Human Walking
William S. Pease
Brian L. Bowyer
Walking is one of the most basic and ubiquitous forms of human motion. While studying gait, one can simply observe the world in motion. Airports and shopping malls are excellent places to watch walking. Observe the changes that occur based upon each person’s gait speed, height, or age. Compare those who are carrying loads with those who are unfettered. And observe yourself walking in different surface conditions and hills and at different speeds. Which pattern causes fatigue and shortness of breath, and which muscles become fatigued? Building up this personal knowledge base will help the physician to evaluate complaints of walking difficulty and gather information that might clarify the problem.
The basic unit of walking is the gait cycle, which is typically recorded from the time one foot strikes the ground until that episode recurs and starts the next, repeating cycle. During one gait cycle, the body traverses a distance of one stride (Table 5-1). Each stride is made up of one step by each foot, and these two steps are normally symmetrical in length. The frequency of stepping is known as the cadence (steps/min). The speed of walking is calculated as the cadence times the step length. In practice, walking speed is often computed by recording the time needed to traverse a measured distance (e.g., 50-ft walking speed) (1).
The gait cycle is divided into segments (Fig. 5-1) that serve specific functions, each for a limited time during the gait cycle. Each lower limb supports the body during its stance phase and then leaves the floor for its swing phase, during which it advances (or steps). Although the swing phase is the “motion” portion of the gait cycle, one must remember that the horizontal push for the forward motion is the responsibility of the contralateral limb, which is in stance. This forward push requires adequate friction between the foot and the walking surface so that hip extension torques are translated into forward propulsion of the pelvis and swing limb.
NORMAL HUMAN GAIT
The human gait pattern is normally fluid and shows continuous movement in the direction of travel. It has a natural repeating character, with the basic unit of the gait cycle, including one step with each foot. The basic function of walking involves each foot in turn either advancing forward as a step or supporting the body weight and balancing during the advancement of the contralateral lower limb. A period of double-limb stance (DLS) occurs between each step. During DLS, the weight is transferred from one foot to the other in a complex coordinated pattern known as weight acceptance (or loading) and weight release (preswing) for each of the respective limbs (see Fig. 5-1). Although related, the associated actions of arising from sitting and starting and stopping walking will not be addressed in this chapter.
During each gait cycle, a carefully timed pattern of acceleration and deceleration is produced by the muscles. This muscle activity must overcome gravity, as represented by the vertical component of the ground reaction force (GRF), and provide forward propulsion (Figs. 5-2 and 5-3). The entire body is seen to be involved in the gait cycle, with movement occurring simultaneously in each of the three planes of movement, as well as each of the three axes of rotation. These six degrees of freedom of movement need to be considered throughout the gait cycle. For convenience in understanding, the gait cycle is divided into natural subunits called phases that have distinct functions in the gait cycle and have both propulsion and control aspects.
The stance phase of gait begins with the period of weight acceptance. Weight acceptance, also called loading, is a decelerating portion of the gait cycle where the foot must stop after traveling at about 4 m/s during the end of swing phase. This sudden stop requires controlled braking involving simultaneous action of the ankle, knee, and hip. (At the same time, the left leg is involved in the equally complex task of liftoff to initiate its swing phase.) The ankle is in a few degrees of dorsiflexion at heel strike and then rapidly plantar flexes under the control of an eccentric (lengthening) contraction of the anterior tibialis muscle, as well as the lesser dorsiflexors, until the foot is flat on the ground. Simultaneous with this, the knee begins to flex under the eccentric control of the quadriceps (the loading response), and the trunk reaches its lowest point during the cycle. The hip, which was flexed approximately 40 degrees at heel strike, immediately begins to extend on the pelvis as the trunk smoothly continues forward. The forward momentum of the trunk is similarly controlled by contraction of the hip extensors, both the gluteus maximus and the long hamstrings, resulting in controlled hip extension as the trunk moves forward. The hip and pelvis rotate opposite to one another at the same time, the hip rotation of the stance limb being internal. The critical role that the stance limb’s hip extension and internal rotation has on forward propulsion was demonstrated by Sadeghi (2). He also reported that the ankle power is much less important to forward progression or propulsion.
