12 Eosinophils
Eosinophil Development and Morphology
Similar to neutrophils, eosinophils follow a classic pattern of granulocyte differentiation, passing through blast, promyelocyte, myelocyte, metamyelocyte, and band stages before reaching maturity. Along the way, eosinophils successively acquire morphologically distinct classes of granules. Factors required for eosinophil differentiation include granulocyte-macrophage colony-stimulating factor (GM-CSF) and interleukin (IL)-3, which also are required for neutrophil differentiation and cannot account for eosinophil commitment. An essential role for IL-5 in eosinophil development has been described, supported by the observation that intravenous administration of IL-5 rapidly results in peripheral eosinophilia. IL-5 may not be completely eosinophil specific, however, because studies in animal models suggest that it is also trophic for B cells. Similar to IL-5, IL-2 can stimulate eosinophilia. The IL-2 effect seems to be mediated through production of IL-5, however. CCL11 (eotaxin-1) also may cause bone marrow release of mature eosinophils and eosinophil precursors via engagement of CCR3 receptors, which are expressed mainly on eosinophils.1 Cooperation between IL-5 and eotaxins, in particular eotaxin-1, seems to be needed to induce tissue eosinophilia. Knockout mice with targeted deletion of CCR3 show deficiency in gastrointestinal eosinophils. Several transcription factors are involved in the eosinophilic lineage commitment, including the CCAAT/enhancer binding protein family (C/EFB) members, the interferon consensus sequence binding protein (ICSBP), and GATA-1.
Eosinophils contain at least three distinguishable classes of granules (Table 12-1). Primary granules form first and are analogous to the primary (azurophilic) granules of neutrophils. In contrast to neutrophils, eosinophil primary granules lack myeloperoxidase. Eosinophil primary granules are most numerous in eosinophilic promyelocytes and persist in smaller numbers in mature forms. In mature eosinophils, a lysophospholipase that is present in large quantities (7% to 10% of total eosinophil protein) has been tentatively localized to primary granules, which when released extracellularly precipitates into bipyramidal structures known as Charcot-Leyden crystals. Deposition of these crystals in tissues is taken as evidence of present or past eosinophilia.
Eosinophil-derived neurotoxin (EDN) (18 kD molecular weight), the third of the basic granular proteins, is slightly less basic (isoelectric point 8.9) than the aforementioned proteins and is present in smaller quantities. In contrast to MBP and ECP, which have likely roles in host defense, EDN is mainly recognized for its function as a neurotoxin for myelinated neurons, the evolutionary advantage of which is unclear. The Gordon phenomenon, in which injection of eosinophil-laden tissue into an animal produces profound neurologic deficits, is likely due to eosinophil-derived neurotoxin. It has been shown that EDN serves as an endogenous ligand of TLR2, can activate Myd88 in dendritic cells, and shifts adaptive immunity toward a T helper (Th)-2 response, suggesting a pivotal role for esoinophils in the innate-adaptive immune response.2 Finally, eosinophil specific granules contain large quantities of eosinophil peroxidase, an enzyme distinctly different from neutrophil myeloperoxidase, but probably subsuming the same function of generating hypohalides for cell killing and activation of latent proteinases. ECP, EDN, and eosinophil peroxidase are localized within the primary granule matrix region. A third population of smaller eosinophilic granules has been identified by virtue of its acid phosphatase and arylsulfatase B content and is present mainly in tissue eosinophils.
