4.1 Diseases of the nervous system
Chapter 4.1a The physiology of the nervous system
At the end of this chapter you will:
Estimated time for chapter: Part 1, 120 minutes; Part 2, 100 minutes.
Introduction to the nervous system
This chapter focuses on the physiology of the nervous system. Together with the endocrine system, the nervous system is one of the topics explored in this text which arguably has the most relevance to the understanding of the physical basis of holistic approaches to healing. This is because, together with the endocrine system, the nervous system is involved in the process of communication of one body part with another. Together, these systems form, from a medical perspective, part of the physical foundation of what makes the body an integrated whole. It is important to point out that some holistic practitioners, (exemplified by Myers (2001) who writes about muscle trains), also propose that the myofascial system (comprising the muscles and the body-wide interconnected web of connective tissue that links them with the periosteum of the bones) is another integrated body system that is a medium for the communication of ‘energy’ between disparate body parts.
As there is a great deal of material to study, the chapter is divided into two parts. If you are following this text as part of a study course, you are recommended to work through this chapter in two separate study sessions. Part I describes the nervous tissue, which is the basic building material for the nervous system. The three parts of the nervous system, the central nervous system, the peripheral nervous system and the autonomic nervous system, are then introduced. Finally, the central nervous system is explored in more detail. In Part II the peripheral nervous system and the autonomic nervous system are described in more detail.
Part I The physiology of the nervous system
The nerve cells (neurons)
The physiology of the nerve cell
Figure 4.1a-I illustrates a generalised nerve cell, and shows the long extension of the axon and the smaller tree-like extensions called dendrites. The branching dendrite-like structures at the bottom of the axons have endings called ‘boutons’, which are adapted to release tiny quantities of neurotransmitter chemicals from secretory vesicles inside the cell. The axon is an extension that can be very long, and for this reason it can also be termed a ‘nerve fibre’. For example, the nerve cells that relay information from the feet have axons or nerve fibres that run from the spinal cord right down to the toes.
When a nerve cell receives a stimulus, the electrical impulse is conducted to the tips of all the dendrites and also down the length of the axon. Some axons are coated in a white substance called ‘myelin’. This myelin sheath is simply represented in Figure 4.1a-I, but the structure of myelin is complex as it is actually a fine layer of fatty substance secreted between layers of a glial cell membrane wrapped many times around the nerve fibre. The glial cell that forms myelin in this way is called the ‘Schwann cell’. Myelin enables these cells to transmit an impulse more quickly than those cells that do not have this coating.
The tips of the dendrites make contact with other nerve cells. In the case of motor nerves, the tips of some of the axons make contact with muscles. The way in which dendrites or axons make contact with other nerve cells is shown in Figure 4.1a-II. In can be seen from the figures that the branching ends of the axons come very close to the dendrites of another nerve cell. However, they do not actually touch, but leave a tiny gap called a ‘synapse’ or “synaptic cleft”.
Nerve cells are highly active because they constantly manufacture neurotransmitter chemicals. The manufacture of substances within the cell requires basic nutrients and energy in the form of energy-charged adenosine triphosphate (ATP), and takes place within the endoplasmic reticulum and the Golgi body (see Chapter 1.1b). The neurotransmitters are exported to the outside environment by the process of exocytosis of vesicles, which is one of the methods of active transport described in Chapter 1.1b. This process also requires energy in the form of ATP.
Glial cells (neuroglia)
The last type of glial cell, called the ‘microglia’, is derived from the leukocyte. It is similar to the macrophage in ordinary connective tissue, and can phagocytose (see Chapter 1.1c) unwanted cellular material.
The composition of nervous tissue
In the spinal cord, the tracts of nerve fibres are very prominent. Thousands of nerve fibres run up and down the length of the spinal cord, allowing communication between different parts of the body and the brain. These also appear as white matter when the spinal cord is dissected. However, in the core of the spinal cord are dense clusters of the nerve cell bodies of connector and motor neurons. On dissection these are seen as a central H-shaped area of grey matter. Figure 4.1a-III shows a diagram of a cross-section of the spinal cord, illustrating the appearance of the white and grey matter. Like the brain, the spinal cord is of a very soft consistency and is also vulnerable to injury.
