Table 9.1 MR Examinations of the Shoulder, Arm, and Brachial Plexus at 1.5 T | |||||||||||||||||||||||||||||||||||||||||||||||||||||||||||||||||||||||||||||||||||||||||||||||||||||||||||||||||||||||||||||||||||||||||||||||||||||||||||||||||||||||||||||||||||||||||||||||||||||||||||
---|---|---|---|---|---|---|---|---|---|---|---|---|---|---|---|---|---|---|---|---|---|---|---|---|---|---|---|---|---|---|---|---|---|---|---|---|---|---|---|---|---|---|---|---|---|---|---|---|---|---|---|---|---|---|---|---|---|---|---|---|---|---|---|---|---|---|---|---|---|---|---|---|---|---|---|---|---|---|---|---|---|---|---|---|---|---|---|---|---|---|---|---|---|---|---|---|---|---|---|---|---|---|---|---|---|---|---|---|---|---|---|---|---|---|---|---|---|---|---|---|---|---|---|---|---|---|---|---|---|---|---|---|---|---|---|---|---|---|---|---|---|---|---|---|---|---|---|---|---|---|---|---|---|---|---|---|---|---|---|---|---|---|---|---|---|---|---|---|---|---|---|---|---|---|---|---|---|---|---|---|---|---|---|---|---|---|---|---|---|---|---|---|---|---|---|---|---|---|---|---|---|---|---|
|
appreciated and classified. Signal intensity in the rotator cuff increases from proton density to T2-weighted images when partial or complete cuff tears are present. Signal intensity does not increase significantly in areas of tendinosis on T2-weighted sequences.25,26,27
capsular distention that aids delineation of the labroligamentous structures.
![]() Figure 9.6 Illustrations of anterior injection sites with the patient supine (A) and slightly rotated (B). |
![]() Figure 9.7 Illustration of injection site for MR arthrography using the anterior inferior approach. (From Berquist TH. Imaging of Orthopedic Trauma, 2nd ed. New York: Raven Press; 1992.) |
rotated superiorly to align the glenohumeral articular surface. Patient position is supported by bolsters. After sterile preparation, the skin is marked over the lower medial humeral head. Following anesthetic injection, a spinal needle is advanced vertically until it contacts the humeral head. Intra-articular position is confirmed with iodinated contrast.51,53
![]() Figure 9.9 Illustration of the typical anterior injection site (A) through the subscapularis and higher (B) in the rotator cuff interval between the subscapularis and supraspinatus. |
or proximal nerve root abnormalities. These techniques are fully discussed in Chapter 5. Evaluation of the brachial plexus requires images from the mid cervical spine to the humerus, so a large FOV is necessary (Fig. 9.12). A torso coil is commonly employed. If symptoms are unilateral, a smaller off-center FOV can be used. Comparison is helpful, so we often examine both brachial plexus regions simultaneously. The axial images (right, left, or bilateral) are obtained using cardiac and respiratory gating to minimize the artifact from respiratory and cardiac motion. Slice thickness of 5 mm with 1.5-mm skip and an 18- (unilateral) or 36±-(bilateral) cm FOV with 256 × 256 matrix and 1 to 2 acquisitions are commonly used. Axial images are usually obtained from the mid cervical level (C3-C4) to the mid humerus, which allows the lower neck, shoulder, and upper arm to be completely included in the FOV (Fig. 9.13).
bundle. In most situations, the axial and sagittal images provide an adequate screening examination for brachial plexus pathology. We typically add coronal and axial turbo inversion recovery (TIR) images (Fig. 9.15). Post-contrast fat-suppressed T1-weighted images are useful for evaluation of neural inflammation and characterizing adjacent masses or soft tissue abnormalities. Technical details are discussed in depth in the clinical applications section of this chapter.
