Fig. 1
The applications of image fusion (marked with *) in the workflow of CATS
CT image is the commonest imaging modality used for image-guided computer navigation in bone tumor surgery [7, 8, 10, 14] though MR-based computer navigation was also reported [16]. CT-based navigation can provide a reliable image-to-patient registration [10, 11, 14] that is a prerequisite for any accurate image-guided navigational procedures. Also, bone information can be easily extracted from CT image dataset that is essential for custom-made computer-aided design (CAD) implants and allograft selection in bony reconstruction after tumor resections. Therefore, CT images are normally used as the base layer of imaging on which other image modality (MRI/PET) or CAD data (prosthesis/allograft) are overlaid.
2.1 CT/MR Image Fusion for Surgical Planning
In the fusion of two or more imaging modalities, the image co-registration can be performed by “rigid” or “non-rigid” registration. As bone structures are not shifted or deformed by surgical manipulation as for abdominal soft tissue organs, rigid registration of imaging modalities is sufficiently accurate and the most ideal for surgical and interventional procedures for bone tumors. The technique of image fusion for CATS was described in 2008 [15]. Overlaying MRI over CT images with the same spatial coordinates generates fusion images (Fig. 2a–d). The multimodal images can be overlaid automatically in the navigation software (OrthoMap 3D module, version 2.0, Stryker, Hong Kong) that is the only commercially available navigation software dedicated to bone tumor surgery. The process can also be manually adjusted as different imaging datasets may be acquired at different scanning positions. The MR image dataset can be shifted manually to match the corresponding axial, coronal and sagittal views with that of CT images. The fusion image is considered to be acceptable for subsequent navigation planning if the bony contours on the CT/MR images at the region of interest matched within a 1-mm margin of error as visually assessed by surgeons. In general, the process of image takes less than few minutes and will improve with practice. Tumor extent is outlined from MRI which best shows the pathological details of bone tumors. The tumor edge is determined by looking at the transition of marrow signal from abnormal to normal in T1-weighted MR images. A 3D bone model is also generated by adjusting the contrast level of CT images. The tumor volume segmented from MRI and the CT-reconstructed bone model are combined to create a 3D bone–tumor model (Fig. 3a–c). Surgeons can then scrutinize all the fused image data sets in three spatial dimensions and the 3D model concurrently on one screen of computer navigational display. Another dimension of image analysis is possible by continuous blending between different proportions of CT and MR images (Fig. 2a–c). The image fusion is not restricted to plain CT and MRI. Additional information on vascular anatomy from CT angiography or tumor metabolic activities from PET scan can also be integrated into the surgical planning via image fusion technique. The PET fusion has the additional advantage to distinguish tumor from scar tissue in recurrent tumor patient with prior surgery or radiotherapy [15]. However, there is no report investigating the role of image fusion in diagnosis or assessment in orthopaedic bone tumors. This new interactive way of image analysis allows surgeons to obtain a better mental picture of tumor’s location and regional anatomy. The best surgical access can be planned. Vital structures in relation to tumor location can be visualized. Resection levels and planes can be precisely defined and then executed via intraoperative image-guided computer navigation.