Figure 4.1a-III also shows the two ‘roots’ of the nerve fibres that leave this part of the spinal cord. These meet to leave the vertebral column as a single spinal nerve containing thousands of nerve fibres. With the exception of the nerves that supply the head and the neck, all the bodily nerves originate from spinal nerves. In this case, the word ‘nerve’ means thousands of nerve fibres bundled together, rather than a single cell (correctly called ‘nerve cell’, “nerve fibre” or ‘neuron’).
All the nerves that run outside the spinal cord consist entirely of sensory and motor nerve fibres, and have originated from the anterior (containing motor fibres) and posterior (containing sensory fibres) spinal nerve roots (see Figure 4.1a-III). The cell bodies of the motor nerve fibres sit within the grey matter of the spinal cord. However, the cell bodies of the sensory nerve sit outside the spinal cord, mostly within the spinal root ganglion on the sensory nerve root. This is seen as a swelling in Figure 4.1a-III. The spinal root ganglion is, therefore, a little mass of grey matter. (NB: The sensory nerves of the autonomic nervous system have their cell bodies in ganglia situated further away from the spinal cord, as described later in this chapter.)
The central nervous system
The CNS consists of the brain and the spinal cord, and sits protected within spaces formed within the bony architecture of the brain and the vertebral column. Figure 4.1a-IV illustrates the structure of the main parts of the CNS. Note that this diagram is not drawn to scale; the spinal cord is, in reality, much longer than indicated in this drawing.
The meninges
The meninges (Figure 4.1a-V) consist largely of fibrous tissue. The outermost layer, known as the ‘dura mater’ (dura) is composed of dense white fibrous tissue, the outer layer of which is adherent to the skull, where it forms the periosteum (see Chapter 4.2a) and the bony margins of the vertebral canal (central canal) right down to the coccyx. In certain regions in the skull the dura splits into two layers, and venous blood courses in the spaces between them in canals called ‘sinuses’. An epidural anaesthetic is one that is injected carefully in the lumbar region of the spinal cord so that the anaesthetic drug collects outside the dura and so acts to numb the lower thoracic and lumbar spinal nerve roots as they course down to supply the abdomen and legs.
Beneath the layers of the dura is the subdural space. This contains fluid which separates the dura from the next layer, the arachnoid mater, which is also composed of fibrous tissue. The arachnoid mater loosely follows the internal contours of the dura mater. Veins also pass through the subdural space. These can become vulnerable to damage in old age as the substance of the brain shrinks, and there is more possibility of traction on these vessels if the skull is suddenly moved as can occur in a fall. This is the cause of subdural haemorrhage (see Chapter 4.1d).
Beneath the arachnoid mater is the subarachnoid space, which separates the arachnoid mater from the deepest meningeal layer, the pia mater. The delicate pia courses along the surface of the brain and the spinal cord, and in some areas there is quite a pronounced space between it and the arachnoid mater. This space is filled with a fluid known as ‘cerebrospinal fluid’ (CSF). This space is in connection with the CSF, which bathes internal portions of the brain within the chambers known as ‘ventricles’. It is in the subarachnoid space that the major arteries which course along the surface of the brain are located, and it is into this space that blood can leak should one of these arteries become damaged. This is the situation of subarachnoid haemorrhage (see Chapter 4.1d). A lumbar puncture is an investigation that involves inserting a needle into the region of the subarachnoid space as it widens out in the lower lumbar area below the termination of the spinal cord (see Figure 4.1-V) so that a sample of CSF can be obtained (see Q4.1a-3-Q4.1a-6).
The cerebrum
The cerebrum is the upper part of the brain (see Figure 4.1-IV), and is divided into two cerebral hemispheres. These have a convoluted surface, giving this part of the brain a cauliflower-like appearance. Despite this visual comparison with a lowly vegetable, the cerebrum is the most complex part of the brain, and is the part that is most enlarged in human beings compared to other animals.
