67 Intraoperative neurophysiologic monitoring (IONM) is the continuous use of electrophysiologic recording techniques to identify changes in the functional status of neural elements (spinal cord, spinal nerve roots, peripheral nerves) at risk for iatrogenic injury during the course of spinal surgery. It permits detection of changes in neural function early enough to initiate timely interventional measures, thereby minimizing the risk of postoperative neural deficit. In general, IONM is warranted whenever the cost of potential complications (e.g., medicolegal, extended hospital stay, rehabilitation, quality of life change) outweighs that of intraoperative monitoring fees. The monitoring techniques used most commonly during spinal surgery include (1) transcranial electrical motor evoked potentials (tceMEPs), (2) mixed-nerve somatosensory evoked potentials (SSEPs), (3) spontaneous electromyography (spEMG), and (4) stimulated electromyography (stEMG). These individual modalities should be combined to form a neuromonitoring battery that provides broad-scale neurophysiologic surveillance of the spinal cord motor and sensory tracts, nerve roots, and peripheral nerves/brachial plexus, which are potentially at risk for neural injury. TceMEPs are myogenic responses elicited by the application of electrical stimulation to the brain. Electrical pulse trains are applied through subdermal scalp electrodes that overlie motor cortex. Following depolarization of cortical neurons, efferent neural signals course down the internal capsule to the caudal medulla, where the majority of corticospinal tract (CST) fibers decussate and descend into the spinal cord motor pathways. CST axons then enter the spinal cord gray matter, interact with spinal cord interneurons, and go on to synapse with alpha motor neurons. Alpha motor neuron axons exit through their corresponding neural foramina as spinal nerve roots that innervate skeletal muscle. Mixed-nerve somatosensory evoked potentials (SSEPs) are elicited by electrical stimulation applied to a peripheral nerve (e.g., ulnar, median, posterior tibial, peroneal), which initiates an afferent neural volley that enters the spinal cord via the lumbosacral plexus. The afferent volley ascends within the posterior sensory column and medial lemniscal pathways of the spinal cord and brainstem, respectively, and is relayed from the thalamus to the cortex via the internal capsule. Electrodes placed anywhere along this pathway, such as over the cervical spine (C2), and at multiple scalp locations, are used to record the volume conducted evoked potentials. Intraoperative electromyography differs from that used in the electrodiagnostic laboratory in several ways. The conventional electrodiagnostic EMG is based on a quantitative and qualitative analysis of motor unit potentials recorded from an insulated, concentric needle electrode inserted into muscle at varying depths with the target muscle at rest, and again in a state of voluntary contraction. Diagnosis of neuromuscular disorders or nerve root injury is then made on the basis of pattern recognition for spontaneous motor unit activity, as well as calculation of duration, amplitude, and shape of the motor unit potential relative to a laboratory norm. While intraoperative spontaneous electromyography also involves the recording of electrical activity produced by skeletal muscle, its purpose is not to diagnose neuromuscular disease/disorder, but to identify acute irritation of a spinal nerve root secondary to mechanical contact, direct traction, heat dispersion from electrocautery, or other such noxious stimuli that trigger nerve root depolarization. The compound muscle action potential elicited by irritation of the innervating nerve root is recorded using short, uninsulated, monopolar needle electrodes inserted subdermally into muscle prior to surgery. No attempt is made to record motor unit force or to assess alterations in spontaneous activity with muscle at rest and under voluntary contraction, as with the awake patient in the electrodiagnostic setting. Although intraoperative monitoring of spontaneous electromyographic activity (spEMG) allows for real-time identification of spinal nerve root irritation from mechanical contact, acute tractional force, or heat dispersion, it is insensitive to nerve root ischemia, sharp dissection, or the application of slow progressive traction. The spine surgeon should be aware that recording of spontaneous EMG activity alone cannot assess the functional integrity of the spinal nerve root. Rather, its