Chapter 45 Knee Injuries
Anatomy
Extraarticular Tendinous Structures
Pes anserinus is the term for the conjoined insertion of the sartorius, gracilis, and semitendinosus muscles along the proximal medial aspect to the tibia. These primary flexors of the knee have a secondary internal rotational influence on the tibia and help protect the knee against rotary and valgus stress. Their counterpart on the lateral side of the knee is the strong biceps femoris insertion into the fibular head, lateral tibia, and posterolateral capsular structures. This muscle is a strong flexor of the knee that also produces simultaneous strong external rotation of the tibia. It provides rotary stability by preventing forward dislocation of the tibia on the femur during flexion. Its contributions to the arcuate ligament complex at the posterolateral corner of the knee also provide varus and rotary stability. The iliotibial tract, the posterior third of the iliotibial band, inserts proximally into the lateral epicondyle of the femur and distally into the lateral tibial tubercle (Gerdy tubercle). It thus forms an additional ligament that is contiguous anteriorly with the vastus lateralis and posteriorly with the biceps. The iliotibial band moves forward in extension and backward in flexion but is tense in both positions. During flexion, the iliotibial band, the popliteal tendon, and the lateral collateral ligament cross each other, whereas the iliotibial band and biceps tendon remain parallel to each other as in extension, all serving to enhance lateral stability (Fig. 45-1).
The popliteus muscle has three origins, the strongest of which is from the lateral femoral condyle. Other important origins are from the fibula (popliteofibular ligament) and from the posterior horn of the lateral meniscus. The femoral and fibular origins form the arms of an oblique Y-shaped ligament, the arcuate. The arms are joined together by the capsule and meniscal origin. The arcuate ligament is not a separate ligament but is a condensation of the fibers of the origin of the popliteus (Fig. 45-2). With electromyographic studies, Basmajian and Lovejoy found that the popliteus muscle is a prime medial rotator of the tibia during the initial stages of flexion and also acts to withdraw the meniscus during flexion. In addition, it supplies rotary stability to the femur on the tibia and aids the posterior cruciate ligament in preventing forward dislocation of the femur on the tibia.
The semimembranosus muscle is especially important as a stabilizing structure around the posterior and posteromedial aspects of the knee. It has five distal expansions (Fig. 45-3). The first is the oblique popliteal ligament, which passes from the insertion of the semimembranosus on the posteromedial aspect of the tibia obliquely and laterally upward toward the insertion of the lateral gastrocnemius head (Fig. 45-4A). It acts as an important stabilizing structure on the posterior aspect of the knee. The semimembranosus helps tighten this ligament with contraction (see Fig. 45-4B). When the oblique popliteal ligament is pulled medially and forward, it tightens the posterior capsule of the knee. This maneuver can be used to tighten the posterior capsule in the posteromedial corner of the knee in surgical repair. A second tendinous attachment is to the posterior capsule and posterior horn of the medial meniscus. This tendinous slip helps tighten the posterior capsule and pulls the medial meniscus posteriorly during knee flexion. The anterior or deep head continues medially along the flare of the tibial condyle and inserts beneath the superficial tibial collateral ligament just distal to the joint line. The direct head of the semimembranosus attaches to the tubercle on the posterior aspect of the medial condyle of the tibia just below the joint line. This tendinous attachment provides a firm point in which sutures can be anchored for posteromedial capsular repair. The distal portion of the semimembranosus tendon continues distally to form a fibrous expansion over the popliteus and fuses with the periosteum of the medial tibia. The semimembranosus, through its muscle contraction, tenses the posterior capsule and posteromedial capsular structures, providing significant stability. Functionally, it acts as a flexor of the knee and internal rotator of the tibia.
