Chapter 6 Biomechanics of the spine
Physiologic loads
Mechanical loading of the spine is an important factor in the etiology of spinal disorders. It also affects the outcome of orthotic treatments for spinal disorders. Loads on the human spine are produced by (1) gravitational forces due to the mass of body segments, (2) external forces and moments induced by a physical activity, and (3) muscle tension. These loads are shared by the osseoligamentous tissues and muscles of the spine. Tensile forces in the paraspinal muscles, which exert a compressive load on the spine, balance the moments created by gravitational and external loads (Fig. 6-1). Because these muscles have a small moment arm from the spinal segment, they amplify the compressive load on the osseoligamentous spine.
Stability of the spinal column
Load-bearing ability of the osteoligamentous spine
In the absence of muscle forces, the osteoligamentous spine cannot support vertical compressive loads of in vivo magnitude. Experiments in which a vertical load was applied at the cephalic end of cervical, thoracolumbar, or lumbar spine specimens caused buckling of the spines at load levels well below those seen in vivo. The stability of the spine, characterized by a critical load (maximum load carrying capacity, or Euler buckling load of spinal column), was determined by these experiments. When the load exceeded the critical value, the spine, constrained to move only in the frontal plane in these experiments, became unstable and buckled. The cervical spine buckled at a vertical load of approximately 10 N, the thoracolumbar spine at 20 N, and the lumbar spine at 88 N, all well below the compressive loads expected in vivo during activities of daily living. When a compressive load is applied in a vertical direction to a multisegment spine specimen, segmental bending moments and shear forces are induced as a result of the inherent curvature of the spine. This load application causes large changes in the specimen’s posture at relatively small loads. Further loading can cause damage to the soft tissue or bony structures.
Stability of the spinal column under a follower load
It can be reasoned that coactivation of trunk muscles alters the direction of the internal compressive force vector such that its path follows the lordotic and kyphotic curves of the spine, passing through the instantaneous center of rotation of each segment (Fig. 6-2). This would minimize the segmental bending moments and shear forces induced by the compressive load, allowing the ligamentous spine to support loads that otherwise would cause buckling and providing a greater margin of safety against both instability and tissue injury. The load vector described is called a follower load.
Experiments on human cadaveric specimens of lumbar (L1–5), thoracolumbar (T2–sacrum), and cervical spines (C2–7) as well as mathematical models have demonstrated that (1) the ligamentous spine with multiple motion segments can withstand physiologic compressive loads without tissue injury or instability if the compressive load vector is applied along a follower load path approximating the curve of the ligamentous spine, (2) the ligamentous spine subjected to compressive preloads of in vivo magnitude along the follower load path permits physiologic mobility under flexion–extension moments, and (3) the follower preload simulates the resultant vector of muscles that allow the spine to support physiologic compressive loads. Intradiscal pressures in human cadaveric lumbar spines under a follower preload are comparable to those measured in vivo, and spinal stability is increased without compromising its mobility in flexion–extension and lateral bending. A superimposed follower preload renders more physiologic the in vitro loading of the ligamentous spine with pure moments.