glenohumeral ligament. The transverse ligament extends across the greater and lesser tuberosities, enclosing the synovial sheath and long head of the biceps tendon.69,70,71
with rotator cuff tears.4,12,64,72 The supraspinatus muscle arises from the supraspinous fossa of the scapula and is covered by the trapezius in its proximal portion. As the muscle extends peripherally, it passes under the acromion, coracoclavicular ligament, and AC joint (Fig. 9.25) to insert on the most superior of the three facets of the greater tuberosity. Tendon of the supraspinatus is broad, covering the top of the shoulder and blending with the capsule superiorly. The primary function of the supraspinatus is to assist the deltoid in abduction of the humerus (Table 9.2).61,69,73,74
separated from the scapula by a bursa that sometimes communicates with the shoulder joint (Table 9.3). The infraspinatus is separated from the supraspinatus by the scapular spine (Figs. 9.16,8.17,9.18). Its tendon forms the upper posterior portion of the rotator cuff and inserts in the greater tuberosity posterior and inferior to the supraspinatus tendon.69,72,75,76,77,78
passing across and anterior to the muscle (Fig. 9.26). The subscapularis bursa or recess lies between the muscle and the neck of the scapula and can communicate with the shoulder joint (Table 9.3). The primary function of the subscapularis is internal rotation of the humerus (Table 9.2).61,69
![]() Figure 9.19 Illustration of the shoulder, demonstrating the glenohumeral and acromioclavicular joints and surrounding structures. |
![]() Figure 9.21 Illustration of the shoulder, demonstrating the glenoid articular surface, labrum, and supporting structures. Radial image planes and labral configuration are demonstrated. |
pectoralis major is a large, triangular, flat muscle covering much of the upper thorax. The pectoralis major has an extensive origin from the medial inferior aspect of the clavicle, the lateral margin of the sternum, and the chondral junctions of the upper ribs. The fibers of this muscle converge to form a broad tendon that passes just anterior to coracobrachialis and biceps brachii. The muscle then passes deep to the anterior margin of the deltoid to insert on the crest of the greater tubercle or lateral aspect of the intertubercular groove. The pectoralis major is a strong adductor of the humerus and is innervated by two nerves, the lateral and medial (anterior thoracic) nerves (Table 9.2).61,69
![]() Figure 9.24 Illustration of the glenohumeral ligaments (A) and their relationship to the coracohumeral ligament (B). |
Table 9.2 Muscles of the Shoulder and Upper Arm | |||||||||||||||||||||||||||||||||||||||||||||||||||||||||||||||||||||||||||||||||||||||||||||||||||||||||
---|---|---|---|---|---|---|---|---|---|---|---|---|---|---|---|---|---|---|---|---|---|---|---|---|---|---|---|---|---|---|---|---|---|---|---|---|---|---|---|---|---|---|---|---|---|---|---|---|---|---|---|---|---|---|---|---|---|---|---|---|---|---|---|---|---|---|---|---|---|---|---|---|---|---|---|---|---|---|---|---|---|---|---|---|---|---|---|---|---|---|---|---|---|---|---|---|---|---|---|---|---|---|---|---|---|
|
to insert on the coracoid process of the scapula. Its chief action is to depress the angle of the scapula. Nerve supply is via the medial pectoral nerve (C8-T1) (Table 9.2).61
Table 9.3 Shoulder Bursae | ||||||||||||||||||||||||||||||||||||
---|---|---|---|---|---|---|---|---|---|---|---|---|---|---|---|---|---|---|---|---|---|---|---|---|---|---|---|---|---|---|---|---|---|---|---|---|
|
![]() Figure 9.26 A and B: Coronal T1-weighted images through the anterior shoulder, demonstrating the subscapularis and adjacent neurovascular structures. |
(Figs. 9.14 and 9.15). At this level, the lateral cord and a major portion of the posterior cord lie lateral and superior to the axillary artery. The lower trunk of the medial cord is posterior to the artery, with the major continuation of the other groups in close approximation to the vessel (Figs. 9.15 and 9.27). On sagittal images, this forms a closely approximated group of low-intensity structures (Fig. 9.14).6,59,61,69
occur less frequently in the shoulder compared to the elbow and wrist. Motion artifacts may be related to pain, tremor, respiratory motion, or forced external or abduction-external rotation, which may be difficult positions to maintain (Fig. 9.30).2,85 Motion artifacts can be reduced by increasing patient comfort, positioning, and using faster sequences to reduce imaging time.2,12 Flow artifacts are a less significant problem. Artifact occurs in the phase encoding direction. Therefore, phase encoding directions should be selected on the basis of the suspected pathology. A transverse phase encoding should be selected for rotator cuff disease (Fig. 9.31) to reduce confusion that may occur when flow artifact is in the superior-inferior direction that would pass through the rotator cuff.2,89
example, Theodoropoulos et al.84 demonstrated significant performance differences comparing musculoskeletal trained radiologists and general radiologists for technique and interpretation. Improper injection of contrast can result in extravasation (Fig. 9.8), which can be confused with pathology.50,81,83,84 Inadvertent injection of air bubbles may cause areas of low signal intensity on MR arthrograms that mimic loose bodies. “Blooming” may cause the air bubble to appear larger due to magnetic susceptibility artifact. This phenomenon is more common on GRE images.81
![]() Figure 9.31 Coronal T2-weighted fast spin-echo image with fat suppression. The flow artifact (arrows) runs transversely. |
![]() Figure 9.32 Coronal SE 500/11 sequence demonstrating increased signal (white arrow) in the supraspinatus that is angled at 55° to the magnet bore (white line). |
subdeltoid bursa can also be detected in patients without an associated rotator cuff tear.73,97 However, other conditions such as impingement, bursitis, and tendinitis may be associated with fluid in the bursa.47 Fat-suppression techniques may be useful to reduce problems caused by chemical shift artifacts that occur at fat-water interfaces.73,97
glenohumeral ligament. When the superior ligament is thicker, the middle glenohumeral ligament may be absent or thinner.12,37,100 The superior glenohumeral ligament may be absent in up to 10% of patients.37
inflammation or communication with the joint via a rotator cuff tear or capsular defect (Figs. 9.19 and 9.40A).12,36,61
injections. Fluid typically remains up to 48 hours after an injection.81,108
rotator cuff, labrum, fluid changes, and marrow changes are most frequently discussed in the literature as noted above. Other variants, such as tendon attachments (Fig. 9.35A), can be confused with osteophytes.86 Confusion can be avoided by comparing MR findings with radiographic features. Other findings such as soft tissue calcifications (Fig. 9.47) provide additional value for radiographic comparison when interpreting MR images.
