Fig. 1
Medical robots for musculoskeletal surgery
Major clinical application of robotic systems is arthroplasty. Now, there are two commercially available robotic systems; one is ROBODOC (Curexo Thechnology Corp., Fremont, California) and the other is RIO (MAKO Surgical Corop., FortLauderdale, Florida). Other application is a guidance system for screw insertion to spine. Renaissance (MAZOR Robotics Inc., Orlando, Florida) is the only commercial product in this field. Several robotic systems have been developed for trauma surgery though they are not commercially available until now. Next section, we briefly review three commercialized robotic systems. And we will treat safety issues related to robot-assisted fracture reduction with the fracture reduction robot. The developing process and strategy will be introduced with the fracture surgery robotic system that provides total solution for fracture surgery. Finally, MoebiusTM robotic system that is multi-purpose bone surgical robot will be introduced.
1.1 ROBODOC
The development of the ROBODOC was started from two doctors’ idea, which was to make a precise cavity in a femur for an accurate positioning of artificial hip implant and restore a proper joint biomechanics. In 1986, they cooperated with IBM research center, and IMB funded a startup company, ISS (Integrated Surgical Systems) to commercialize the idea. ISS had developed ROBODOC by customizing an industrial robot of NIDEC SANKYO Inc. and firstly tried to use ROBODOC to human surgery under FDA approval of feasibility study in 1992. ROBODOC was firstly sold in Europe at 1996 while the FDA approval was pending. ISS was taken over by CUREXO Inc. at 2006, and then clinical trial in USA was completed with a subsidiary of CUREXO. ROBODOC was finally approved for commercial sale under a 510(k) notification by FDA at 2008.
A surgical planning procedure using 3D CT data is required before surgery. If ROBODOC is connected to patient’s bone and a registration procedure was finished, it automatically milling the bone for exact contact with implants.
Bach et al. reported that ROBODOC surgery did not impair hip abductor function in spite of a wider exposure of the proximal femur, a rigid fixation of leg from comparing gait of patients after ROBODOC and conventional total hip arthroplasty [3]. Honl et al. [15] reported that the robotic assisted technology had advantage in accuracy from a prospective study, but there are disadvantage such as high revision rate, the amount of muscle damage, and longer surgical time.
Nishihara et al. evaluated the clinical accuracy of femoral canal preparation using 75 consecutive total hip arthroplasties performed with ROBODOC system. They compared the preoperative planning with the postoperative CT data at one month after surgery. Results show a high degree of accuracy with less than 5 % in canal fill, less than 1 mm in gap, and less than 1 degree in alignment [35]. They also reported that in the robotic milling group does not occur intraoperative femoral fracture and this group shows a radiographically superior implant fit, and shows significant superior Merle D’ Aubigne hip score at two year after surgery [34]. As the comparison study of Nakamura et al., robotic-milling shows slightly better clinical scores until 3 years after surgery. This difference was no longer present at 5 years after surgery, but robotic milling groups showed less variance in limb-length inequality and less stress shielding of the proximal femur [33].
Schulz et al. reported that the results of total hip arthroplasty of 97 hips with ROBODOC were equaled compared to a manual technique. However, they found technical complications directly related to the robotic device in nine cases, such as fine halted milling process, two femoral shaft fissures, one damage to the rim of the acetabulum, and one defect at the greater trochanter [39].
The benefits of ROBODOC surgery for total hip arthroplasty are still controversial. The robotic assisted milling of a bone certainly improved accuracy, and surgical outcome were equaled compared to a conventional technique. However, the robot-assisted surgery has shown clinical and technical complications.
1.2 RIO robotic arm
Another approach from an automatic robotic system like ROBODOC for robotic arthroplasty is semi-active, and this method is adopted by Arcrobot Company Ltd., and MAKO Surgical Corp. MAKO was relatively recently founded in 2004 than Acrobot that was founded in 1999. Though their robotic systems use active constraint, which gives surgeon’s hand resistance force for keeping the safety zone during knee arthroplasty, MAKO’s robotic system does need rigid fixation to bony structure. MAKO recently acquired Acrobot as a settlement in an intellectual property litigation [37]. MAKO got the FDA clearance of RIO Robotic Arm Interactive Orthopedic System in 2008, and is merged with Stryker Medical in 2013.