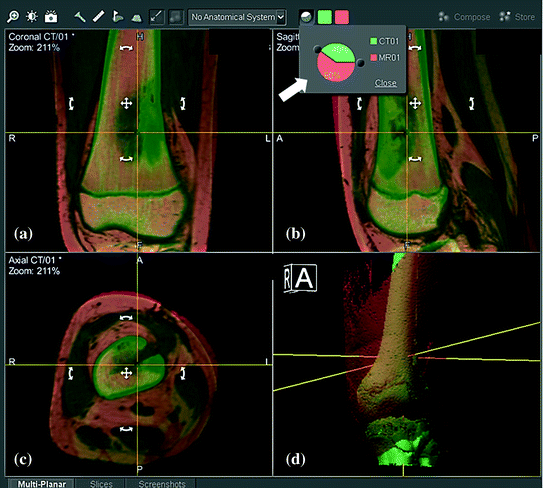
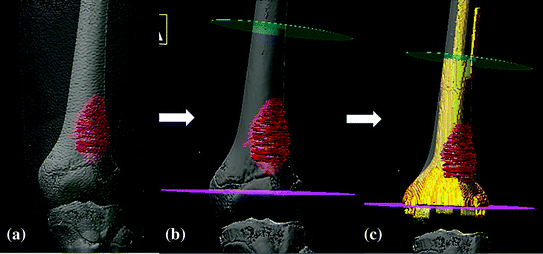
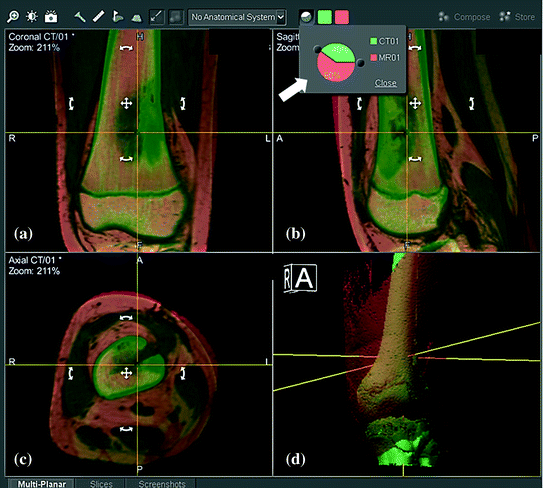
Fig. 2
CT/MR fusion images are shown in the navigation display in a 11-year-old patient with osteosarcoma at left distal femur metaphysis. MR images (orange) are overlaid and realigned on CT images (green) so that the region of interest (femur bone) has the same spatial coordinates on both images. The process can be done automatically or manually (by moving MR images with cross or curved arrows in white). The accuracy of image fusion is verified in reformatted coronal (a) and sagittal (b), axial (c) views and 3D bone models (d) of both images. The fusion is acceptable only when the femur bone surfaces of both images exactly coincided. Different proportions of individual image dataset can be blended (big white arrow) to examine the characteristic features of each image dataset (Color figure online)
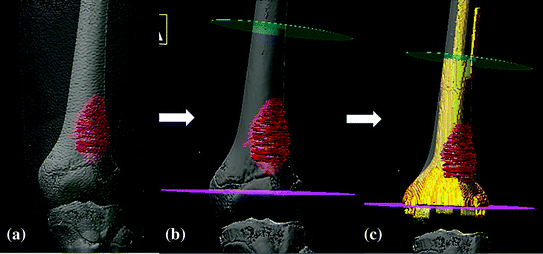
Fig. 3
3D models of the patient with left distal femur osteosarcoma. After fusing CT/MR images and outlining the tumor extent from MRI, a 3D model that includes skin, femur bone and tumor a is generated for resection planning. b The intended resection planes of joint-preserving tumor surgery (pink and green) can be defined precisely in the navigation software after examining all the reformatted views of the fused images and 3D models. c A CAD prosthesis is designed that matches with the bone defect and the remaining distal femur epiphysis. It is performed by integrating the data of CAD prosthesis into the navigation planning by image fusion technique (Color figure online)
Wong et al. [15, 17] reported the results of image fusion in 22 cases with CATS. The results showed all tumor resections could be carried out as planned under navigation guidance. Navigation software enabled surgeons to examine all fused image datasets (CT/MRI) together in reformatted 2D images and 3D models. It enabled surgeons to understand the tumor extent and its anatomical relationship with nearby structures. The mean time for preoperative navigation planning was 1.85 h (1–3.8). Intraoperatively, image guidance with fusion images could provide precise orientation of surgical anatomy, easy identification of tumor extent, neural structures and intended resection planes in all cases. The bone resection could be precisely implemented in terms of the resection level and orientation, according to the pre-defined tumor volume and data of custom prosthesis.
2.2 Integrating Virtual CAD Planning into CATS
Surgical planning in orthopaedic bone tumors require precise definition of bone resections with planes that are away from the tumor edge and also in correct orientation to accommodate a custom implant if it is chosen for bony reconstruction. Virtual planning with manipulation of the resection bone models, incorporation of CAD implant models or allograft bone models for reconstruction are not possible in surgical navigation systems. They only accept medical imaging data in Digital Imaging and Communications in Medicine (DICOM) format and do not offer complex surgical simulation on these data. They offer relatively simple planning and serve more as intraoperative guidance tools. On the other hand, CAD engineering software allows surgical simulation with complex tumor resections with preoperative image datasets, design of custom tumor prostheses and selection of bone allografts from a 3D digital bone bank [18]. However, the planning in its proprietary format of the engineering software is incompatible for direct use in surgical navigation system. Therefore, successful implementation of this virtual surgical plan at OR still relies on the experience of surgeons if no intraoperative guiding tools are available. The difficulty increases with case complexity. The technique of integrating virtual CAD planning into CATS was first described in 2010 [19]. For surgical planning of musculoskeletal tumors, the virtual surgical plan datasets contain the original CT images, intended resection planes and CAD custom prostheses. This modified CT datasets in CAD format are back converted and exported in DICOM format. The virtual surgical plan datasets are then imported into the navigation machine and co-registered with the original CT datasets that will be used for navigation-guided surgery. The exact locations of intended resection and custom CAD implants that are obtained from the engineering software can be accurately transferred to the navigation planning (Fig. 4a–d). Therefore, this type of image fusion can enhance the capacity of surgical navigation and may enable surgeons to precisely execute complex virtual surgical simulation with any CT-based surgical navigation system at OR.