Extraarticular Ligamentous Structures
The joint capsule and the collateral ligaments are the principal extraarticular static stabilizing structures. The capsule is a sleeve of fibrous tissue extending from the patella and patellar tendon anteriorly to the medial, lateral, and posterior expanses of the joint. The menisci are attached firmly at the periphery to this capsule, especially so medially and less so laterally. Laterally, the passage of the popliteal tendon through the popliteal hiatus to its origin on the femoral condyle produces a less secure meniscal attachment than is present medially. The medial capsule is more distinct and well defined than its lateral counterpart. The capsular structures, along with the medial and lateral extensor expansions of the powerful quadriceps musculature, are the principal stabilizing structures anterior to the transverse axis of the joint. The capsule is especially reinforced by the collateral ligaments and the medial and lateral hamstring muscles as well as by the popliteus muscle and the iliotibial band posterior to the transverse axis. The medial and lateral “quadruple complexes” have been identified as principal stabilizers of the knee (Fig. 45-5). The medial quadruple complex is made up of the medial collateral ligament, the semimembranosus, the tendons of the pes anserinus, and the oblique popliteal ligament portion of the posterior capsule. The lateral quadruple complex is made up of the iliotibial band, the lateral collateral ligament, the popliteal tendon, and the biceps femoris. The capsule is reinforced posteriorly by the oblique popliteal ligament, at the posteromedial corner by the ramifications of the semimembranosus, and posterolaterally by the structures contributing to the arcuate complex.
The posteromedial region of the medial capsule extends from the posterior edge of the medial collateral ligament posteriorly to the insertion of the direct head of the semimembranosus. Hughston described this posterior oblique ligament as a thickening of the medial capsular ligament attached proximally to the adductor tubercle of the femur and distally to the tibia and posterior aspect of the capsule. The distal attachment is composed of three arms: (1) the prominent central, or tibial, arm, which attaches to the edge of the posterior surface of the tibia close to the margin of the articular surface and central to the upper edge of the semimembranosus tendon; (2) the superior, or capsular, arm, which is continuous with the posterior capsule and the proximal part of the oblique popliteal ligament; and (3) the poorly defined inferior, or distal, arm, which attaches distally both to the sheath covering the semimembranosus tendon and to the tibia just distal to the direct insertion of the semimembranosus tendon (Figs. 45-6 to 45-9).
The medial collateral ligament is a long, rather narrow, well-delineated structure lying superficial to the medial capsule and capsular ligaments, originating on the medial epicondyle and inserting 7 to 10 cm below the joint line on the posterior half of the medial surface of the tibial metaphysis deep to the pes anserinus tendons. It has been referred to as the superficial tibial collateral ligament or the superficial portion of the medial collateral ligament. Biomechanical studies have shown that it provides the principal stability to valgus stresses. It glides forward over the side of the femoral condyle in extension and posteriorly in flexion (Fig. 45-10). The long fibers of the medial collateral ligament are the primary stabilizers of the medial side of the knee against valgus and external rotary stress. The anterior fibers of the ligament tighten as the knee flexes, with fibers more posteriorly becoming slack (Fig. 45-11).
In their classic study of knee anatomy, Warren and Marshall divided the knee into three layers. Layer I includes the deep fascia or crural fascia; layer II is composed of the superficial medial collateral ligament, various structures anterior to this ligament, and the ligaments of the posteromedial corner; and layer III is made up of the capsule of the knee joint and the deep medial collateral ligament. Layer I is the first fascial plane encountered after a skin incision. Its plane is defined by the fascia that invests the sartorius muscle (Fig. 45-12). Proceeding posteriorly, layer I is a thin sheet that overlies the two heads of the gastrocnemius and the structures of the popliteal fossa. If a vertical incision is made in layer I posterior to the parallel fibers of the medial collateral ligament and the anterior portion of layer I is reflected forward, the entire superficial medial collateral ligament is exposed (Fig. 45-13). Inferiorly, the tendons of the gracilis and semitendinosus can be seen as distinct structures that can be separated from layer I superficially and layer II beneath (Fig. 45-14). Layers I and II are separated by these tendons as they cross to their insertions on the tibia.
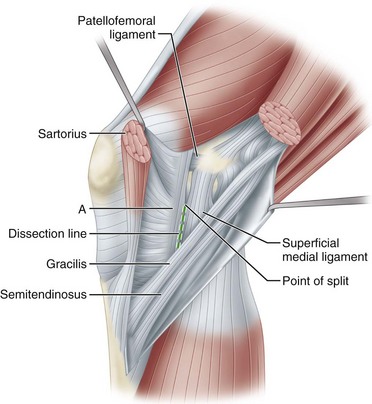
FIGURE 45-13 With sartorius transected and layer I held open with forceps, split in layer II can be seen just anterior to superficial medial ligament. Anterior to superficial medial ligament above dissection line, layer II joins layer I to become part of retinacular tendons (A). “Incision” will be made along “dissection line” marked here so that layer II can be reflected farther forward as shown in Figure 45-16.