Lonner et al. [25] reported that the robotic arm-assisted unicompartmental knee arthroplasty (UKA) shows more accurate and less variable in initial results from comparing 37 UKA using robotic arm-assisted bone preparation with 27 UKA using conventional technique. Pearle et al. [36] also agreed that haptic guidance in combination with a navigation module allows for precise planning and execution in 10 patients with UKA.
Citak et al. [7] evaluated whether the robotic system with dynamic bone tracking that was newly updated function would provide more accurate implant from six fresh-frozen cadaver studies (six knees with robotic UKK and six knees with conventional method). Robotic UKA showed the decreased RMS error in both position and orientation for the tibial and femoral components.
1.3 Renaissance
Mazor robotics Inc. was founded at 2001 based on research of Israel Institute of Technology. Their early product, SpineAssist that was a mechanical guidance system for spine surgery, got an approval from FDA at 2004, and the company commercially released new product, Renaissance guidance System in 2011.
Renaissance can improve the insertion accuracy of pedicle screws using a hexapod-type robot that guides mechanically an entry point of the screw. Surgical procedure is quite simple; this is plan, mount, registration, and operation. Surgeon plans for the ideal surgery with 3D images before surgery. In operation, surgeon rigidly attaches a guide rail to patient, and then takes two fluoroscopic images to registration between patient and CT data. If Renaissance is positioned at indicated location on the rail, it starts guiding tools and implants to the planned position.
From a prospective randomized comparison between a robot-assisted placement of lumbar and sacral pedicle screws and a conventional freehand screw implantation, Ringel et al. [38] reported the conventional method was more accurate than the robot-assisted method, and radiation exposure was equaled. But, they thought the modification of the robotic system would increase accuracy of screw position.
In recent study, Dravel et al. [8] reported the use of the robotic system enables minimally invasive, percutaneous transpedicular interventions with safety and a high accuracy of screw placement. The adequacy of quality control of robot-assisted pedicle screw fixation accuracy was also reported from a cumulative summation test [20].
2 Robot Assisted Fracture Surgery
2.1 Minimally Invasive Fracture Surgery
The principles of fracture surgery are accurate alignment of bone fragments, rigid fixation, avoidance of soft tissue injury, and early recovery. Generally, it has priority to restore a bony structure between a proximal bone fragment and a distal bone fragment than to fit all pieces of bone fragment of diaphysis. A repositioning to normal anatomical structure is important in upper limbs fracture, whereas a restoration of mechanical axis is emphasized in lower limbs fracture. Bone fragments should be rigidly fixed not to make movement at the fracture site.
There are two main methods for fixation of bone fragments; a closed intramedullary nailing and a plate osteosynthesis. The closed intramedullary nailing is now considered as a standard treatment for a long bone shaft fracture. The plate osteosynthesis has showed particularly advantageous when an intramedullary nail may be technically not feasible.
Lee et al. reported that MIPO (Minimally Invasive Plate Osteosynthesis) had the shorter bony union time and the longer operation time than interlocking intramedullary nailing. Two groups did not show statistical significance in clinical results [24]. Apivatthakakul et al. [2] reported that although the biomechanics of the plate fixation are less stable compared to the intramedullary nail, the mechanical stability is stable enough for bone healing. Guo et al. [13] conclude that both an intramedullary nailing and a percutaneous locked compression plate can be used safely to treat distal metaphyseal fractures of the tibia from a prospective comparison study. They prefer the intramedullary nailing, because of shorter operating and radiation time, and easy removal of the implant.