coracoclavicular sprain. Stress radiographs demonstrate subluxation of the joint. MR images demonstrate increased signal in the AC ligaments and marrow edema in the acromion and clavicle. There may also be increased signal intensity in the coracoclavicular ligament.135 Axial and sagittal image planes are most useful. Type III injuries result in disruption of the AC and coracoclavicular ligaments. The joint dislocates radiographically. MR images demonstrate increased signal intensity in both ligament complexes (Table 9.4) and widening with varying degrees of joint subluxation or dislocation.135,143 The deltoid and trapezius muscles may be detached from the distal clavicle.135 All three image planes maybe required toassess the soft tissue and articular changes completely. A fracture at the base of coracoid has also been reported instead of coracoclavicular ligament disruption.143 Fractures of the coracoid base are most obvious on sagittal images. Type IV dislocations result in posterior displacement of the clavicle which is most easily appreciated on axial
images.135 Sternoclavicular dislocations may also occurwith this injury. Therefore, both medial and lateral ends of the clavicle should be imaged.135,143 Type IV dislocations are similar to type III except the trapezius and deltoid muscles are stripped from the ends of the clavicle and acromion. The clavicle is significantly elevated by cephalad traction of the sternocleidomastoid muscle. This feature is best appreciated on coronal images. Type VI dislocations result from severe force from superior to the clavicle with the humerus abducted.143 Type IV injuries may be most easily identified on coronal or sagittal images.
![]() Figure 9.47 Anteroposterior radiograph demonstrates dense calcification in the supraspinatus tendon. |
![]() Figure 9.50 Coronal proton density-weighted image demonstrating a Hill-Sachs lesion (arrow) not evident on routine radiographs. |
positive clinical examination. Other changes did not correlate with clinical findings.116 Schweitzer et al.148 reported joint effusions in 66% of patients with grade 2 or 3 impingement compared with 12% in normal volunteers.
Table 9.4 Acromioclavicular Joint Injuries | ||||||||||||||||||||||||
---|---|---|---|---|---|---|---|---|---|---|---|---|---|---|---|---|---|---|---|---|---|---|---|---|
|
![]() Figure 9.53 Axial T1-weighted image shows residual erosive changes (arrow) in the distal clavicle after traumatic osteolysis. |
![]() Figure 9.54 Chronic untreated traumatic osteolysis with marked joint hypertrophy on this sagittal T1-weighted image. |
Table 9.5 Etiologies of Rotator Cuff Tears | |||||||||||||||||
---|---|---|---|---|---|---|---|---|---|---|---|---|---|---|---|---|---|
|
impingement, etc.) and primary cuff degeneration, which may be ischemic.