TABLE 5.1 Typical Temporal Gait Parameters for Comfortable Walking on Level Surfaces in Normal Adult Subjects Temporal Gait Parameter Average Value | ||||||||||||||||
---|---|---|---|---|---|---|---|---|---|---|---|---|---|---|---|---|
|
As the left foot leaves the ground, the right hip continues to rotate internally as that limb enters the single-limb support portion of stance phase. The right ankle begins to passively dorsiflex as the tibia tilts forward and the pelvis lowers on the left in the familiar Trendelenburg tilt. The pelvis is also moving laterally to the right so that the center of mass (COM) of the body is aligned over the right foot to allow balance. During this period, the pelvis and COM of the body are rising. To achieve this, some of the kinetic energy of forward motion is converted into potential energy as the trunk rises against the force of gravity (3).
In the middle of single-limb stance (SLS) phase, the ankle reaches maximum dorsiflexion and the heel begins to rise from the floor. This heel rise signals a major shift in the right leg’s function in the gait cycle as it ends its decelerating role and begins to serve as the acceleration for its step phase. The foot now rocks over to the forefoot as the knee extends, and the pelvis (not the hip) maximally rotates externally and the right hip maximally extends in preparation for opposite heel strike. The left lower limb is fully stretched out for its heel strike so that the pelvis is supported and the drop of the COM of the body is minimized.
The weight-release phase on the right must now shift weight support to the left lower limb while accelerating the right leg for its forward propulsion or swing phase. During weight release, the ankle is actively plantar flexed by concentric action of the gastrocnemius, soleus, posterior tibialis, and lesser plantar flexors. These forces contribute primarily to the
vertical components of the GRF. At the same time, right hip flexion is being produced by the iliacus, psoas, and tensor fascia lata muscles, and the left hip internal rotators are causing forward rotation of the right pelvis. These left hip muscles contribute much to the horizontal forces or forward propulsion of the right lower limb. As the toe finally leaves the ground as a result of this combined push and pull on the right lower limb, the swing phase is initiated, causing a step to occur.
vertical components of the GRF. At the same time, right hip flexion is being produced by the iliacus, psoas, and tensor fascia lata muscles, and the left hip internal rotators are causing forward rotation of the right pelvis. These left hip muscles contribute much to the horizontal forces or forward propulsion of the right lower limb. As the toe finally leaves the ground as a result of this combined push and pull on the right lower limb, the swing phase is initiated, causing a step to occur.
During the beginning of swing phase, the right leg continues to accelerate as hip flexion, knee flexion, and ankle dorsiflexion combine to cause the toe to pass over the ground cleanly. Typically, the toe reaches a minimum height of less than 2.5 cm. at the middle of the swing phase. This minimal elevation is energy conserving by reducing the work done to elevate body parts against gravity. This closeness to the ground can be a safety problem, causing stumbles on uneven ground, but the gains of energy efficiency caused by reducing the step height are important enough for this safety risk. The energyconserving nature of the gait cycle, in part attributable to these mechanics and its highly repetitive and symmetrical nature, will be discussed in more detail, but most of us have experienced the rapid fatigue caused by high stepping (“steppage gait”) when traversing deep snow or the lack of repetitive steps when walking on very uneven ground.
The second half of the swing phase returns the right leg into a role of decelerating as the forward motion is slowed in preparation for heel strike. The ankle is held in dorsiflexion while the hip flexes and the knee extends. This combination, along with the forward rotation of the right pelvis and external rotation of the right hip, results in maximal length of the step. Any injury or dysfunction of a joint or muscle-tendon unit that reduces the step length will have a major impact on the efficiency of walking. At the end of swing, the hip extensors serve to brake the forward flexion of the hip; and, at faster gait speeds, the hamstrings slow and control the knee extension. The speed of the leg and foot must be controlled to prevent slipping at heel strike.