If surgeon makes incision at fracture site for accurate bone alignment and rigid fixation, this incision may be cause of a soft tissue injury, a failure or delay of a bone healing, and an infection. Over the past decades surgeons have tried to fix bone fragments by inserting implant away from the fracture site through minimally invasive incision. This surgical technique, called minimally invasive fracture surgery, can keep blood flow to the injured tissues and reduce the risk of infection. Consequentially, it shows good surgical results and early recovery than the conventional surgical methods.
In early state of MIPO (minimally invasive plate osteosynthesis), David et al. were confident that the MIPO technique for the treatment of distal tibial fractures would be a feasible and worthwhile while avoiding the severe complications [14].
Mahmood et al. [29] reports that minimally invasive technique shows less blood loss, minimal soft tissue destruction, shorter hospital stay, and early mobilization comparing to conventional methods in dynamic hip screws for fixation of intertrochanteric fractures of femur. Wong et al. [46] concluded that MIDHS (Minimally invasive Dynamic Hip Screw) fixation for intertrochanteric femoral fractures is superior to the conventional technique from their double-blind, prospective, randomized, and controlled clinical trial. The MIDHS produces less blood loss, less pain and a shorter rehabilitation period, while still achieving good radiological outcome.
MIPO for mid-distal humeral shaft fractures could effectively treat with advantages of shorter fracture union time and lower incidence of iatrogenic radial nerve palsies but with similar functional outcomes to the conventional open plating technique [1].
2.1.1 Related Issues
Though the minimally invasive fracture surgery has many advantages as describe above, it has also disadvantages, such as technical demanding, malreduction, malalignment, and radiation exposure.
Malreduciton
Krettek et al. [23] discussed that MIPPO (Minimally Invasive Percutaneous Plate Osteosynthesis) technique is technically demanding and the intraoperative determination of limb alignment must be improved, though the technique yields clinical results comparable to those achieved with the traditional plating techniques. Khoury et al. [19] pointed out that for the MIPO technique, reduction should be performed cautiously due to the tendency of sagittal plane malreduction. Apivatthakakul et al. [2] also agreed that major complications of MIPO were malalignment and screw breakage. Buckley et al. [6] reported that the incidence of malrotation was 38.5 and 50 % respectively, following fixation of distal femoral and proximal tibial fractures with the minimally invasive percutaneous osteosynthesis technique.
Radiation Exposure
Muller et al. reported that the recommended dose limit of 500 mSv to the dominant hand of the primary surgeon and first assistant would be exceeded if more than 407 intramedullary nailing procedures. But the radiation dose to thyroid by wearing lead protection were very lower compare to 300 mSv per year. Despite the relatively low dosage, they recommend to reduce the radiation dose to a minimum, considering a risk of incidental radiation damage [32].
Madan and Blakeway [26] showed that it was within acceptable limits that the overall radiation to patient’s gonads and surgeon’s hands in intramedullary nailing of the lower limb. On the other hand, they warned a surgeon to avoid live fluoroscopy, because they do not yet know the long-term effects of radiation.
Thomas et al. found that the hands are at higher risk and additional hazard is created for the less experienced surgeons [4]. Michael et al. concluded that the emission of radiation depends on the fracture type and the experience; higher emission rates are surveyed at the type C fractures and inexperienced team members [22].
Kim and Kim [21] reported that surgery must be more cautious about radiation exposure during fracture management from their experimental result, which the estimated annual equivalent dose outside the lead apron was close to or higher than the maximum limit of radiation exposure. Particularly, radiation exposure times of the minimally invasive intramedullary nailing and MIPO were higher than other orthopaedic surgeries.
Physical Burden
Weight of limbs and stiffening of muscles are other problems. Medical team endures to pull out a lower limb for fracture reduction. The weight itself makes difficult for traction of limbs, and the stiffening makes it more difficult.