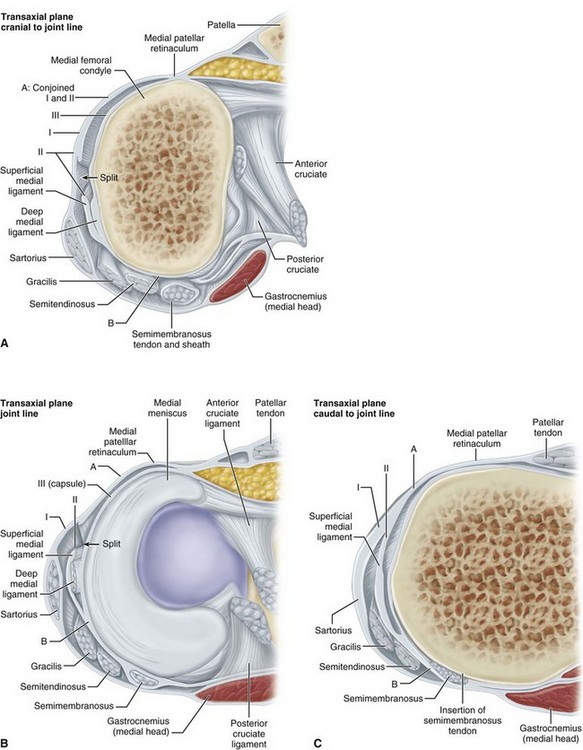
FIGURE 45-14 A, At this level, retinacular fibers of layer II anterior to superficial medial ligament have joined layer I. Point of junction is shown at A; B indicates point where layer II and layer III merge with each other and with tendon sheath of semimembranosus. B, Cross section at level of joint line. At this level, “split” can be seen where retinacular fibers anterior to superficial medial ligament leave layer II and superficial medial ligament to join layer I. C, Cross section approximately 1 cm inferior to joint line. Note insertions of semimembranosus posteriorly and medially. Deep medial ligament is not seen because its attachment to tibia is below joint line, above this level (see Fig. 45-17).
The plane of layer II is clearly defined by the parallel fibers of the superficial medial collateral ligament. As layer II is traced posteriorly from the anterior edge of this ligament, the fibers joining the femur to the tibia become more oblique in their orientation (Fig. 45-15). At the posteromedial corner of the knee, layer II merges with layer III and with the tendon sheath of the semimembranosus (Figs. 45-16 and 45-17; see also Fig. 45-14). The conjoined structure formed by layer II and layer III extends posteriorly to form the posteromedial capsule that envelops the medial condyle of the femur. This posteromedial capsule is augmented by fibers from the semimembranosus tendon sheath. Most of the semimembranosus tendon inserts onto bone through the direct insertion at the posteromedial corner of the tibia just below the joint line (see Fig. 45-16). A more anterior insertion, which is an extension of the direct insertion, proceeds around the medial side of the tibia just below the joint line. This anterior insertion lies deep to the superficial medial collateral ligament and layer II and distal to the tibial margin of the capsule, or layer III (see Figs. 45-14C and 45-16). These two insertions do not participate in any of the three layers, because they go directly to bone. The semimembranosus tendon sheath sends fibrous extensions upward and downward into layer II (see Figs. 45-15 to 45-17). The most clearly defined of these fiber tracts are the ones that extend directly upward over the medial femoral condyle and across the back of the knee to the lateral condyle, forming the oblique popliteal ligament (see Fig. 45-16). A third, smaller extension of the semimembranosus sheath runs distally to insert on the tibia posterior to the inferior oblique portion of the superficial medial collateral ligament and to blend with the oblique fibers of that ligament to a varying degree. Judging from their morphological features, these fibers do not appear to have much functional significance.
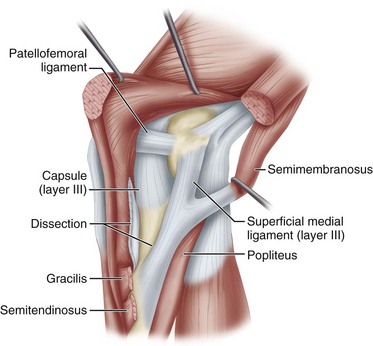
FIGURE 45-15 With split extended distally along dissection line shown in Figure 45-14, layer I and part of layer II anterior to split and dissection line are reflected forward to expose capsule anterior to superficial ligament. Long parallel and oblique fibers of superficial medial ligament can be seen posteriorly (layer II) and capsule anteriorly (layer III).
