension in the sagittal plane, and rotation about the long axis of the humerus. Arm abduction has an arc of motion of approximately 0–180°, flexion and extension is approximately 180°, and internal and external rotation is approximately 150°. In the glenohumeral joint, as in all diarthrodial joints, six degrees of freedom are present, three translational and three rotational. The three motions that best describe shoulder function are spinning, sliding, and rolling. Spinning occurs when the contact point on the glenoid remains the same while the humeral head contact point is changing. Sliding is pure translation of the humeral head on the articular surface of the glenoid. At the extremes of motion, and certainly in unstable joints, glenohumeral translations occur. In this circumstance, the contact point on the glenoid is moving, while that for the humerus remains the same. The third type of action, rolling, may also occur at the glenohumeral joint. Rolling is a combination of humeral head translation and spinning with respect to the glenoid, and the contact point changes on both the glenoid and the humeral head [6]. All three rolling motions may take place at the glenohumeral joint about all three orthogonal axes of the glenohumeral joint.
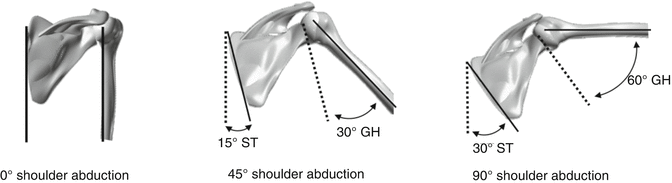
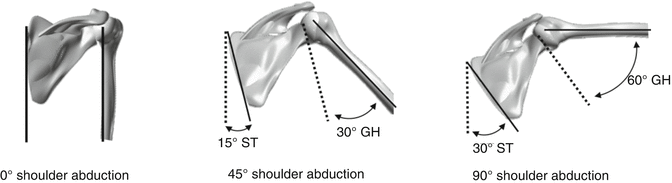
Fig. 1.2
Shoulder motion is comprised of many joints, primarily the glenohumeral (GH) and scapulothoracic joints (ST). Abduction in the scapular plane is created by both joints in a movement termed “scapulohumeral rhythm”
1.2.2 Passive Bony Stabilizers
The anatomic relationship between the humeral head and glenoid can be thought of in a relative ratio of the diameter of each, known as the glenohumeral index (Fig. 1.3). This glenohumeral index is calculated by the maximum diameter of the glenoid divided by the maximum diameter of the humeral head. It is reported to be approximately 0.75 in the sagittal plane and 0.6 in the transverse plane [7]. Glenohumeral stability is often characterized by the stability ratio, which is the force necessary to dislocate the humeral head from the glenoid divided by the compressive load [8, 9]. This stability ratio is dependent on the depth of the glenoid and increases with greater glenoid depth. The labrum contributes to this by deepening the concavity of the glenoid. It has been shown that this ratio decreases approximately 20 % if the labrum is removed and even further with chondrolabral defects [10]. Stability ratios have been shown to be higher in the superior-inferior versus anterior-posterior plane and with humeral adduction compared to abduction. In this same report, the labrum was noted to contribute only 10 % to the stability [11].
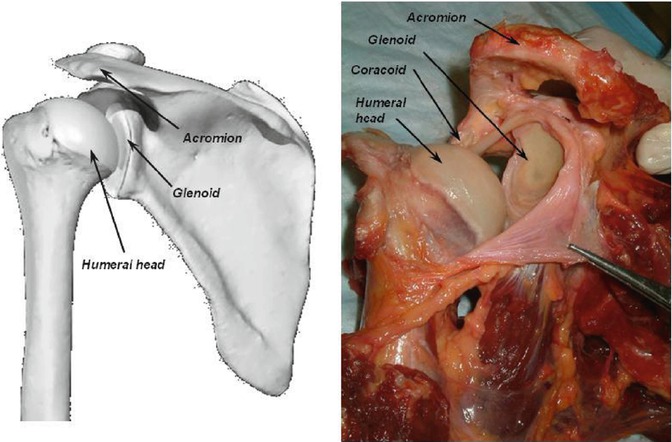
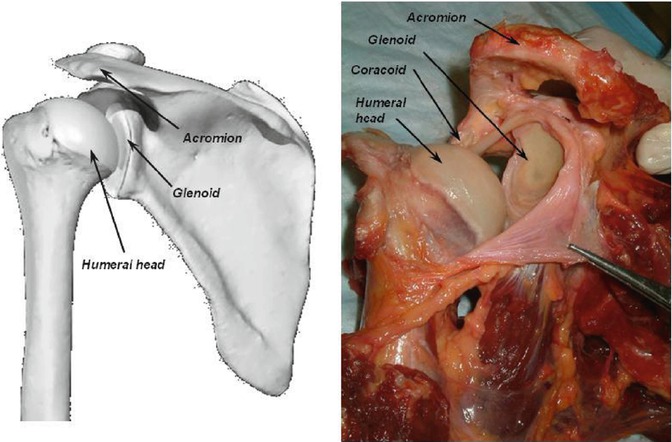
Fig. 1.3
Images showing the bony stability of the glenohumeral joint
1.2.2.1 Humeral Head