Maeda et al. measured forces and torques that were applied to the lower limbs of 62 healthy and young volunteers with the robotic system, the end effector of which connected to the patient’s lower limbs using a boot. The average of maximum traction force was 232.9 N, range from 114.0 to 311.0 N, and the maximum torque was 6.31 Nm in external rotation and 7.69 Nm in internal rotation [28]. Maximum traction forces were 267.7 N in male group and 201.6 N in female group. Their following works, including subjects of seven female patients with intertrochanteric fractures, reported that the average traction force and rotation torque needed for reduction were 215.9 N, ranged from 146.3 to 294.9 N, and 3.2 Nm, respectively [27].
On the other hand, Gosling et al. measured forces and torque during fracture reduction in seven patients with eight fractures of the femoral shaft using a load cell that is connected to distal bone fragment with two Schanz screws. Results showed that the maximum resulting force was 411 N and the maximum resulting torque 74 Nm using the load cell [10].
In short, the minimally invasive fracture surgeries have shown similar outcomes to the conventional surgical techniques at many kinds of fracture surgeries. And it has many advantages such as less blood loss, minimal soft tissue damage, shorter rehabilitation, shorter hospital stay, and lower incidence of complication. On the other hand, the surgeons should be experienced in an accurate bone alignment and screw insertion. They give attention to malalignment and radiation exposure. And they must also put up with the large force required to pull out the lower limbs during fracture reduction.
2.1.2 Suggestions
Advantages and disadvantages of the minimally invasive fracture surgery are listed up in Table 1. It would be a good suggestion to use a robotic system which may utilize its advantage while overcome its disadvantages in the minimally invasive fracture surgery. As mentioned before, the robot system can provide the precise motions for the accurate bone positioning and the exact determination of insertion point of fixation screws. The robot has sufficient power to pull out a lower limb and to endure its position during a fixation procedure of bone fragments. Of course the robot can work in hazardous condition like high radiation exposure area. In this case, the robot can be controlled in remote, or it will automatically work. With these points, several research groups have developed the robotic system for fracture surgery.
Table 1
Minimally invasive fracture surgery and benefits of robotic system
Minimally invasive fracture surgery | Benefits of robot | |
---|---|---|
Advantage | Disadvantage | |
Good or similar outcome to the conventional technique Avoiding severe complication Less blood loss Minimal soft tissue destruction Early mobilization Shorter rehabilitation Shorter fracture union time Lower revision incidence | Technically demanding Malalignment/Malrotation Difficult Screw insertion Additional radiation exposure | Standardization of surgeon’s skill Precise motion Work at hazardous condition Heavy load |
2.2 Previous Studies for Robot Assisted Fracture Srugery
The robot system has two main roles in fracture surgery; one is a guidance or insertion of a needle or a screw, and the other is a positioning the bone fragment for a fracture reduction.
Though there are already some commercialized surgical navigations for determining the insertion positon, only a few laboratory-level’s robotized systems are reported. K. Bouazza-Marouf et al. first introduced robot assisted orthopedic surgery. Their robot allowed a drill-bit guide to be automatically aligned with an intra-operatively planed drilling trajectory [5]. Shoham et al. [41] have developed a bone-mounted miniature robot to precisely position and orient a drill or a needle for the same use.
The robotic system for assisting the fracture reduction is also not commercially available until now. A reduction robot system called “RepoRobo” was firstly introduced by Fuchtmeier et al. [9]. They converted a commercial industrial robot for medical use by appropriate modification. The requirements for using the industrial robot for the reduction of femoral shaft fractures are well described.
Westphal et al. also tried to use an industrial robot for medical use. They showed that robot assisted fracture reduction for the femur provides a high precision in alignment, while reducing the amount of intra-operative imaging from the statistical analysis [11]. Then, they have developed a surgical telemanipulator system to support long bone fracture reduction procedures [44, 45]. A joystick with force feedback was installed in their system as the control device for the telemanipulator, and the interaction for 3D fraction reduction with a 2D joystick input device was described. They showed the very accurate reduction results in their experiments with the telemanipulator.
Gramham et al. introduced a parallel robot for long bone fracture reduction [12, 31]. They used a foot holster to fix the leg to the parallel type robot. The geometric model of the bone fragments were visualized in a monitor. They also introduced force modeling during fracture reduction to determine the requirements for a robotic device.