The cerebrum deals with the most complicated aspects of nervous activity. To use once again the example of the pin prick, although the reflex withdrawal from the pain need not involve the brain at all, the emotional experience of pain, the cry of indignation and the laying down of memory about the event all involve the nerve cells in the cerebral hemispheres. In keeping with this complexity, the cerebrum contains a lot of grey matter (nerve cell bodies) concentrated on its convoluted surface. This part of the cerebrum is called the ‘cerebral cortex’. The functions of particular parts of the cerebral cortex have now been extensively mapped. For instance, the voluntary control of movement of all the body parts can be located onto precise segments of a single ridge of cortex, called the ‘motor area’, situated on the side of each cerebral hemisphere. The complexity of such representation is illustrated in Figure 4.1a-VI.
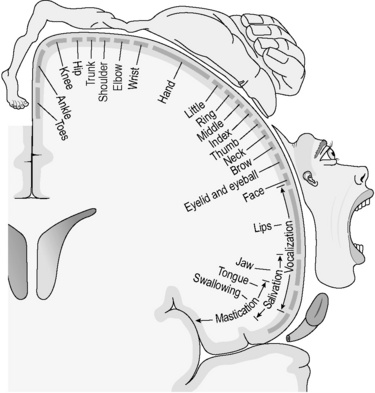
Figure 4.1a-VI • The mapping of the motor cortex to bodily function
• This diagram illustrates how different strips of the motor cortex have been mapped to muscle function in the body. The distorted humanoid figure is called a ‘homunculus’ and is drawn to illustrate that some parts of the body (the hand, face and tongue) have disproportionate representation on the motor cortex, in keeping with the complexity of their muscle action.
In order to comprehend the complicated effects of cerebral diseases it helps to understand that, if parts of the cerebrum are lost through injury or disease, the precise function of those parts is also lost. For example, a person who has a small stroke (see Chapter 4.1d) that damages a part of the motor area of the cortex only will suffer an inability to move the corresponding part of their body. In this situation, other functions such as sensation and speech will be unaffected. In contrast, a person who has a stroke that affects the visual cortex only will suffer from visual problems, while movement will be unimpaired.
The main areas and functions of the cerebral cortex are illustrated in Figure 4.1a-VII. The cortices are each anatomically divided into four lobes named after the skull bone that overlies them. Functions can therefore be associated anatomically with lobes; for instance, vision is a function of the occipital lobe. The areas associated with speech production (frontal lobe) and processing of language (temporal lobe) are concentrated on the so-called ‘dominant hemisphere’ only, which is usually on the left-hand side in right-handed people and the right-hand side in left-handed people. In contrast, the areas responsible for spatial awareness (parietal lobe) tend to be more developed on the non-dominant side of the cerebrum (see Q4.1a-7 and Q4.1a-8).
The brainstem
The brainstem consists of the midbrain, the pons variolii (the pons) and the medulla oblongata (the medulla) (see Figure 4.1a-IV). These three sections of the brain contain large tracts of white matter which carry nerve fibres between the cerebrum and the spinal cord.
The spinal cord
The spinal cord is the long extension of nervous tissue that leaves the brain at the base of the brainstem. It projects through the large hole at the base of the skull called the ‘foramen magnum’, and extends down as far as the first or second lumbar vertebrae. The spinal cord is protected as it runs in the vertebral canal (also known as ‘spinal canal’), formed by the ring-shaped vertebrae that sit one on top of the other. The solid vertebral bodies lie in front of the spinal cord, and the spinous and transverse processes project out to the back and the side of the vertebral rings that embrace the cord along it length. A section of the thoracic spinal cord is illustrated in Figure 4.1a-VIII.
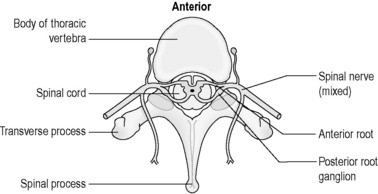
Figure 4.1a-VIII • Horizontal section through the vertebral column and spinal cord at the level of the thorax.