Only 25–30 % of the humeral head is covered by the glenoid at any given anatomic position. Humeral position has been shown to affect contact area between the humeral head and glenoid. With increasing abduction, the contact area as well as the congruity between the glenoid and humeral head improves [12]. This is due to an articular surface mismatch in adduction. Rotation affects contact area with the contact point of the humeral articular surface moving forward and inferior during internal rotation and posterior and inferior in external rotation [7]. With elevation, the contact moves superiorly on the humeral surface [13] and contact area of the glenoid shifts posteriorly. The humeral contact in elevation moves from inferior to superocentral-posterior region with the maximum contact areas occurring at 120° of elevation [14]. Ultimately the stability provided by the humeral head lies in its ability to achieve congruence with the glenoid which is maximized in elevation, a common functional position, and a position that occurs in the act of throwing.
1.2.2.2 Glenoid
In the coronal plane, the glenoid is inclined superiorly with a mean inclination reported to be 4.2° (range −7 to 15.8°). In the sagittal plane, the glenoid is retroverted 1.2 ± 3.5° (range 9.5° anteversion to 10.5° retroversion is measured from the axial plane) [15]. The little stability the glenoid provides comes from the depth, version, and inclination. Glenoid version varies greatly with excessive retroversion causing posterior instability [16]. Shoulder arthroplasty studies have shown that when the glenoid component is retroverted, a common occurrence in osteoarthritis, significant eccentric forces are placed on the glenoid component that could lead to loosening [17]. The exact effect and amount of excessive retroversion or anteversion of the glenoid on native shoulder stability are unclear.
The slight superior tilt of the glenoid articular surface is considered a factor in preventing inferior subluxation of the humerus when combined with the superior capsule and superior glenohumeral ligament [18]. A downward-facing glenoid is related to multidirectional instability. A biomechanical study by Itoi et al. demonstrated the relationship between scapular inclination and inferior stability [19]. In this study, as the scapulae were adducted to create a downward-facing glenoid, the shoulders dislocated inferiorly. As the scapulae were abducted, creating a more inclined glenoid, the humeri reduced. Combined with the superior capsuloligamentous structures, the mechanism of scapular inclination is that of a cam effect determined by the geometry of the glenoid and humerus. This was confirmed in a biomechanical study on posteroinferior glenoplasty where the glenoplasty procedure increased the slope from 0.55 ± 0.07 to 0.83 ± 0.12 and increased the posteroinferior stability ratio from 0.47 ± 0.10 to 0.81 ± 0.17 [20].
1.2.3 Passive Soft Tissue Stabilizers
The passive soft tissue stabilizers include the glenoid labrum and the glenohumeral ligaments (Fig. 1.4). These help limit glenohumeral joint rotation and translation, often in a position-dependent manner. The role of the soft tissue passive stabilizers and particularly the glenohumeral ligaments on shoulder function has been extensively studied. Of the soft tissue stabilizers, the middle glenohumeral ligament and the anterior fibers of the inferior glenohumeral ligament (IGHL) work together as barriers to dislocation at 45° glenohumeral abduction. The IGHL alone prevents anterior dislocation of the joint at 90° of abduction [21]. For anterior stability, the anterior-superior portion of the IGHL has been shown to be the primary capsular restraint [22]. In this section, we will discuss the anatomy and biomechanics of the soft tissue passive stabilizers.