![]() Figure 9.55 Illustration demonstrating the coracoacromial arch and supporting structures of the shoulder. Note the coracoacromial ligament (arrow). |
has also been implicated in impingement (Fig. 9.57) and rotator cuff tears.115,168,178,179 A type 1 acromial configuration is straight; type 2, curved; type 3, hooked; and type 4 has a convex inferior surface (Fig. 9.57).21,31 Anterior or lateral angulation of the acromion may also cause impingement (Fig. 9.58).178,186 Type 1 acromions occur in 18% to 23%; type 2 in 42% to 68%; and type 3 10% to 39% of patients. Type 4 acromia occur in 7% of the general population.12,16,179 Type 3 or hooked acromia are more common in males.180 Zlatkin and Falchook reported rotator cuff tears in 51% of patients with type 3 acromia, os acromiale, or anterior inferior acromial bone spurs (Fig. 9.59). More recent studies noted type 3 acromia in 70% to 80% of rotator cuff tears. Only 3% of patients with rotator cuff tears had type 1 acromia.181
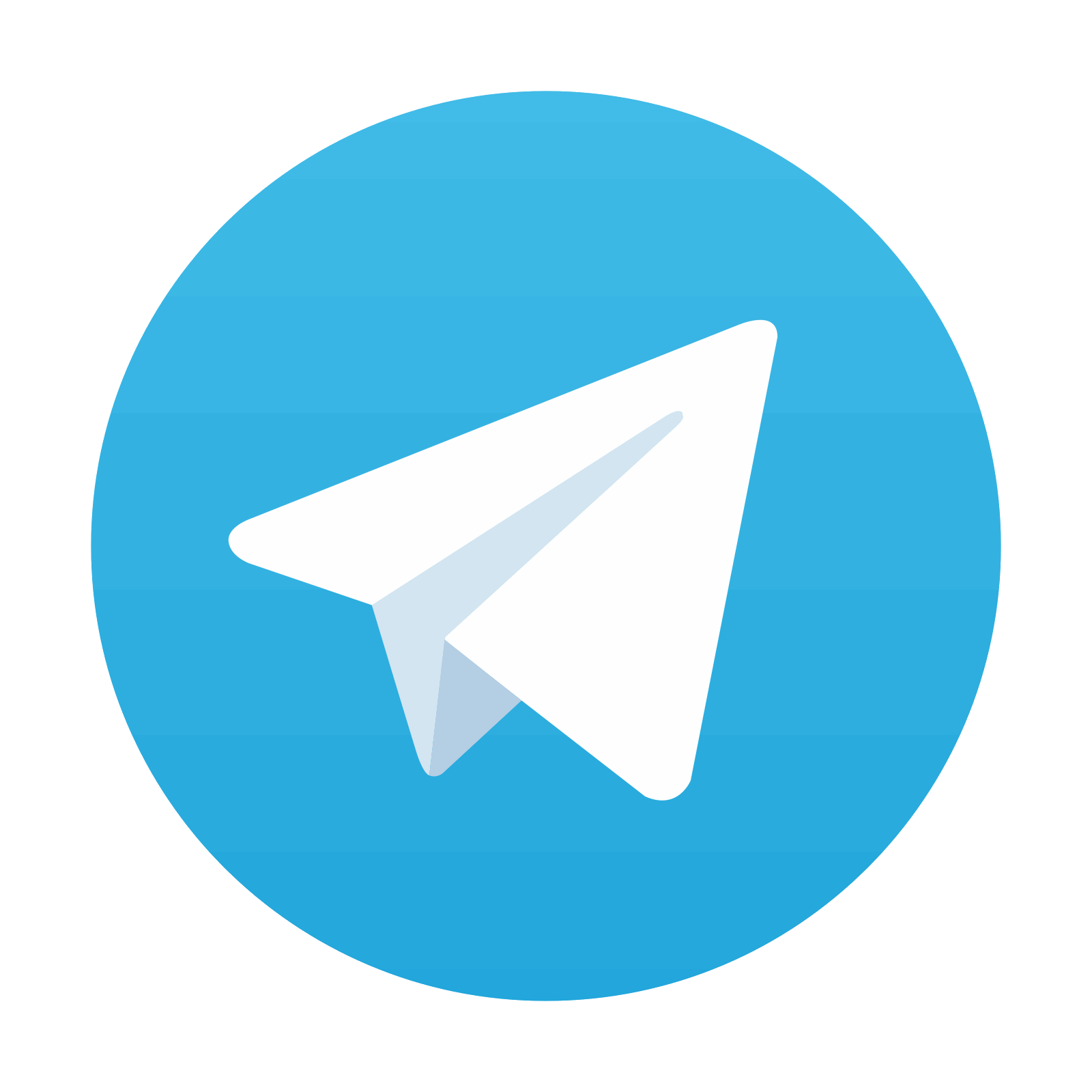
Stay updated, free articles. Join our Telegram channel

Full access? Get Clinical Tree
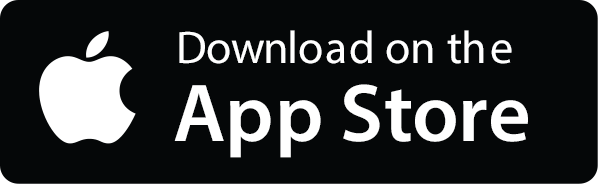
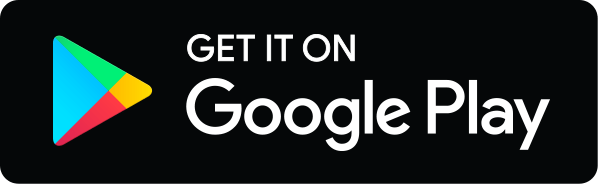