As shown in Figure 4.1a-IX, the 31 pairs of spinal nerves leave the cord at regular intervals. These spinal nerves project out of the meningeal membranes, which protect the cord and leave the vertebral column through spaces between the vertebrae. Figure 4.1a-X illustrates the base of the spinal cord as it looks on a vertical section of the vertebral column. This diagram shows how the cord only goes down as far as the first lumbar vertebra. The labels T1, T7, T12, L1, S1 and S3 point to the solid bodies of three thoracic vertebrae, one lumbar vertebra and two of the sacral vertebrae which are fused to form the sacrum bone. This diagram also shows the spinous processes projecting back from the vertebral canal. These processes are linked by a tough fibrous ligament, not shown in this diagram, which runs from the base of the skull to the sacrum. It is this ligament that is penetrated when a lumbar puncture is performed.
Figure 4.1a-IX shows the paired spinal nerves. In this diagram the nerves are cut off just at the point where they exit the vertebral canal between the vertebrae. This shows how the lower thoracic, lumbar and sacral nerves descend by increasing lengths through the vertebral canal before they actually leave the space between the vertebrae.
The spinal cord consists of tracts of white matter containing vertically ascending and descending nerve fibres, and a core of grey matter containing cell bodies. This core is approximately H-shaped when seen in horizontal section (see Figure 4.1a-VIII). Some of the nerve fibres in the white matter of the spinal cord travel to and from the brain, whereas others simply link different levels in the spinal cord.
The spinal cord receives sensory information from most parts of the body below the head and the neck through sensory nerve cells that enter the cord through the sensory root of the spinal nerves. The muscle action within the body is controlled by information carried by motor nerve cells that leave the spinal cord at the motor root of the spinal nerves. (see Figures 4.1a-III and 4.1a-VIII).
The protective reflex arc
The protective reflex of withdrawal from pain is one example of a movement that is controlled by the spinal cord alone. Once again the example of the response to a pin prick of the finger is a useful illustration. In this case, the pain of a pin prick leads to impulses in the sensory nerves that supply the finger. These travel up the arm to where the nerves enter the spinal cord at the thoracic level of T6/T7. Here the sensory nerve cells make connections with a number of motor nerve cells in the spinal cord by means of connector nerve cells. These motor nerve cells send impulses down to various muscles in the arm and shoulder to cause a withdrawal of the arm from the pain. In such a reflex, the brain is not involved. A diagrammatic representation of a simple reflex arc is given in Figure 4.1a-XI. In this case the diagram illustrates the “knee jerk” reflex which is an involuntary response to the stretch of the patellar tendon.
Part II The physiology of the nervous system
The peripheral nervous system
The spinal nerves
The structure of the spinal cord was described at the end of Part I of this chapter. The 31 pairs of spinal nerves, which leave the cord at regular intervals (see Figure 4.1a-IX), divide to form the largest part of the PNS.
The division of the spinal and cranial nerves
Each spinal and cranial nerve branches in a tree-like fashion to ‘supply’ a particular portion of the body. In the cervical, lumbar and sacral regions, the adjacent spinal nerves intermingle with each other close to the spinal cord before they separate out again into a number of nerve branches that then supply the tissues of the neck, arm, leg and genital areas. This intermingling forms a net-like web of nerves called a ‘plexus’. Figure 4.1a-XII illustrates the location of the major nerve plexuses.