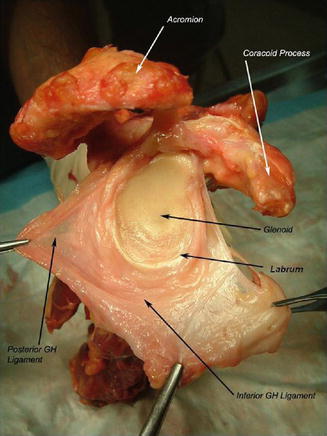
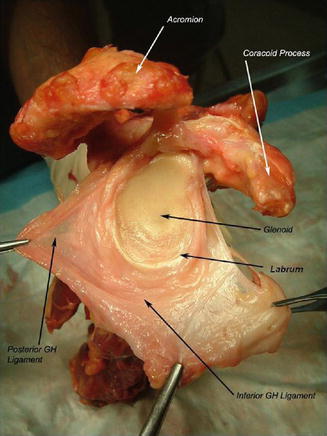
Fig. 1.4
Passive soft tissue stabilizers include the glenoid labrum and the glenohumeral ligaments. The middle glenohumeral ligament is not shown
1.2.3.1 Labrum
The labrum is a triangular rim of fibrocartilaginous tissue that surrounds the glenoid. It deepens the socket by an average of 9 mm in the superior-inferior plane and 5 mm in the anterior-posterior plane. This accounts for up to 50 % of the total glenoid socket depth. Removal of the labrum, as in a Bankart lesion, reduces the depth from 5.0 to 2.4 mm [23]. A cadaveric study in which the labrum was removed but the capsule remained intact demonstrated an increased laxity with labral resection in the adducted position [24]. The stability ratio decreases 20 % with resection of the labrum and further decreases with chondrolabral defects [10].
The superior labrum has a distinct anatomy from the remainder of the labrum, due to the long head of the biceps tendon insertion. The superior labrum functions as a passive stabilizer of the humeral head. In a cadaveric study, surgically created Type II SLAP tears resulted in a significant increase in total range of motion, external rotation, internal rotation, anterior-posterior translation, and inferior translation. After arthroscopic repair, these values were restored [25]. However, the glenohumeral kinematics were not significantly altered by a surgically created Type II SLAP tear [26].
1.2.3.2 Inferior Glenohumeral Ligament Complex (IGHLC)
The anterior IGHL is the most important stabilizer in 90° of abduction and external rotation [21]. Strain measurements confirmed this by showing the anterior band to be tight in abduction and external rotation with the posterior band tight in abduction and internal rotation [27]. The axillary pouch (6 o’clock position) was also found to be an important anterior stabilizer with the arm in abduction and external rotation [28]. Further, the anterior band of the IGHL has been shown to be the primary anterior stabilizer with the arm in abduction in the scapular plane [29]. It is important to note that IGHL plastic deformations exist in shoulders with pathologic recurrent instability. This has been shown to occur in a cyclic overloading study whereby repeated sub-failure strain resulted in an overuse injury to the IGHL [30]. However, the permanent stretching in the IGHL has been shown to be quite small (less than 1 mm), suggesting that only a slight plication is necessary to restore capsular anatomy after a primary instability injury [31, 32]. In another cadaveric study, a positive linear correlation between the length of the anterior band of the inferior glenohumeral ligament, external rotation of the humerus, and anterior translation was demonstrated [33]. For anterior instability resulting from capsular laxity, arthroscopic anterior-inferior plication of 10 mm has been found to be effective in reducing anterior translation and external rotation but altered the glenohumeral center of rotation posteriorly and inferiorly [34, 35].
The labrum-IGHL complex does not have any significant role in the joint reactive forces of the glenohumeral joint. In a cadaveric study, incision and resection of the anteroinferior capsule and labrum did not significantly affect the joint reactive forces demonstrating that the concavity compression effect seen in the shoulder is greatly affected by the dynamic stabilizers [36]. However, the IGHLC is a major contributor to the passive stabilizers of the glenohumeral joint, with its function dependent on arm position. Based on cadaveric studies, arthroscopic techniques have the potential to restore the normal passive stabilizing function of the capsule and labrum but significantly alter the path of glenohumeral joint articulation and potentially initiate subsequent glenohumeral osteoarthritis.
1.2.3.3 Superior and Middle Glenohumeral Ligaments
In conjunction with superior glenoid tilt, the SGHL acts to provide passive resistance to inferior subluxation [18, 37, 38]. Strain in the SGHL is maximal with the arm in adduction and external rotation. The MGHL is a major constraint to anterior humeral displacement and becomes taut in the abducted, externally rotated position [21]. With the arm in external rotation, strain in the MGHL was found to be highest at 0 and 45° of abduction but decreased in 90° of abduction [39]. Sectioning of the MGHL does allow increased excursion of the humeral head but does not typically result in instability, making this a contributor to anterior stability, but not the essential ligament that is damaged in anterior instability [37].