ENERGY CONSERVATION AND GAIT
Energy is consumed in three different categories during walking (4). First, there is the work of moving the body’s mass through the required distance in a period of time. Second, there is the work done to accomplish the up-and-down motion of the trunk for each step as the pelvis rises to a position above a single supporting leg during midstance and is lowered during double support when it lies between the two lower limbs supporting it at opposite angles. Third, energy is being consumed by the body for general, or basal, metabolism. Because work requires energy and faster walking involves more work, there is an energy cost. However, since the constant basal metabolic rate continues, as well as some muscular energy consumption for standing, the total energy consumed per unit distance traveled is seen to decrease initially as speed increases from a very slow walking speed. As an optimal speed is reached that is traditionally called comfortable walking, the combined metabolic rate is most efficient for traveling over the ground. The higher energy requirements of faster walking and running require greater work output by the muscles.
The vertical displacement of the body is minimized by a number of factors that are known as the determinants of gait (5). These six determinants operate independently but simultaneously to produce a smooth sinusoidal vertical and horizontal path, which has one vertical peak and trough for each step. There is also one lateral sinusoid, or curve, for each stride as the body moves toward the supporting foot during each cycle. To illustrate the effects of the determinants of gait, they are removed and replaced in models of the gait cycle as shown in Figures 5-4, 5-5, 5-6, 5-7 and 5-8. This “compass gait” analysis was restudied by Della Croce et al. (6) with the inclusion of data recorded in a contemporary gait lab, largely reaffirming the model.
The first determinant of gait is the rotation of the pelvis (Figs. 5-4 and 5-5). During each step, the pelvis rotates forward on the side of the swinging limb. The axis of this rotation
is the hip joint of the stance leg, which undergoes internal rotation. As the pelvis forms a bridge between the two hips, it reduces the angle of intersection of the thighs to reduce the vertical descent of the trunk.
is the hip joint of the stance leg, which undergoes internal rotation. As the pelvis forms a bridge between the two hips, it reduces the angle of intersection of the thighs to reduce the vertical descent of the trunk.
The pelvic Trendelenburg motion, or pelvic list, is the second determinant (Fig. 5-6). The pelvis drops a few degrees so that the hip of the leg in swing phase is lower than the hip of the stance limb. This reduces the vertical rise of the COM of the trunk and reduces the work of lifting this mass. The cost of this pelvic list is to reduce the space for toe clearance, but this is an affordable cost. Pelvic list, as well as rotation, has been shown to decrease at slower speeds (7). This fact, and the data that reveal that these motions are less important modifiers of the vertical movement of the COM (6), suggests that these movements are important to the control of the momentum during forward propulsion.
Knee flexion in stance phase is an important determinant of gait for two reasons. First, it provides a shock-absorbing mechanism at the beginning of the stance phase. The reduction of the shock of foot impact on the floor helps to maintain momentum and thereby reduces energy loss of stopping and restarting the gait cycle. Also, the knee flexion in stance reduces the height of the hip joint in midstance. This additional height reduction prevents energy loss from lifting the body but at the cost of quadriceps muscle work.
Lateral displacement of the pelvis also occurs during each step (Fig. 5-7). The pelvis and trunk must move to the stance side to balance the COM of the trunk above the stance foot. This also aligns the tibia into the vertical position during stance. This determinant of gait is a net loss of energy since it causes upward movement of the body, but it is necessary for balance in bipedal gait.
The trunk and shoulder rotate during normal gait in a direction opposite to the pelvic rotation. This 180-degree phase shift of total trunk movement balances the angular acceleration so that balance and forward momentum are maintained. Smooth, coordinated movement here is an energy advantage.