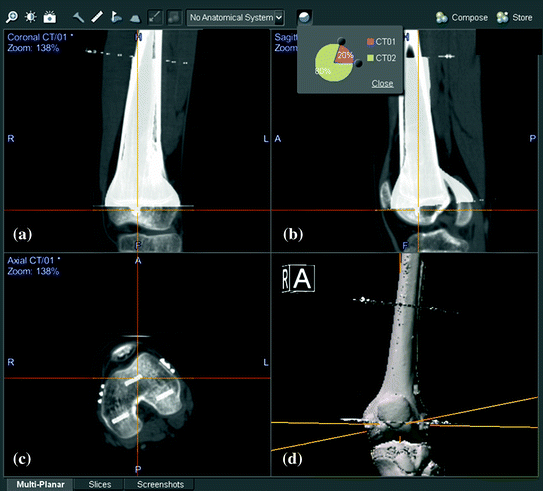
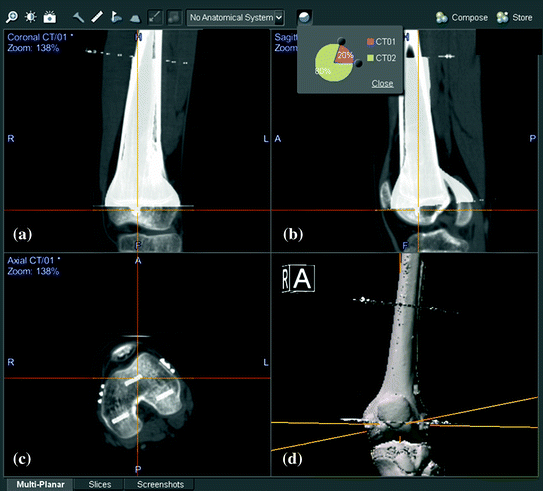
Fig. 4
shows the integration of virtual surgical planning into navigation planning by image fusion technique in the patient with left distal femur osteosarcoma. Virtual planning with femur bone resection and reconstruction with a CAD prosthesis was first performed in the CT image datasets in a medical engineering software. The virtual planning was exported in DICOM format and then imported into the navigation system. This modified image datasets (CT02) that contain the resection planes and the CAD prosthesis was fused with original CT image datasets (CT01). Reformatted coronal (a) and sagittal (b), axial (c) views and 3D bone models (d) of both image datasets shows the femur bones of both CT01 and CT02 image datasets are aligned with same spatial coordinates. This technique of CAD to DICOM conversion of virtual surgical planning and image fusion with modified CT datasets enables accurate translation of virtual CAD planning to subsequent navigation-assisted tumor surgery
Image fusion technique has also been used in selection of the most optimal bone allograft for reconstruction of a massive bone defect after tumor resection [18]. CT scan of both patients’ bones and allograft are performed. The 3D bone model of the patient is then matched with that of allograft in digital bone bank by 3D image registration in an engineering software. This facilitates and expedites in finding a suitable allograft that matches with the planned skeletal defect after bone tumor resection. The same intended resection for bone tumors in the navigation planning can be transferred to the matched allograft when the CT images of the allograft are imported and fused with the CT images of bone tumors. Same image-guided navigation resection can be performed on both the bone tumor and allograft at OR. Better matching of the allograft to the resection defect will improve host-allograft contact, with less chance of nonunion.