1.2.3.4 Rotator Interval
Shoulders with rotator interval lesions demonstrate inferior instability with the arm in internal rotation [40]. Sectioning of the rotator interval has been shown to create inferior and posterior instability while imbrication provides resistance to inferior and posterior translation [41]. In a more specific study, the coracohumeral ligament (CHL) has been shown to be a restraint to inferior translation but only in the position of external rotation. In internal and neutral rotation, the negative intra-articular pressure provided by an intact rotator interval capsule has been shown to provide superior-inferior stability [42]. Rotator interval closure involves imbrication of the capsule from the supraspinatus to the subscapularis and has been shown to improve translation in all directions for multidirectional instability [43]. More specifically, in 60° of glenohumeral abduction, a medial-lateral rotator interval closure restored range of motion to the intact state and was more effective in reducing posterior translation than a superior-inferior closure [44]. Additional studies have shown that a rotator interval closure reduced translation as well as abduction, flexion, and external rotation [45, 46]. However, when combining rotator interval closure with capsule plication in the treatment of multidirectional instability, a surgeon should evaluate patients individually, so as to avoid over-tightening when performing both of these procedures [47].
1.2.3.5 Coracoacromial Ligament
The coracoacromial (CA) ligament spans from the anterolateral acromion to the coracoid. It consists of an anterolateral band and a posteromedial band with the anterolateral band covering the entire anterior acromial undersurface. This ligament has been shown to act as a humeral head stabilizer and suggests that it plays a role in the static restraint of the glenohumeral joint. It provides a suspensory function and may restrain anterior and inferior translations through an interaction with the coracohumeral ligament [48]. Its function in rotator cuff pathology however is unclear. Cyclic loading of the CA ligament demonstrated a greater drop in peak stress in rotator cuff tear shoulders than in normal shoulders, whereas the stress relaxation response was not different. This was attributed to ultrastructural changes within the ligament as a result of an altered loading environment. However, it remains unknown whether this occurs as a result of a rotator cuff tear or if it contributes to the pathogenesis of cuff disease [49]. Currently there is debate regarding management of the CA ligament in the setting of rotator cuff pathology and further studies are warranted.
1.2.4 Active Stabilizers
The active stabilizers of the shoulder are muscle-tendon complexes that provide stability and function to the shoulder. These include the rotator cuff, biceps, deltoid, pectoralis major, and latissimus dorsi. The effect of the shoulder muscles on shoulder stability has been recognized since 1884 [50]. These shoulder muscles are very important to normal shoulder function [51, 52]. These muscles generate a joint compressive force, which in combination with the passive restraints maintain joint stability [8, 51]. Muscle forces are probably most important in the midranges of shoulder motion when the capsule and glenohumeral ligaments are thought to be lax. However, shoulder muscles are also active when the shoulder is abducted and fully externally rotated [53, 54]. This interplay of active and passive stabilizers is critical for glenohumeral joint stability. Strengthening of the shoulder muscles enhances joint stability and function where large forces are generated in the shoulder for movement [55]. In the normal shoulder, this contributes meaningfully to joint stability through the application of a compression force. This is the component of the glenohumeral joint force that acts perpendicular to the glenoid fossa such that the concave humeral head is compressed into the glenoid fossa. Coined “concavity compression,” this action was initially reported as being important in maintaining joint stability at the midranges of shoulder elevation when the passive restraints are lax [8]. The shoulder muscles that are active in elevation of the arm include the rotator cuff muscles. In addition to the rotator cuff muscles, any muscle that crosses the glenohumeral joint can contribute to concavity compression [56–60]. When this complex and intricate interplay between the shoulder muscles is altered, the force environment in the shoulder will also be altered, according to functional demands. As a result, the abnormal force environment in the shoulder may also initiate a series of subsequent shoulder pathologies. In this section, we will discuss the stability provided by the active stabilizers and the functions of the rotator cuff and surrounding musculature (Fig. 1.5).