Stability of the functional spinal unit
< div class='tao-gold-member'>
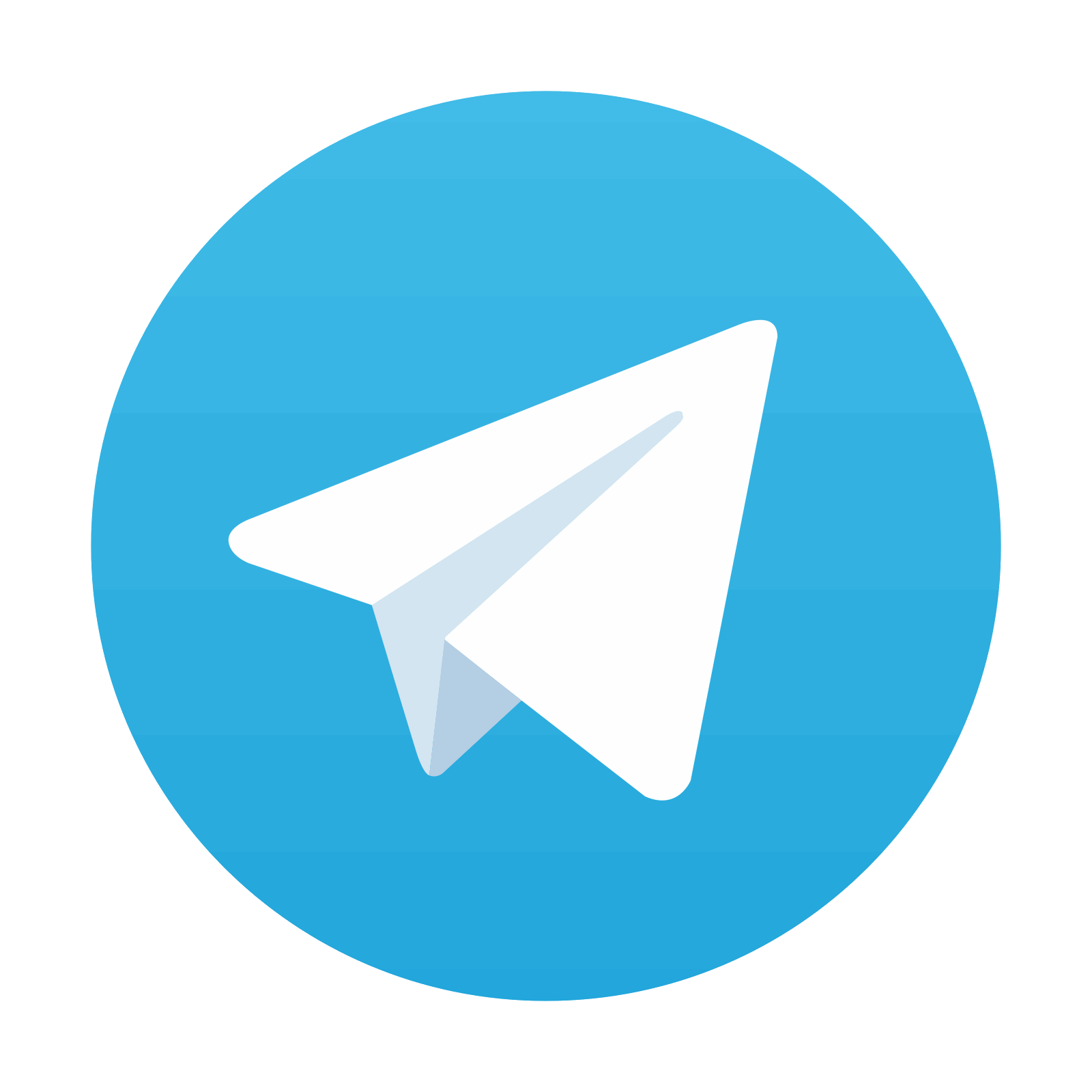
Stay updated, free articles. Join our Telegram channel

Full access? Get Clinical Tree
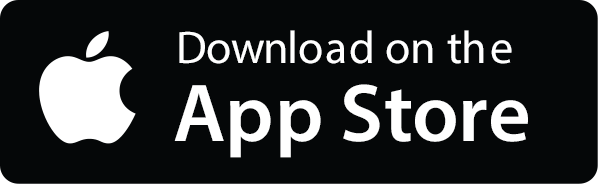
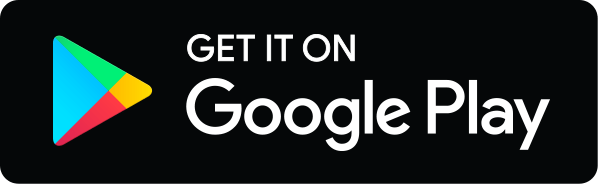