Layer III is the true capsule of the knee joint, attached above and below the joint at the margins of the articular surfaces (Fig. 45-18). The anterior part of the capsule is thin. It does not appear to function as a stabilizing ligament and simply envelops the fat pad. Beneath the superficial medial collateral ligament, layer III becomes thicker and forms a vertically oriented band of short fibers, variably known as the deep medial collateral ligament, deep layer of the medial collateral ligament, or medial capsular ligament (see Figs. 45-14A and B and 45-18). This deep ligament extends from the femur to the midportion of the peripheral margin of the meniscus and tibia (see Fig. 45-17). Elsewhere the capsule is thin. The deep and superficial ligaments are readily separated where they are in direct contact, but farther posteriorly, 1 to 2 cm behind the anterior edge of the superficial medial collateral ligament, layers II and III blend. The result is that the meniscofemoral portion of the deep ligament tends to merge with the overlying superficial ligament near its proximal attachment (see Figs. 45-14A to C and 45-18). The meniscotibial ligament (coronary ligament) is consistently separated readily from the overlying superficial ligament. Farther posteriorly, layer III merges with layer II (see Fig. 45-14A to C), and their combined fibers envelop the posteromedial corner of the joint, forming a composite structure.
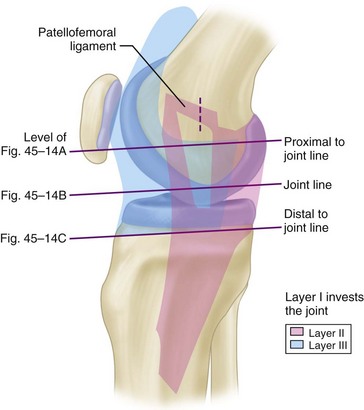
FIGURE 45-18 Blue area represents layer III, capsule. Posterior extent is deep medial ligament. Anteriorly, it invests entire joint. Pink area represents layer II as it appears when layer I is reflected as far forward as possible. Inferior angle of multicolor triangle is at point of split in layer II. Lines indicate level of cross-sectional diagrams (see Fig. 45-14).
Warren et al. identified a strong direct attachment of the popliteal tendon to the fibula, which has been called the popliteal fibular fascicle and the fibular origin of the popliteus muscle. These researchers called this structure the popliteofibular ligament because it connects the fibula to the femur through the popliteal tendon (Fig. 45-19). This ligament is located deep to the lateral limb of the arcuate ligament; it originates from the posterior part of the fibula and posterior to the biceps insertion and joins the popliteal tendon just proximal to its musculotendinous junction. Thus the popliteus muscle-tendon unit is a Y-shaped structure with a muscle origin from the posterior part of the tibia, a ligamentous origin from the fibula, and a united insertion on the femur. The popliteal tendon has a constant, broad-based femoral attachment at the most proximal and anterior fifth of the popliteal sulcus. The popliteal tendon attachment on the femur is always anterior to the lateral collateral ligament. The average distance between the femoral attachments of the popliteal tendon and the lateral collateral ligament is 18.5 mm. The popliteofibular ligament has two divisions, anterior and posterior. The average attachment of the posterior division is 1.6 mm distal to the posteromedial aspect of the tip of the fibular styloid process, and the anterior division attaches 2.8 mm distal to the anteromedial aspect of the tip of the fibular styloid process. Selective cutting studies confirmed that the popliteal tendon attachments to the tibia and the popliteofibular ligament are important in resisting posterior translation, varus rotation, and external rotation.
Seebacher, Inglis, Marshall, and Warren defined three distinct layers of the lateral structures of the knee. The most superficial layer, or layer I, has two parts: (1) the iliotibial tract and its expansion anteriorly and (2) the superficial portion of the biceps femoris and its expansion posteriorly (Figs. 45-20 and 45-21). The peroneal nerve lies on the deep side of layer I, just posterior to the biceps tendon. Layer II is formed by the retinaculum of the quadriceps, most of which descends anterolaterally and adjacent to the patella.
Posteriorly, layer II is incomplete and is represented by the two patellofemoral ligaments. The proximal ligament joins the terminal fibers of the lateral intermuscular septum; the distal one ends posteriorly at the fabella or at the insertions of the posterolateral capsular reinforcements and of the lateral head of the gastrocnemius on the femoral condyle (see Figs. 45-20 and 45-21). The patellomeniscal ligament also is part of layer II. It extends obliquely from the patella, attaches to the margin of the lateral meniscus, and terminates inferiorly on the lateral tibial (Gerdy) tubercle deep to the iliotibial tract. Layers I and II are adherent to each other in a vertical line at the lateral margin of the patella. Discrete attachments of the uppermost fibers of the patellofemoral ligament to the overlying iliotibial tract occur just below the termination of the lateral intermuscular septum at the lateral femoral epicondyle.