Ye et al. [47] suggested a hybrid robotic system to balance the accuracy, payload and workspace. And Tang et al. [42] showed a hexapod computer-assisted fracture reduction system could reduce long-bone diaphyseal fractures effectively.
Mitsuish et al. had developed a fracture reduction assisting robotic system, which consists of a newly designed robot for only medical user, and a reduction path navigation system based on 3D CT imaging [30, 43]. The foot is fixed using a boot like the conventional fracture table. Clinical data of reduction forces/torques are reported with this robotic system by Maeda et al. [28] as mentioned before. An automatic reduction for fracture surgery was also developed by modifying this system [16]. The robot was fixed to a distal bone fragment through two fixation pins and the goal position and the reduction path were generated from a navigation system based on surgeon’s opinion. The phantom experiment results were very promising.
3 Safety Issues of Robot Assisted Fracture Reduction
In case of an industrial robot, the major strategy for ensuring human safety is to physically separate the robot from vulnerable human by creating a safety zone from robot’s workspace. However, this strategy is obviously inappropriate to surgical robots which interact with surgeons. Moreover, the surgical robots need to touch an affected part or to be coupled to patients for the treatment.
3.1 Related Studies
Fuchtmeier et al. [9] suggested some safety features of “Reporobo”. First, the robotic system has the velocity limits using the large reduction gear ratios, though the general operating speed of the robot may be controlled via software. At the same time, “back-driven” should be possible so that the surgeon can physically move the robot arm away from the patient in an emergency. “Harmonic drives” were applied to their system in this end. Secondly, they used a robotic gripper with integrated compliance to prevent the robot from pulling the fixator out. Lastly, they designed the control software for the force sensor to enable a free definition of the load limits to avoid over-correction and over-extension of the extremity.
Westpal et al. [44] took safety precautions by dividing the project into two layers, a software layer and a hardware layer, while developing the surgical telemanipulator. The software layer checks all the variable sensor information against predefined thresholds. If one safety threshold is exceeded, a stop command is sent to the robot control unit as soon as possible. For instance, if forces/torques exceeds a defined threshold, the motion of the robot is immediately stopped, and subsequently only those motions that lead to a reduction of the applied forces are allowed. Position information is used to limit the translational or rotational speeds. The hardware layer also limits the forces or torques with a load limiter (ULS 100, IPR-Intelligente Peripherie fur Roboter GmBh, Schwaiger, Germany). As soon as the forces or torques applied to this device the thresholds, the robot controller performs an emergency stop.
Gramham et al. [12] explained that parallel robots have inherent safety such as an increased stiffness, a high gear ratio, and high accuracy. Thus, they used the parallel robot mechanism for fracture reduction. And it was said that they plan to incorporate safety features such as a watchdog timer, a dead-man switch, force monitoring, encoder redundancy, and software motion limits.
3.2 Hazard Analysis
Here, safety and system design methods will be described based on developing experience at the University of Tokyo [17]. First of all, inherent hazards of the surgery and the robot-induced hazard should be listed up. Figure 2 shows the hazards related to a robot-assisted fracture reduction. An indirect reduction means that connection part does not directly connect to the bone fragment. In this way, limbs are just grabbed by hands or seized with a connection part like a boot. The conventional surgical technique belongs to the indirect reduction. A direct reduction method was suggested to improve reduction accuracy by fixing an end effector to a bone fragment using fixation screws. And a robotic system was introduced for more accurate and safe fracture reduction. Though the accuracy of the reduction can be increased by applying the new technique to the conventional methods, the extended system also results in an increase of number of hazards. However, if the robotic system is able to control the hazards satisfactorily, the application of this system could increase reduction accuracy with lower risk. Some hazards that must be controlled and its preventive measures will be suggested.