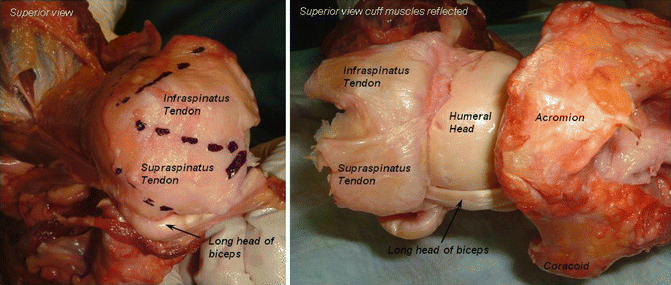
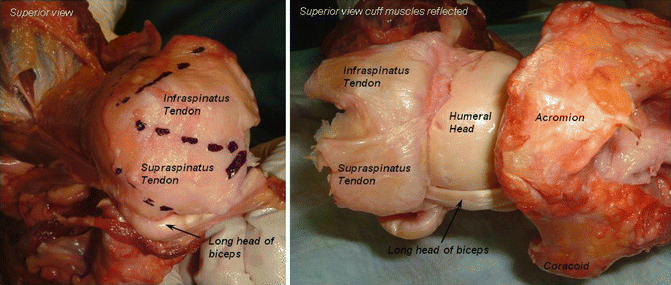
Fig. 1.5
The rotator cuff muscles provide significant stability to the shoulder joint, almost hugging the joint to the glenoid
1.2.4.1 Rotator Cuff
The rotator cuff provides stability through concavity compression and barrier effects [61–63]. The contribution of the active stabilizers was thought to contribute more than the passive stabilizers [64]. However, one study has shown an equal contribution to anterior stability with the cuff playing a more important role posteriorly [65]. The subscapularis functions to adduct and internally rotate the humerus. The supraspinatus functions as a shoulder abductor, initiating the first 30° of forward elevation and assisting the deltoid in the first 90° of abduction. The supraspinatus is not a single fusiform muscle and tendon, but rather a more complex structure with distinct anterior and posterior subregions [66–69]. The tendon associated with the anterior subregion is thicker and more tubular, while that of the posterior subregion is thinner and flat (Fig. 1.6). The anterior tendon also extends further medially from its insertion on the greater tuberosity and branches into a fibrous framework, while the posterior tendon does not have this fibrous organization. The anterior and posterior subregions also have different muscle physiological cross-section areas (PCSAs) despite having similar tendon cross-sectional areas [67]. The function of the supraspinatus is complex. Besides initiating shoulder abduction and stabilizing the glenohumeral joint, it contributes to humeral rotation. Its role in humeral rotation, however, is dependent on the initial position of the humerus [70]. In the scapular plane, the anterior subregion of the supraspinatus acts as both an internal and external rotator, depending on the initial humeral position, while the posterior subregion acts only as an external rotator. The infraspinatus and teres minor work as humeral external rotators and are most active with the humerus adducted [70].
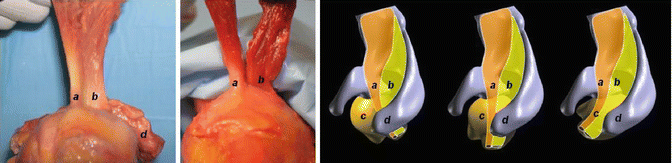
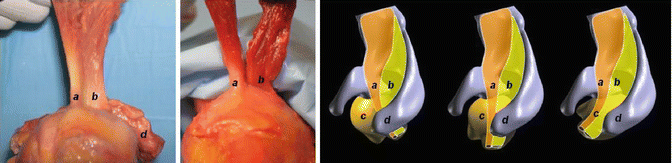
Fig. 1.6
Photographs and schematic drawing demonstrating the supraspinatus subregional footprint orientation at different humeral rotation positions (a anterior supraspinatus, b posterior supraspinatus, c humeral head, d acromion) [70]
1.2.4.2 Long Head of Biceps Tendon (LHBT)
The long head of the biceps tendon’s function has been difficult to establish, mostly because it spans the glenohumeral and ulnohumeral joints. It has been shown to be a depressor of the humeral head as well as aid in rotational and translational stability [71, 72]. This was demonstrated by sequential loading of the LHBT in a human cadaveric model where significant decreases in external rotation, internal rotation, and total range of motion were observed. Glenohumeral translation in all planes was significantly decreased as well. The humeral head shifted posteriorly with biceps loading at maximum internal rotation, 30° and 60° of external rotation. At maximum external rotation, the biceps loading shifted the humeral head anterior. These findings support the belief that the LHBT may act to center the humeral head on the glenoid during extremes of motion [73]. The biceps has also been shown to be as efficient as the supraspinatus, infraspinatus, and teres minor as a stabilizer, because the biceps becomes more important than the cuff muscles in an unstable shoulder [74]. In the setting of rotator cuff tears, active contraction of the biceps prevents superior migration of the humeral head, almost normalizing the kinematics of the glenohumeral joint [75]. In a cadaveric study, cuff-deficient shoulders had more hypertrophy of the long head of the biceps tendon, while muscle hypertrophy did not occur. This hypertrophy of the tendon indicates a local pathology such as impingement or secondary stabilization, rather than compensatory overuse and hypertrophy of the muscle [76].