Layer III, the deepest layer, is the lateral part of the joint capsule (Fig. 45-22). It is attached to the edges of the tibia and femur circumferentially in horizontal planes at the proximal and distal ends of the knee joint. The capsular attachment to the outer edge of the lateral meniscus is called the coronary ligament. The popliteal tendon passes through a hiatus in the coronary ligament to attach to the femur. Just posterior to the overlying iliotibial tract, the capsule divides into two layers. It encompasses the lateral collateral ligament and ends posteriorly at the variably sized fabellofibular ligament (short external ligament) (see Figs. 45-20 and 45-22A). The deep lamina of the posterolateral part of the capsule passes along the edge of the lateral meniscus. The inner lamina terminates posteriorly at the Y-shaped arcuate ligament. These two capsular laminae always are separated from each other by the inferolateral geniculate vessels, which pass forward. Seebacher et al. noted three anatomical variations in their dissections: (1) the arcuate ligament alone reinforced the capsule in 13% of the knees; (2) the fabellofibular ligament alone reinforced the capsule in 20%; and (3) both of these ligaments reinforced the posterolateral aspect of the capsule in 67%. These variations were associated with variations in the size of the osseous cartilaginous fabella in the lateral head of the gastrocnemius. Most commonly, the fabellofibular and arcuate ligaments both were present and were of modest size. When the fabella was large, there was no arcuate ligament and the fabellofibular ligament was robust. Conversely, when the fabella or its cartilaginous remnant was absent, the fabellofibular ligament also was absent and only the arcuate ligament was present (Fig. 45-23; see also Figs. 45-20 and 45-22). Both the arcuate and fabellofibular ligaments insert on the apex of the fibular styloid process. They ascend vertically on the free edges of their respective capsular lamina to the lateral head of the gastrocnemius, where they are joined by the posterior termination of the oblique popliteal ligament. When present, the fabella was traversed by all of these ligaments.
Intraarticular Structures
The principal intraarticular structures of importance are the medial and lateral menisci and the anterior and posterior cruciate ligaments. Numerous functions have been assigned to the menisci, some known and some hypothetical. Among these functions are distribution of joint fluid, nutrition, shock absorption, deepening of the joint, stabilization of the joint, and a load-bearing or weight-bearing function. The cruciate ligaments function as stabilizers of the joint and axes around which rotary motion, both normal and abnormal, occurs. They restrict the backward and forward motion of the tibia on the femur and assist in the control of both medial and lateral rotation of the tibia on the femur. External rotation of the tibia produces an unwinding of the ligaments, and internal rotation produces a winding up of the cruciate ligaments (Fig. 45-24). Further discussion of their specific functions is presented in the section on cruciate ligament injuries.
Mechanics
An accurate plot of the contact points between the femur and tibia reveals that the rate of rolling to gliding does not remain constant through all degrees of flexion. This ratio is approximately 1 : 2 in early flexion and about 1 : 4 by the end of flexion (Fig. 45-25).
Menisci
Function and Anatomy
Meniscal function is essential to the normal function of the knee joint. As stated in the previous section on anatomy, various functions have been attributed to the menisci, some of which are known or proved and others that are theorized. The menisci act as a joint filler, compensating for gross incongruity between femoral and tibial articulating surfaces (Figs. 45-26 and 45-27). So located, the menisci prevent capsular and synovial impingement during flexion-extension movements. The menisci are believed to have a joint lubrication function, helping to distribute synovial fluid throughout the joint and aiding the nutrition of the articular cartilage. They undoubtedly contribute to stability in all planes but are especially important rotary stabilizers and are probably essential for the smooth transition from a pure hinge to a gliding or rotary motion as the knee moves from flexion to extension.