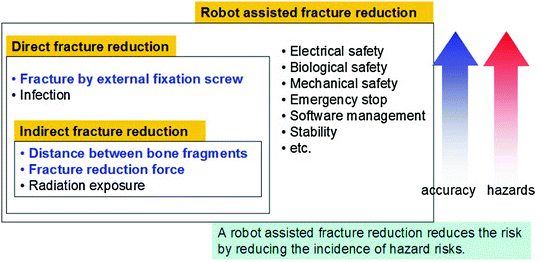
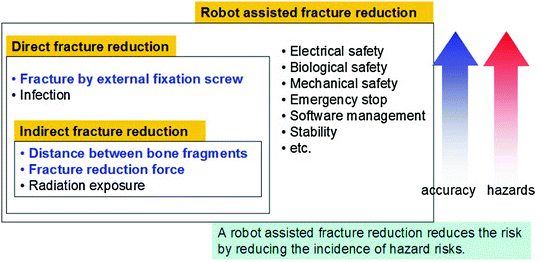
Fig. 2
Hazards related to robot assisted fracture reduction
3.2.1 Distance Between the Bone Fragments
Excessive traction may injure the sciatic nerve, which starts in the lower back and runs through the buttock and down the lower limb and serves nearly the whole of the skin of the leg, the muscles of the back of the thigh, and those of the leg and foot. Though traction of the limbs is required, it must be a safe range. Surgeons recommend restricting the traction distance between a distal and a proximal bone within 10 mm. A navigation system can measure the traction distant. The navigation system sounds an alarm for over-traction and sends a stop signal the robot for stopping. The motions of the robot are spatially constrained to minimize a reduction motion.
3.2.2 Fracture Reduction Force
Excessive reduction force causes the injury of the soft tissues. The robot needs to have functions that can limit the reduction force to a safe range. The safe range can be controlled in reasonable limit because it is not easy to define the safe range using the previously reported data. In literature, traction force and torque is approximately 300 N and 5 Nm. Mechanical failsafe units and a software force limiter is designed for this end.
3.2.3 Fracture Caused by an External Fixation Screw
If the fixation pins were used to connect the end effector of a robot with a bone fragment, these pins have possibility to crack around its insertion point. A finite element analysis was used to estimate this problem.
Finite analysis models of two bones were prepared based on the CT-data of a healthy bone and a fracture bone of a patient. The fixation pin model was generated from the CAD model and its material was set as titanium, which is generally used in clinical application. The pins were inserted into five sites into the healthy bone and into seven sites on the fracture bone. Bending load and pull-out force were applied to the pins as shown in Fig. 3.
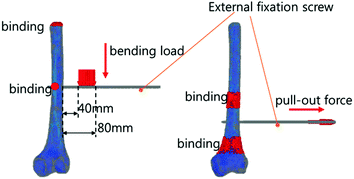
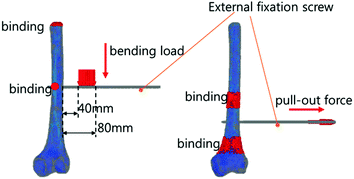
Fig. 3
Bending load and pull-out force in condition of finite element analysis
While the bending load and pull-out force was varied, the number of destroyed elements was estimated. The bending load was varied from 5 to 40 kg, and the pull-out force was varied from 20 to 200 kg.