1.2.4.3 Deltoid
The deltoid functions primarily as a shoulder abductor and forward flexor. An EMG study showed that the action of the deltoid was highly differentiated in its five different regions with a component of dynamic stability in the scapular plane [77, 78]. Anterior stabilization by the deltoid has been shown in the abducted, externally rotated position [79]. The large and powerful nature of the deltoid has a strong influence on stability and normal shoulder motion.
1.2.4.4 Force Couples
To understand the combined function of the rotator cuff, one must understand the concept of force couples. A force couple is defined as two forces that act on an object to cause rotation. In order to reach a state of equilibrium, the sum of forces on an object must be equal in magnitude and opposite in direction. Two major force couples act synergistically on the glenohumeral joint: one in the coronal plane and the other in the transverse plane.
The force couple acting in the coronal plane is comprised of the moments produced by the deltoid and inferior rotator cuff (infraspinatus, teres minor, and subscapularis) [2]. The deltoid moment lies above the center of rotation, while the inferior cuff moment acts below the center of rotation, parallel to the lateral border of the scapula. This force couple is important in producing stable glenohumeral abduction. Also important contributors, the pectoralis major and latissimus dorsi are included in this force couple. The depressor moment produced by the inferior rotator cuff may be too weak to counterbalance the strong deltoid moment. However, the pectoralis major and latissimus dorsi have similar depressor moments as the deltoid [80] and therefore are thought to work along with the inferior rotator cuff in the coronal plane force couple [81]. Recent biomechanical studies have replicated the moments produced by the pectoralis major and latissimus dorsi in order to create a more anatomic shoulder construct [80–86].
The transverse force couple is comprised of moments produced by the subscapularis anteriorly and infraspinatus and teres minor posteriorly. Inability to maintain a balanced transverse force couple can lead to anterior or posterior translation of the humeral head. In the setting of a massive rotator cuff tear involving the infraspinatus and teres minor (or posterior moment), the larger moment produced by the subscapularis can lead to anterior translation of the humeral head. This uncoupling between forces leads to an unstable fulcrum for glenohumeral motion.
When the force couples are disrupted, force imbalance occurs in the shoulder affecting the stability and the kinematics of the shoulder. This was demonstrated in a cadaveric study by simulating a weakened subscapularis, as seen in overhand throwers, on glenohumeral joint kinematics and contact pressures [87]. In this study, the authors replicated multiple lines of pull for the rotator cuff, deltoid, pectoralis major, and latissimus dorsi, using all muscles in the transverse and coronal force couples. They concluded that less force on the subscapularis lead to a significant increase in external rotation and posterosuperior glenohumeral contact pressure. This can be attributed to the disrupted transverse force couple leading to an imbalance between anterior and posterior forces as the strong moment arm of the infraspinatus, usually restrained by the subscapularis, now has a relatively stronger moment arm due to the weakened subscapularis moment arm, leading to posterosuperior translation.
1.3 Kinematics of Throwing
The kinematics of throwing is complex with tremendous forces acting on the shoulder. The throwing motion requires a rapid transmission of force from the lower extremity and core to the upper extremity. These immense forces and velocities place the shoulder at great risk of injury, especially in the setting of improper mechanics. An intricate and complex interplay between the scapula, humerus, and scapulohumeral and scapulothoracic muscles is required to maintain glenohumeral stability throughout all phases of throwing. Overhead throwing can be divided into five stages: windup, early cocking, late cocking, acceleration, and deceleration with follow-through (Fig. 1.7).