The obliquity of the subtalar joint provides a unique relationship between the motion of the foot and that of the shank. Dorsiflexion of the foot causes lateral movement of the forefoot and vice versa. During stance, the passive dorsiflexion of the ankle, therefore, causes internal rotation of the tibia to partially match that similar movement in the hip. This combined rotation is then reversed during the end of stance or weight release.
Sagittal plane foot and ankle movement is referred to as the three “rockers.” The three components are at heel strike, during foot flat, and during toe-off. Each of these has work- and energy-saving factors (Fig. 5-8). For the first rocker, the dorsiflexion of the foot causes the heel to stick out and produce a net lengthening of leg length to maximize the length of the step. This extra length is quickly lost during weight acceptance, when it is no longer needed. Also during this time, the resisting ankle dorsiflexion muscles provide a shock-absorbing descent of the forefoot. During the second rocker, dorsiflexion of the foot occurs during midstance. This serves to reduce the length of the leg until the pelvis passes in front of the ankle. As the heel rises after midstance, the third rocker occurs. The elevation of the heel increases the leg length during push-off and so limits the amount of drop that is experienced by the pelvis. The rockers of the heel, midfoot and forefoot are therefore useful in minimizing the vertical work of the body movement. The second utility of the rockers
is to provide a rolling-like mechanism of foot during stance so that momentum is preserved. Della Croce’s work suggests that heel rise and forefoot support may be the most important determinant of gait (6).
is to provide a rolling-like mechanism of foot during stance so that momentum is preserved. Della Croce’s work suggests that heel rise and forefoot support may be the most important determinant of gait (6).
KINETICS
A complete understanding of gait requires knowledge of the kinetics of movement in addition to the kinematics of motion discussed in the preceding sections. Kinetics is the science of forces acting on bodies to cause motion. Gait kinetics explains the causes of the motions of gait.
The basic principles of kinetics are Newton’s three laws of motion. The first is that a body (of mass m) will change velocity (accelerate (a) or decelerate) only if a force is applied to it. Also, this change in velocity (v) is proportional to the force (F = ma and a = Δv). Newton’s third law, the law of action and reaction, is very important for the study of gait and other aspects of biomechanics. This law relates the forces interacting between the foot and the floor as always being equal and opposite. One can therefore measure the forces of ground reaction on the foot with a force plate and begin to understand the net forces acting on the lower limb and on the body as a whole (Fig. 5-9).
The GRF is represented by three directions perpendicular to each other: horizontal (fore/aft), side to side, and vertical. One can also measure the twisting or rotational load on the force plate as the rotating limb is constrained from moving by friction. The force plate also allows the direct calculation of the center of pressure on the foot. During stance phase, the center of pressure starts at the medial heel. As foot flat occurs and the limb progresses to single stance, the center of pressure typically moves laterally as it progresses forward. Then, after heel rise and into weight release, the center is in the forefoot, progressing to the medial side again.
From the center of pressure, the net vector of the three GRFs can be located at each instant during gait (see Fig. 5-3). This force vector (i.e., a force that has magnitude and direction) acts on each of the limb segments as well as each joint. The perpendicular distance between the GRF and each joint defines a moment arm, or leverage, that when multiplied by the force creates a moment of rotation, or torque, about the joint’s axis.
Through the process known as inverse dynamics, these forces can be calculated sequentially from the ground and foot up to the trunk. Changes in vertical position of the trunk COM result in changes in the potential energy, and forces
causing changes in velocity of limb segments will affect the kinetic energy. Only small amounts of energy change are seen in the transverse and frontal planes, and so studies of gait usually focus on only the energy changes in the sagittal plane relating to forward progression and vertical support.
causing changes in velocity of limb segments will affect the kinetic energy. Only small amounts of energy change are seen in the transverse and frontal planes, and so studies of gait usually focus on only the energy changes in the sagittal plane relating to forward progression and vertical support.