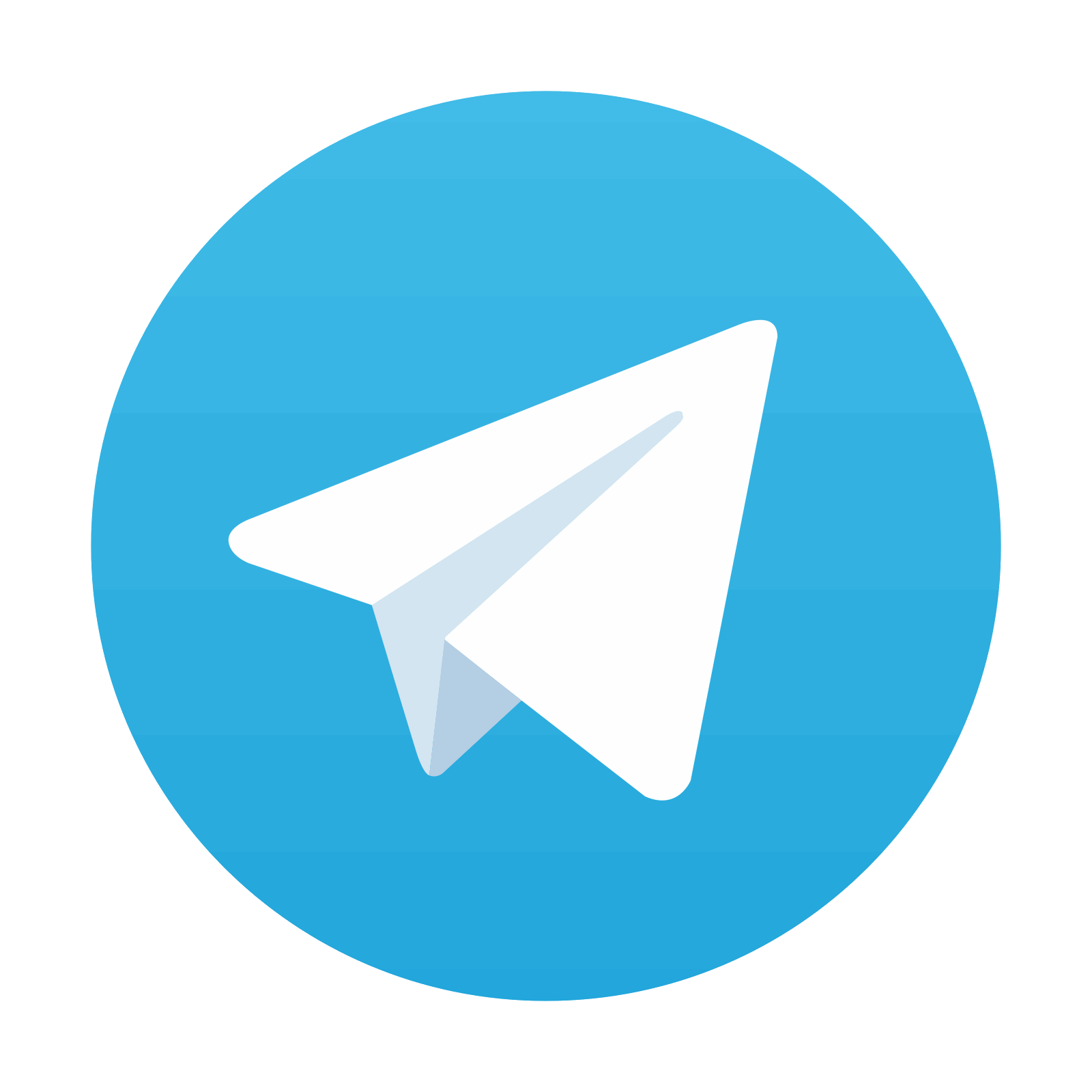
Stay updated, free articles. Join our Telegram channel

Full access? Get Clinical Tree
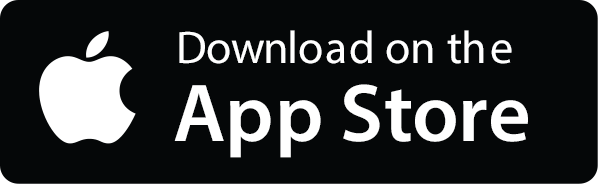
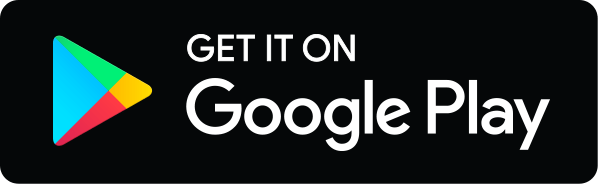