Eosinophil Activation and Distribution
Similar to neutrophils, eosinophils undergo activation in response to stimuli and are capable of adhesion, chemotaxis, phagocytosis, degranulation, and O2− generation. Eosinophils respond to many of the same chemoattractant stimuli as neutrophils, although with different sensitivities. In addition, eosinophils respond to stimuli that do not affect neutrophils, including IL-3, IL-5, regulation upon activation normal T cell expressed and presumably excreted (RANTES), and macrophage inflammatory protein (MIP)-1α. Whether the distribution of these factors is sufficient to explain the tissue distribution of eosinophils relative to other granulocytes is uncertain; however, eosinophils and mast cells secrete IL-3 and IL-5, suggesting their capacity to attract additional eosinophils to sites of atopy. Eosinophils express not only adhesion molecules identified on neutrophils—CD11a/CD18, CD11b/CD18, and L-selectin—but also others, such as the α4β1 integrin VLA-4. IL-4 and IL-13 induce expression of the VLA-4 counterligand, vascular cell adhesion molecule (VCAM)-1, via an eotaxin-1, STAT6-dependent pathway. Oncostatin-M, an IL-6/gp130 family member, also seems to upregulate VCAM-1 in an eotaxin-1, STAT6-dependent manner, and to play a role in eosinophil accumulation in a mouse model.3
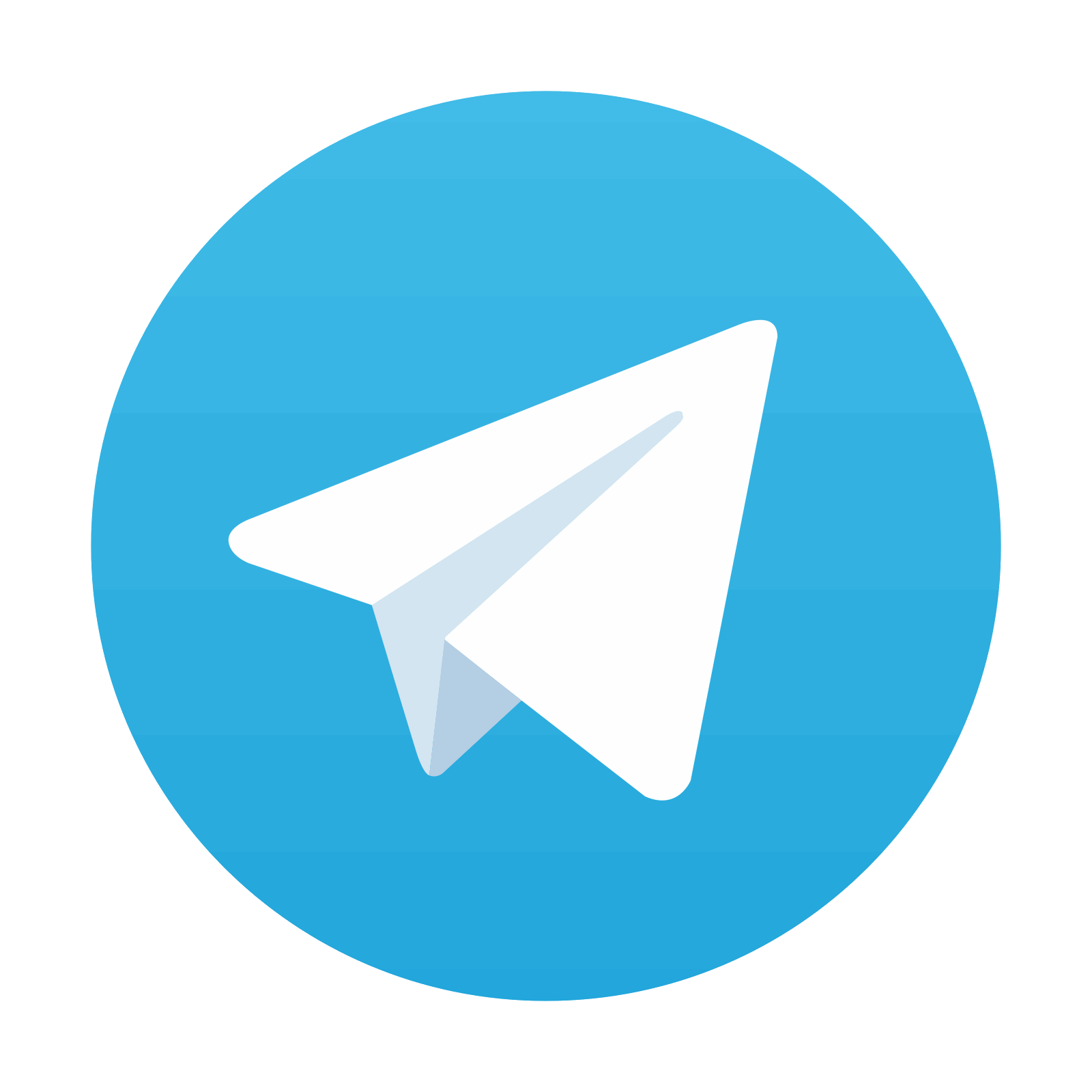