Power is defined as work per unit time. The net power of each joint is shown in Figure 5-10. Keep in mind that this net power is not the same as the individual muscle power or the metabolic power representing those changes in energy levels. It is only the balanced result of those interactions with the GRF. This net power at each joint can represent generation of energy (+) or absorption of energy (−). For example, study the knee power in Figure 5-10. The power is initially negative (decelerating) as the extensor torque produced by the quadriceps is overcome by the flexion moment of the ground reaction. As the trunk progresses forward, the GRF vector angles forward and reduces the flexion moment as the knee stabilizes. Later, the extensor muscles and GRF produce extension together and the net power is positive (accelerating).
ELECTROMYOGRAPHY IN GAIT ANALYSIS
Actively contracting muscles under neural control produce electromyographic (EMG) activity. This EMG signal is easily recorded and amplified so that the muscle activity can be correlated with the kinematics and kinetics of gait. In carefully controlled experiments of isometric contraction, a linear relationship exists between the EMG signal and the muscle force. However, this relationship is greatly disrupted by the movement of the muscles during gait, so EMG does not give accurate information about muscle forces (8). For example, EMG analysis of the rectus femoris’ role as a hip flexor in early swing phase demonstrates that higher amplitude EMG signals are produced at faster walking speeds (9). The muscle must exert greater force to flex the hip more rapidly, but the relationship is nonlinear and complex, and one is still unable to make quantitative conclusions about muscle force from EMG signals alone.
Multichannel amplifiers are used in gait laboratories to record from several muscles simultaneous to the recording of kinematic motion (Fig. 5-11). The electrodes used for this recording may be either surface electrodes or flexible intramuscular (IM) wire electrodes (8). Surface electrodes are noninvasive and record a larger volume of the target muscle. They are unable to record signals from deep muscles and may record unwanted signals from muscles that are adjacent to the target muscle. Wire electrodes can be located to record more precisely from any muscle, including those that are deep. However, in addition to being more painful, the wire electrodes may dislodge or break during activity. EMG also allows the measurement of muscle fatigue during sustained activity. As lactic acid accumulates in a muscle with exercise, the membrane propagation of the action potential (AP) is slowed. This slowing of velocity is seen as a reduction in the frequency of the recorded EMG signals (8).
DEVELOPMENT OF GAIT
Normal adults perform the task of walking without significant active thought or effort. It is a process that is learned and eventually mastered in childhood. Understanding the development of gait is critical to any physician performing neuromuscular examinations of children. To appreciate subtle normal variations or pathologic findings, a physician must have a thorough knowledge of normal development, understand its relationship to gait, and use a systematic approach (10).
Early Infant Development
The first few years of life are characterized by the most rapid growth in the human life span (11). During this period of maturation of the central and peripheral nervous systems, the development of gait emerges according to a typical pattern of motor development: rostral to caudal, proximal to distal, and mass movement to specific action (11, 12). Although there is variation in the development of each child, some general statements concerning milestones of development are appropriate. Head control is a prerequisite to ambulation that begins to improve by the age of 2 months (12, 13). Further improvement in head control is seen at the age of 3 months, along with an integration of primitive reflexes with occasional bobbing during supported sitting (11). From the ages of 4 to 6 months, children learn prone propping followed by rolling. Rolling is first achieved from prone to supine, followed by supine to prone, and by this time, independent sitting is usually achieved with arm support. By 9 months, a significant increase in mobility ensues with the advent of belly crawling and standing up by pushing onto hands and knees (11).
![]() FIGURE 5-10. Kinetics and kinematics at the hip, knee, and ankle. Sagittal joint motion, moments, and power are shown. (Courtesy of D. Casey Kerrigan, MD, with permission.) |
![]() FIGURE 5-11. General muscle group activity as a percentage of the gait cycle in normals. (Courtesy of D. Casey Kerrigan, MD, with permission.) |
Achievements of these skills as well as sitting, creeping, and pulling to a stand are prerequisites that a child must master before supported and independent walking. Balance and support, two major skills required for independent walking (14), are practiced as a child progresses toward supported walking.