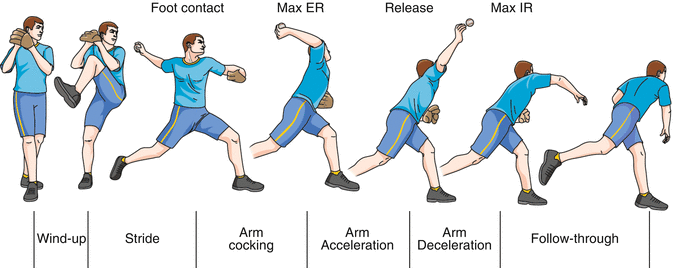
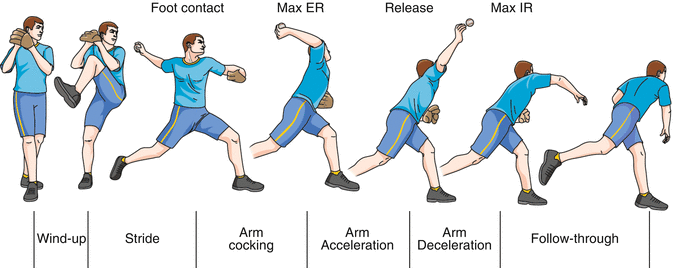
Fig. 1.7
Schematic of the phases of throwing motion from windup to follow-through. ER external rotation, IR internal rotation (Fleisig et al. [111])
1.3.1 Windup
Windup begins with the stride foot stepping backward, away from home plate, and the arms are lifted upward, often overhead. The pivot foot rotates to be parallel on the rubber as weight is transferred to it. This stage ends with the ball leaving the glove hand and the body balanced on the pivot foot. During the windup, EMG activity of the shoulder girdle and upper extremities is low. Instead, there is significant activity in the trunk and lower extremities as energy is stored for transfer to the throwing arm [88]. In the last moments of this phase, stance limb stability is provided by the gluteus medius. This is important for all pitchers, as it provides a stable base to initiate the pitch and minimize anterior-to-posterior sway of the body.
1.3.2 Early Cocking
During early cocking, the hip of the pivot leg slightly flexes in preparation for extension during late cocking and acceleration phase. The gluteus maximus is important in providing this propulsion. The pivot leg propels forward the stride leg, the nondominant upper extremity, and trunk. It is important that the pitcher stride rather than rotate too early. This “opening” of the pelvis and trunk pivots the body instead of propelling forward. While this force transmission and propulsion is occurring in the legs and torso, the trapezius and the serratus anterior muscles form a force couple to upwardly rotate and protract the scapula. This scapula motion is essential to place the glenoid in a stable position for the abducting and rotating humeral head. If the scapula is not positioned correctly, impingement can occur [89]. The deltoid and supraspinatus muscles act in synergy to abduct the humerus. The deltoid provides much of the abduction force with the supraspinatus fine tuning the position of the humeral head in the glenoid [90]. The remainder of the rotator cuff muscles have less activity during this phase, due to the lack of rotational forces applied to the humerus. As the stride foot strikes the ground during late cocking, the biceps becomes mildly active as the elbow is flexed. The hand should be on top of the ball, preventing early external rotation and supination which can decrease velocity.
1.3.3 Late Cocking
Late cocking begins when the stride leg makes contact with the ground with rapid forward motion of the trunk [88]. The nondominant lead shoulder rotates forward and horizontal abduction of this shoulder is minimized by keeping the lead arm closed in front of the body. This optimizes the centripetal forces by keeping more mass close to the center of rotation of the trunk. Abduction of the humerus is maintained and external rotation increases up to 170° [91, 92]. Just before the arm reaches maximum external rotation, an internal rotation torque measuring 67 Nm, shoulder compression force measuring 1,090 N and anterior force measuring 380 N occur. Static and dynamic restraints combine to stabilize against these forces. In this position, the primary static anterior stabilizer of the glenohumeral joint is the anterior band of the inferior glenohumeral ligament complex [21]. While the supraspinatus and the deltoid activity diminish as the humerus ceases to abduct, the subscapularis increases activity to act as a dynamic stabilizer to help center the humeral head [30]. The subscapularis acts as a barrier to anterior translation, together with the pectoralis major and the latissimus dorsi. These muscles act as a dynamic sling to augment the anterior-inferior glenohumeral ligament. The latissimus and teres major act eccentrically as they are internal rotators of the humerus. The infraspinatus and teres minor show increased EMG activity as they act to externally rotate the humerus. Additionally, the posterior rotator cuff functions as a checkrein by preventing excessive anterior subluxation. The scapulothoracic muscles continue to be active to produce a stable platform for the humeral head and to enhance maximal humeral external rotation [89]. The middle portion of the trapezius, the rhomboids, and the levator scapulae are all important in providing this scapular stabilization. The serratus anterior is also important in opposing retraction of the scapula.