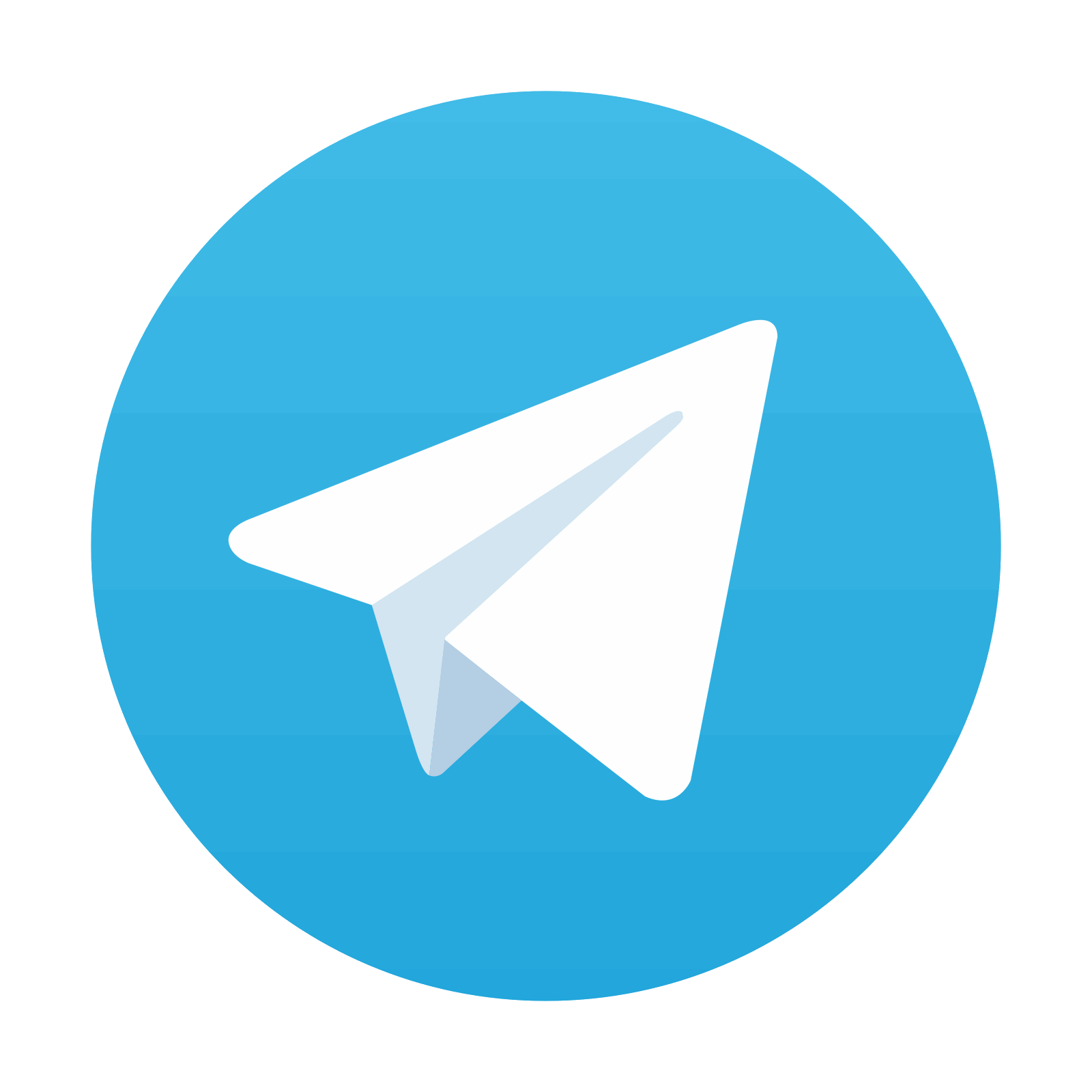
Stay updated, free articles. Join our Telegram channel

Full access? Get Clinical Tree
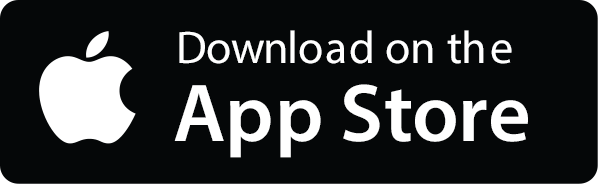
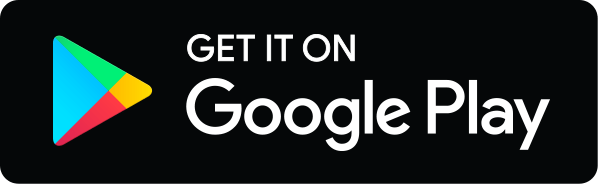