Supported Walking
Around 8 to 10 months of age, children begin to walk by holding onto objects. For example, a child may grab a crib surface or table while attempting to maneuver across a room. On the average, supported walking or cruising lasts 2 months, with significant variability (15). This stage is characterized by the use of arms for support and is a transitional phase that precedes independent walking. In comparison to independent walking, supported walking is characterized by a longer gait cycle time, longer stance phase, increased variability in successive strides, and decreased walking speed. The average walking speed in supported walking is noted to be 39 cm/s, with stride length of 44 cm and 65% of gait cycle being spent in the stance phase (16).
When compared with normal adults, children reveal greater angles of hip flexion during walking. The hip extension pattern shows increased variability, poor smoothness, and decreased total amplitude. There are also striking differences in knee and ankle patterns between normal adults and children during supported walking (16). Knee pattern and amplitude of flexion differences are most impressive during the stance phase. At initial floor contact, Statham and Murray (16) found average knee flexion of children in supported walking (stance) to be 21 degrees, which is in sharp contrast to that of adults where knee flexion was 1 degree. Knee flexion angles during swing were similar to that of adults.
In supported walking, there is no clear heel strike, as a child may make initial floor contact with the heel, flat foot, or forefoot (i.e., toe walking) (16, 17, 18). This is in contrast to normal adults, where heel strike is very consistent and is followed by ankle plantar flexion rather than ankle dorsiflexion. The lack of full knee extension and ankle dorsiflexion at the endof-swing phase have been cited as possible causes for variability at foot strike (15). Initial floor contact is followed by ankle dorsiflexion (modified loading response) in supported walking regardless of which part of the foot makes initial contact. The maximums of ankle dorsiflexion and plantar flexion angles, the degree of ankle dorsiflexion at initial floor contact, and the maximum ankle dorsiflexion during swing phase are also greater in supported walking than they are in adults (16).
In summary, prominent features of supported walking in children that are in contrast to those of adults include increased hip flexion, decreased knee extension, increased ankle dorsiflexion, decreased walking speed, increased variability, and decreased smoothness.
Independent Walking
Following supported walking, children progress to independent walking with a premature gait pattern and, during this phase, are referred to as “toddlers” (15). This is a transitional period between supported walking and a mature gait pattern. Collection of data using quantitative gait analysis in this age group often proves to be very difficult, as these children do not follow simple instructions and display significant variability from one gait cycle to the next. Inconsistent gait pattern, wide-based support, increased hip flexion, and lack of reciprocal arm swing are some of the hallmarks of gait in toddlers. In general, the development of walking skills is completed by the age of 5 years (19, 20).