primary use is to identify presence or absence of nerve root irritation. Consequently, it may be prudent to augment spEMG with intermittent recording of tceMEPs for surgeries in which individual nerve roots are at particular risk for mechanical or ischemic injury. One of the more time-honored applications of stEMG during lumbar spine surgery is in identification of medial pedicle wall fracture. Compound muscle action potentials triggered at screw stimulation intensities of less than 5 mA correlate highly with presence of medial cortical breach, based on the premise of decreased resistance to the flow of electrical energy to adjacent nerve through the fracture site versus intact bone. stEMG is not sensitive, however, to identifying lateral pedicle wall fracture. It is essential that the surgeon use care when stimulating pedicle screws. Inadvertent stimulator contact with a retractor, surrounding muscle, or blood produces current shunting, thereby raising the possibility of a false threshold reading. Taking a few extra seconds to ensure that the stimulation site is free of blood, that the stimulator is touching only the hexagonal port or head of the pedicle screw, and that neuromonitoring personnel have an opportunity to record a series of responses at progressively increasing intensity levels can make the difference between an accurate assessment of pedicle wall integrity and a false-negative high-threshold reading. In recent years, hydroxyapatite-coated pedicle screws have been introduced as a means of increasing pullout strength in dynamic stabilization systems. Unfortunately, these coated screws have high electrical resistance compared with their conventional non-coated counterparts, resulting in shunting of applied electrical current through adjacent soft tissue rather than through the screw shank. The spine surgeon should be aware that use of hydroxyapatite-coated screws will preclude reliable stEMG testing for presence of medial pedicle wall breach. As with coated screws, questions about the reliability of stEMG also arise when placing percutaneous pedicle screws for lumbar fixation. Here, the use of an insulated sleeve can help isolate surrounding soft tissue from the monopolar stimulator, thereby minimizing the possibility of current shunting. Recently there has been a surge of interest in the minimally invasive direct or extreme lateral trans-psoas lumbar interbody fusion. This lateral approach requires blunt dissection through the psoas muscle and proximate neural elements of the lumbar plexus. Continuous monitoring with stimulated and spontaneous EMG can help identify approximate location and distance from these neural elements, as well as induced nerve irritation, thereby facilitating safe entry to the intervertebral disk space. It is important for the spine surgeon to appreciate that stimulated EMG does not provide assessment of lumbar plexus function and, further, cannot detect microvascular changes that may affect nerve conduction during the trans-psoas approach to the disk space. Because of the significant risks associated with mechanical impingement on the lumbar plexus and nerve roots in these cases, as well as the limited direct visualization of neural elements, it is prudent to augment spEMG and stEMG monitoring with intermittent recording of tceMEPs as a means of assessing the integrity of motor conduction. Figures 67.1 and 67.2 provide supportive evidence for this recommendation by illustrating delayed compromise of nerve root function despite stEMG recordings indicating that nerve root elements were not in immediate proximity to the retractors. Similarly, spEMG did not demonstrate evidence of nerve root irritation as the dilators were passed through psoas muscle and retractors were placed for direct lateral interbody fusion. Despite these negative findings, there was a significant loss of tceMEP amplitude ~ 45 minutes following placement of psoas muscle dilators, most likely due to ischemic changes from progressive nerve compression. This example highlights the value of having an experienced surgical neurophysiologist to guide multimodality intraoperative monitoring and emphasizes limitations of automated monitoring devices marketed by some spinal implant manufacturers. An automated monitoring device would have missed this change, placing the patient at potential risk of an unidentified neural injury. Fig. 67.1 stEMG results showing no evidence of nerve root depolarization following stimulation of retractors during a trans-psoas approach to the L4–L5 disk space.