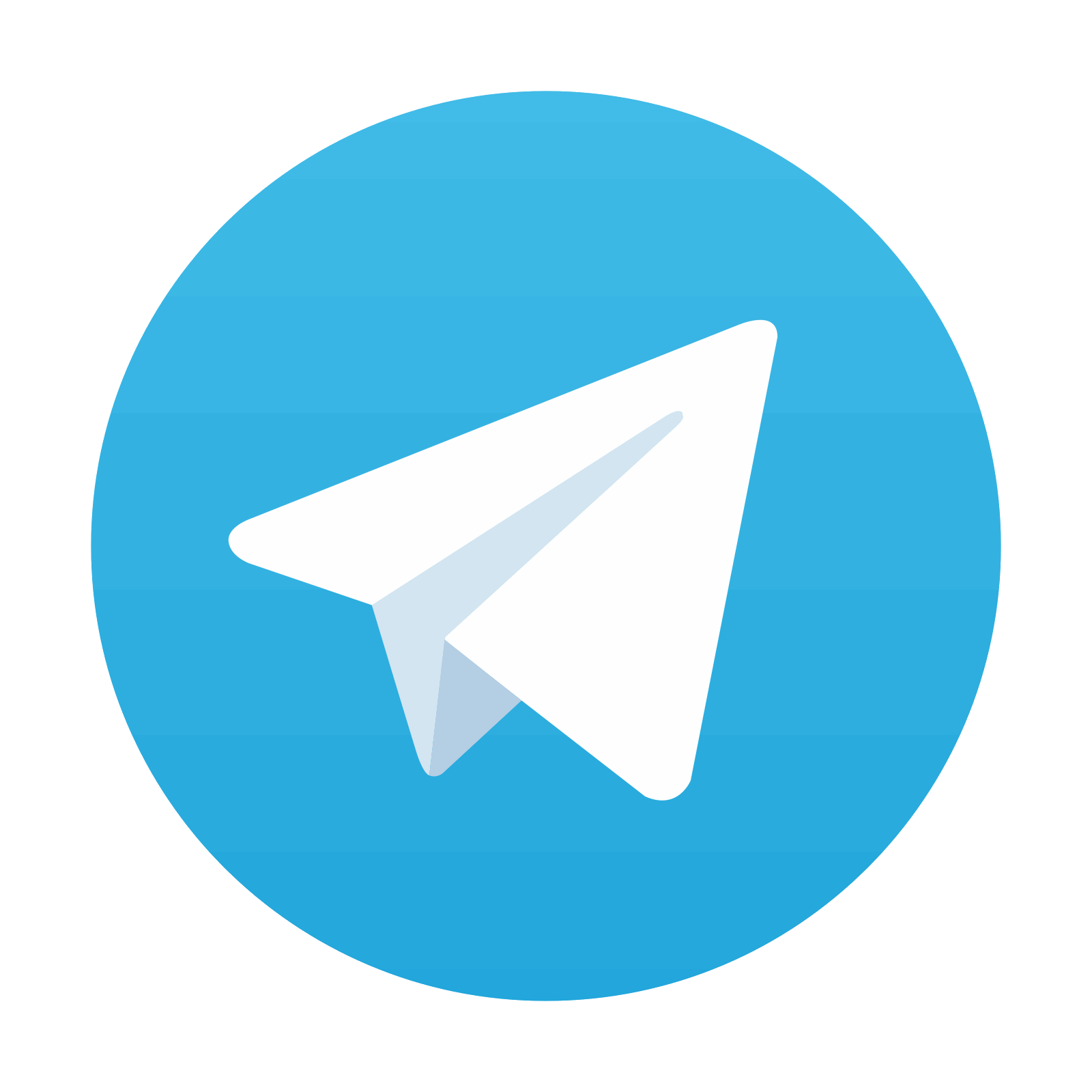
Stay updated, free articles. Join our Telegram channel

Full access? Get Clinical Tree
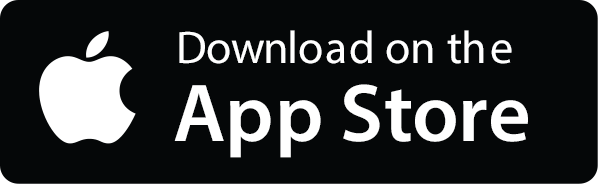
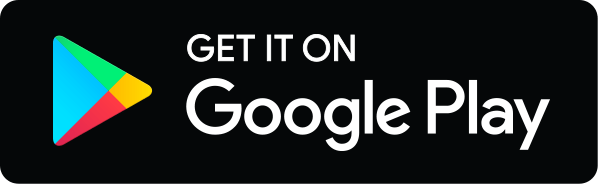