In the progression from supported walking to independent walking, there is a trend toward decreasing excessive hip flexion and greater extension at initial floor contact; however, these movements are still not identical to those of adults (16). On initial floor contact, the knee in independent walkers is held in less flexion, and there is the emergence of an adultlike knee flexion during loading. This knee flexion is established in most children by the age of 2 years (21
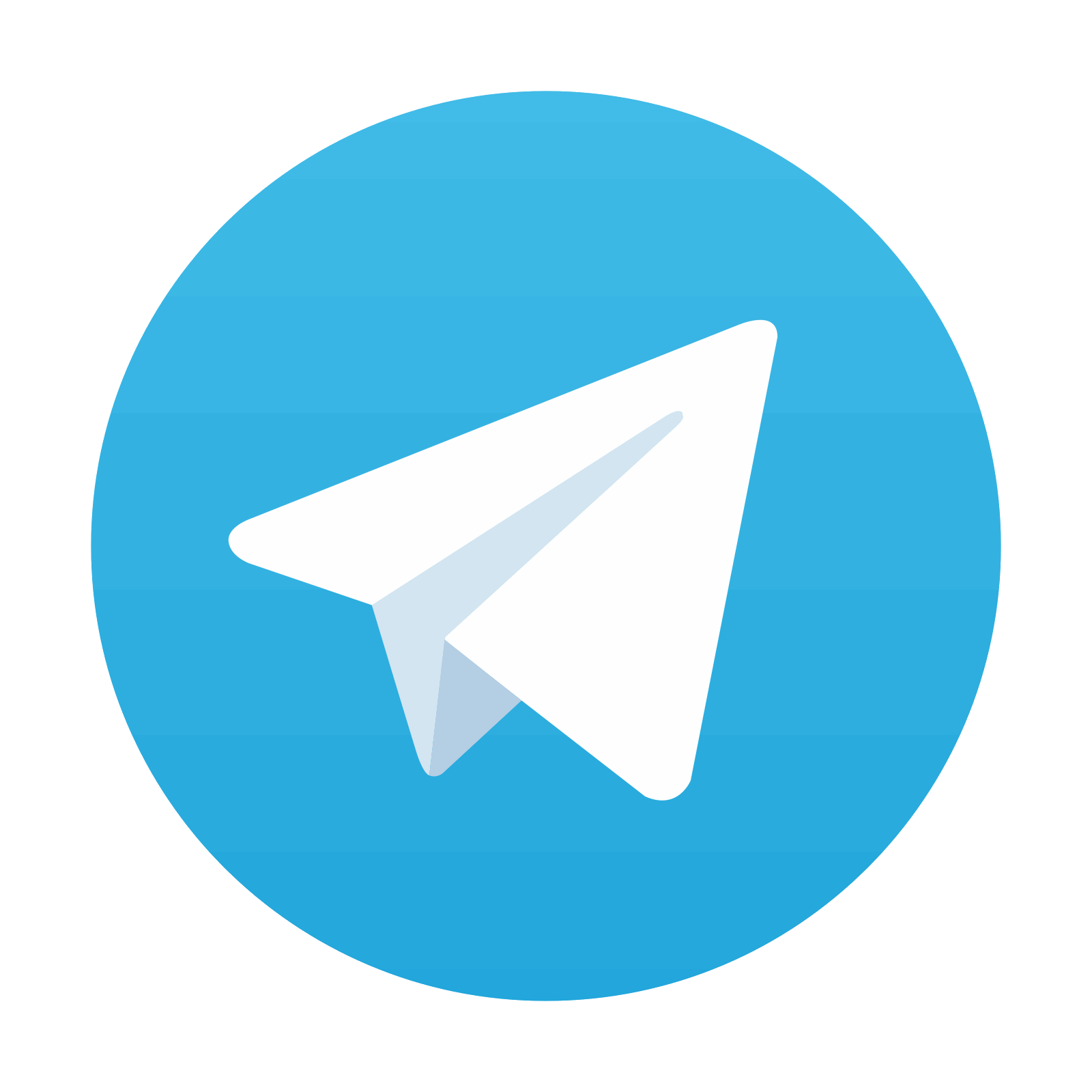
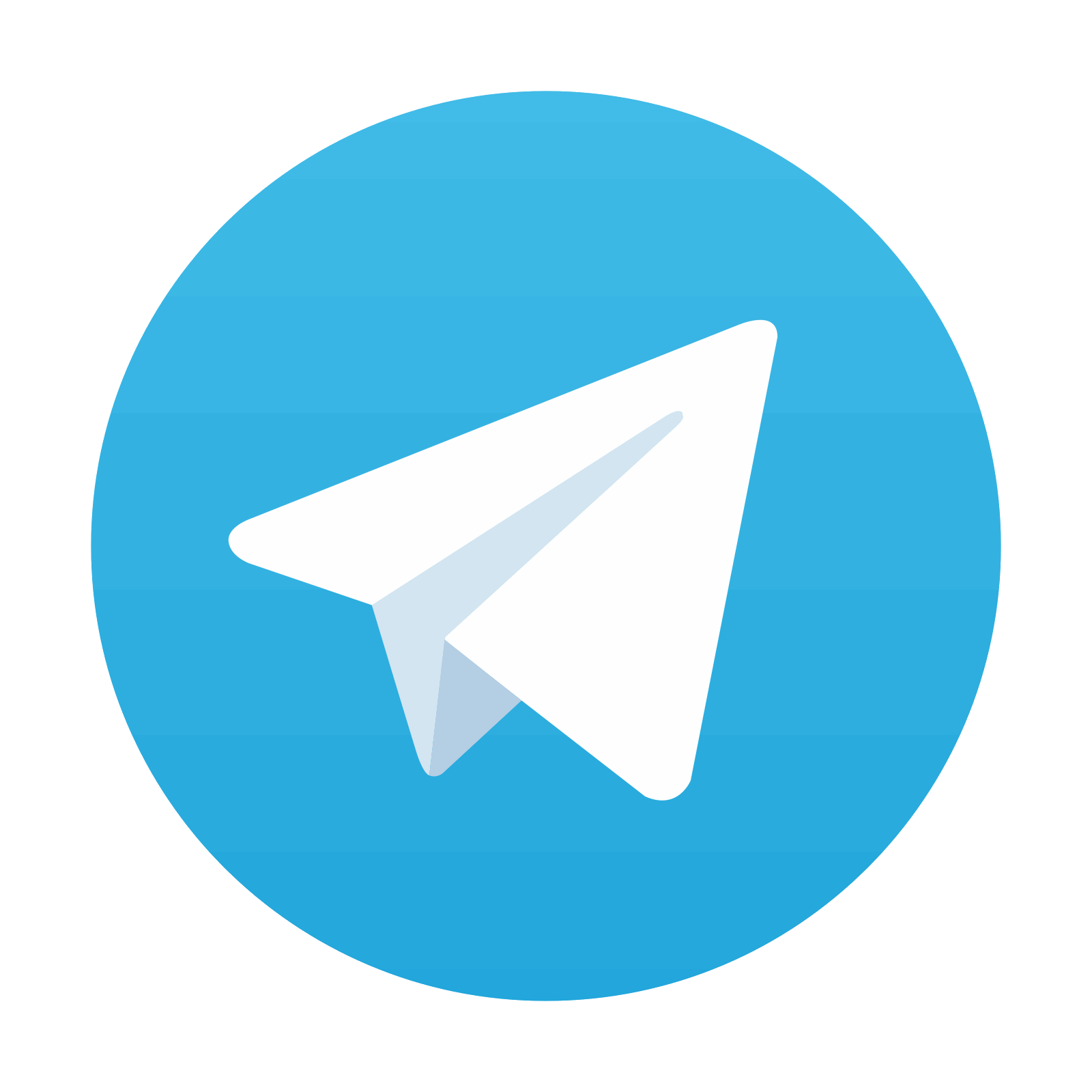
Stay updated, free articles. Join our Telegram channel

Full access? Get Clinical Tree
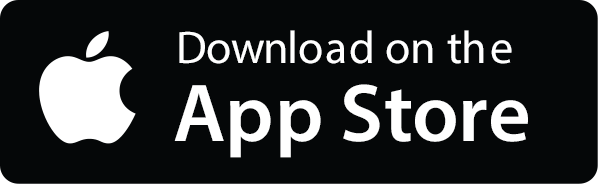
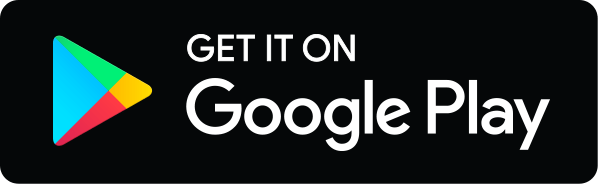