Intraoperative Neuromonitoring During Spine Surgery
Transcranial Electrical Motor Evoked Potentials
Somatosensory Evoked Potentials
Electromyography
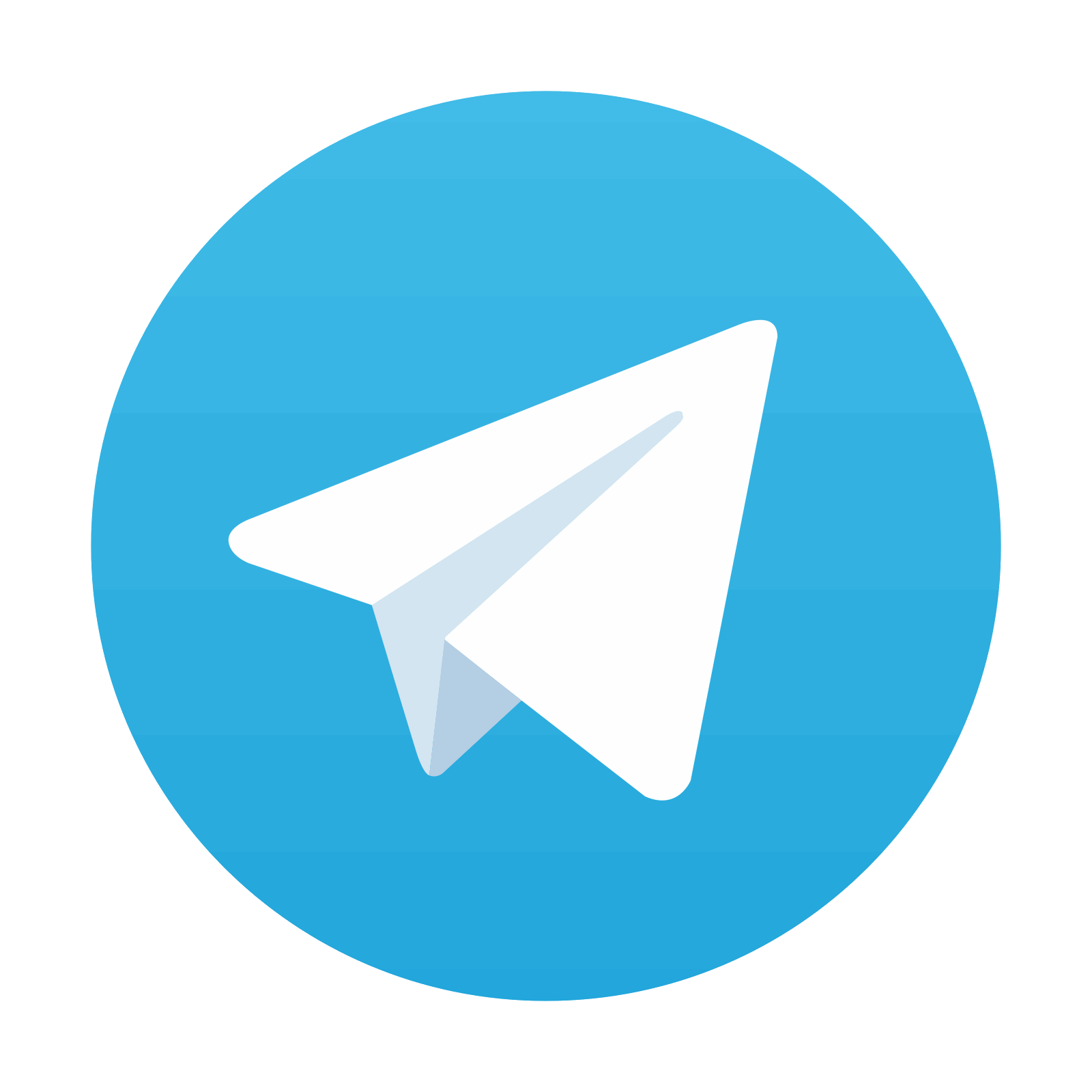
Stay updated, free articles. Join our Telegram channel

Full access? Get Clinical Tree
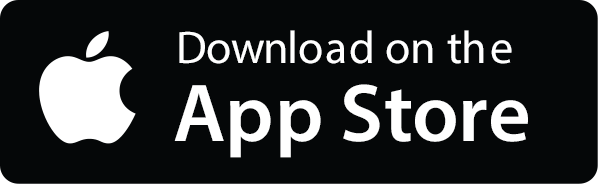
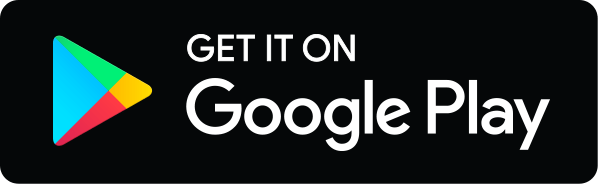