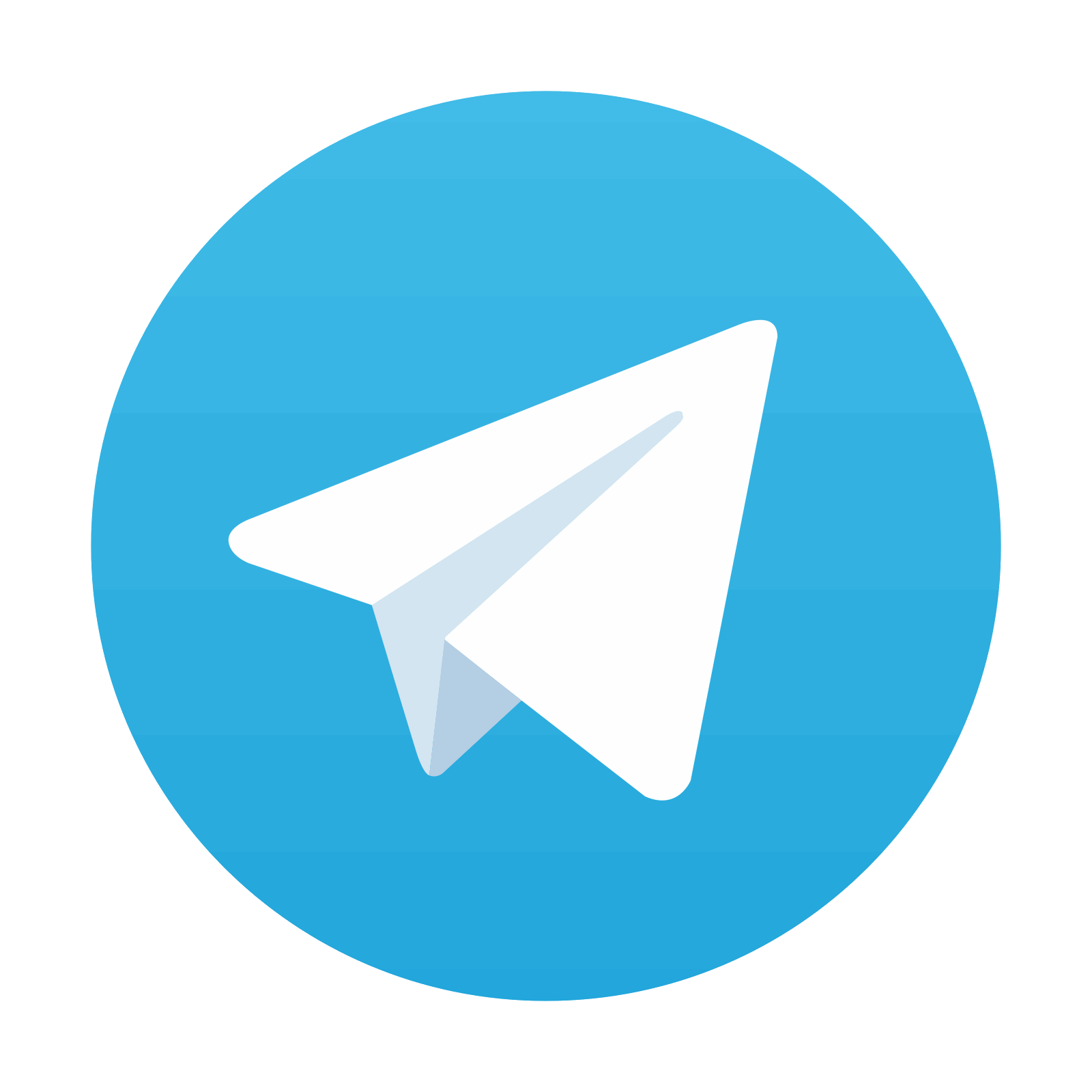
Stay updated, free articles. Join our Telegram channel

Full access? Get Clinical Tree
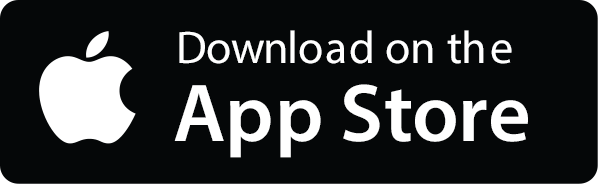
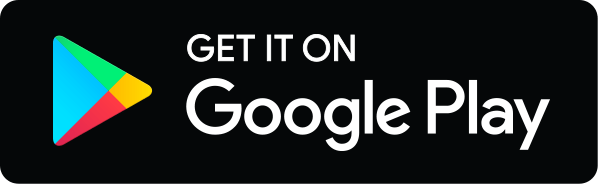