Dermatomes
The parts of the body that are supplied by each spinal and cranial nerve are clearly understood. The areas of skin and tissue supplied by the sensory nerves of each spinal nerve have been mapped, and are much the same for each person. These areas are called ‘dermatomes’, and are illustrated in Figure 4.1a-XIII. Dermatomes represent those strips of skin and areas of the body that are supplied by the individual spinal and cranial nerves. Although the diagram suggests that there is a clear demarcation between dermatomes, in reality this is not the case, there being considerable overlap of the regions supplied by adjacent spinal nerves. Nevertheless, these diagrams can be very useful for health practitioners because they indicate which spinal nerve supplies the nerves to whatever body part is a source of symptoms or a focus for treatment. Some dermatomal diagrams also indicate which nerve branch (e.g. the radial nerve or the sciatic nerve) supplies a particular area. Figures 4.1a-XIV and 4.1a-XV show examples of such diagrams for the arm and the leg, respectively (see Q4.1a-11).
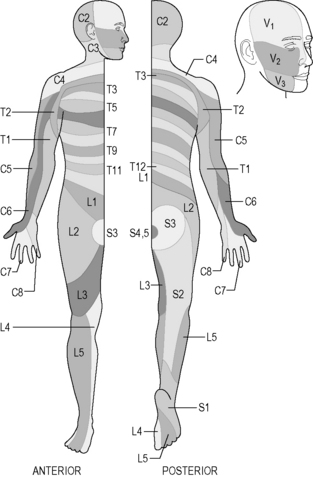
Figure 4.1a-XIII • Dermatomes of the spinal roots and the three divisions of the fifth (V) cranial nerve on the face (the trigeminal nerve).
The autonomic nervous system (ANS)
of the body is responsive to its precise requirements at any one time. For example, the ability of the cardiovascular system to modify the pulse rate and blood pressure according to the needs of the body is mediated by the ANS.
The sympathetic nervous system
The nerve cells of the SNS originate in the brainstem, and descend to connect with nerves in the spinal cord between T1 and L3. From these levels, fibres leave the spinal cord through the spinal nerves and intermingle with each other in a chain of nervous tissue that runs on either side of the spinal column at the back of the abdominal and thoracic cavities. There are a number of cell bodies, known as the ‘sympathetic ganglia’, within this chain of nervous tissue, and thus it can be compared to the grey matter of the brain and the spinal cord (the term ‘ganglion’, from the Greek meaning ‘lump’, is used in this case to refer to a cluster of nerve cell bodies). Figure 4.1a-XVI illustrates how nerve fibres leave the sympathetic ganglia to travel to all body parts, and indicates the wide range of tissues and organs that are influenced by the sympathetic nerves.
As mentioned above, the sympathetic part of the ANS is sometimes described as the part of the nervous system that prepares the body for fight or flight. It causes physical changes that allow the body to expend energy in a focused way, such as was described in the example of running for the bus. The various actions of the SNS are listed in Figure 4.1a-XVI, and it might become clear that all these changes, such as dilatation of the pupil, inhibition of mucus secretion, increased heart rate and reduced peristalsis, are appropriate ones for a body that is about to go into action.
Information Box 4.1a-II The sympathetic nervous system: comments from a Chinese medicine perspective
The parasympathetic nervous system
The parasympathetic nerves originate in the brainstem and the sacral part of the spinal cord. In particular, the vital centres in the brainstem, which deal with essential functions such as respiration and the heart rate, contain many parasympathetic nerve cells. These nerve cells have fibres that leave the cord either through the four pairs of mixed cranial nerves (the oculomotor, facial, glossopharyngeal and vagus nerves) or within the S2, S3 and S4 spinal nerves. The mixed cranial nerves have parasympathetic branches that extend to supply the deep organs and glands of the head, neck and abdomen down to the upper part of the large intestine. The parasympathetic fibres in the sacral spinal nerves supply the lower part of the large intestine, the bladder and the genital organs. Figure 4.1a-XVII illustrates the structure of the PSNS.
Figure 4.1a-XVII shows how the various organs and glands are supplied by nerves that originate from the brainstem and the sacral spinal cord. The parasympathetic nerves increase secretions from the eye, the mouth and the digestive tract. Although not indicated in this diagram, the production of mucus of the respiratory tract is also increased. These secretions are all protective and nourishing to the organs that produce them. The heart and respiratory rate slows with parasympathetic stimulation, and digestion, defecation and urination are also promoted.
