Results of studies attempting to investigate analytical epidemiology should be viewed with caution given the design limitations present in nearly all such studies. In most cases, these studies are able to link a risk factor with injury; but this in no way suggests that the risk factor causes the injury (Meeuwisse et al., 2007). For example, taller gymnasts have been reported as more at risk of injury. This does not suggest that a particular gymnast’s height caused an injury, but rather that height may be one of several contributing factors in the occurrence of an injury. It is possible that such factors as greater height, weight, and age tend to characterize older gymnasts with more years training and involvement in higher levels of training and competition.
Measurement of load
Given the high magnitude of impact load in landings, both acute and accumulative, coaches should monitor impact loads per training session, taking into consideration training quality and quantity such as the control of rotation and the height from which the landings are executed. For these we need practical methods for monitoring training loads. Impact load can be measured biomechanically by the use of instrumented equipment (e.g., beat board), instrumentation on the gymnast (accelerometers), or by landings on force plates. A variety of gymnastic-specific movements have been examined in terms of impact. Pressure distribution under the sole of the foot during leaps and landings, three-dimensional analysis of arm impact during gymnastic back handsprings in children, impacts during beam and floor training in preadolescent gymnasts via video, force platform, and accelerometer (Burt et al., 2007), and elbow joint forces and technique differences in the Tsukahara vault have all been reported. Video and force analysis of six young female gymnasts showed that reaction forces at the hand during back handsprings produced large compression forces (an average of 2.37 times BW) and sizable valgus moments at the elbow (an average of 0.03 times BW) (Koh et al., 1992). The combination of these forces may contribute to the occurrence of lateral compression injuries of the elbow joint such as osteochondritis dissecans of the capitellum. Correlations of measures of elbow angle and measures of reaction force showed that large elbow flexions may protect the elbow from large valgus moments.
Feedback systems via equipment instrumentation can help to monitor training loads, detect fatigue, and improve technique acquisition. However, monitoring training objectively in gymnastics, with the exception of qualitative video feedback, is not common. Some researchers have instrumented men’s gymnastics apparatus such as the rings and horizontal bar; however, application to training or how they might be adapted for use with women’s apparatus, remains unclear. A vault feedback system has been used to provide reliable approach velocity through to beat board contact, as well as preflight and table contact time during regular vault training of elite gymnasts. The advantage of this system was that it was designed to work as a feedback tool for coaches, with simple operation and result/data delivery via a hand-held personal digital assistant device. The system does not require a biomechanist to be present for its operation, or to analyze and interpret the data. This system has potential as an injury prevention tool by alerting a coach when the gymnast is becoming fatigued. For example, the table contact time during vaulting for female gymnasts is approximately 200 milliseconds. A 10% (20 milliseconds) increase in table contact time for an individual gymnast, depending on their task performance variability, indicates a performance decline and potential fatigue. Instrumentation of the vault table and beat board with pressure systems could potentially provide an estimate of force, or actual force could be provided via instrumentation with force plates. However, whilst instrumentation of vaulting apparatus is feasible, careful software development is needed to enable detailed information such as table contact forces to be readily and easily accessible to coaches during training.
Beatty et al. (2005) provided descriptive data on typical impact loads and decelerations during gymnastics training, however no insight was provided on how these measures could assist with injury prevention measures. Burt et al. (2007) used video analysis of training sessions along with accelerometer and force platform peak ground reaction force data to quantify the effects of participation level (international and national), apparatus (beam and floor), and training phase (precompetition and competition) on estimates of training load in gymnasts aged 7–13 years. Monitoring the quality of the training program by counting the number of rotations and heights from which landings are executed, or measuring decelerations during impacts with accelerometers, may be a better injury prevention tool than simple quantification of training hours.
Limb asymmetry, alignment, stiffness, and joint range motion affect load
The neuromuscular control of the center of mass trajectory to control the body’s momentum and angular rotation is specific to the landing task, whether landing from a height without any angular rotation, or from forward or backward somersaults (McNitt-Gray et al., 2001). The amount of knee flexion has an influence on the magnitude of the impact force. A less erect landing posture through increased trunk flexion (forward lean) can encourage more knee flexion and a reduction in the landing impact forces. Either large or restricted ranges of motion are known to produce overuse injuries to the hips (Caine & Lindner, 1985) and back (Kujala et al., 1997).
High-impact landings can result in undesirable movement variability that increases energy expenditure. In contrast, within- or between-joint coupling (coordination) variability may play a functional role in reducing injury through variable loading of the musculoskeletal features of the joint, providing the ability to adjust to changing environments. High coupling variability during the execution phase of a task could enable the gymnast to adjust for various intrinsic factors such as confidence and fatigue, and extrinsic factors such as competition arena temperature, which can influence their performance.
Attenuating more force on one leg amplifies the risk to gymnasts of sustaining an acute or a chronic injury, and is one of the most common means of injury in other sports (Kovacs et al., 1998). Functionally symmetrical drop landings have ranged from less than 10% difference between limbs to 73% asymmetry. The ground reaction forces of two-foot landings in gymnastics are significant during training (~5 BW) and competition (~11 BW), especially if the landing is uneven (~18 BW) or if there is unusual foot placement. Landing with two feet together after high airborne skills requires tremendous stabilization and eccentric strength to prevent the knee joints from collapsing due to the high external knee joint loads. An uneven landing or unusual foot placement can result in increased dynamic valgus knee moments and increases the load on the ACL several-fold. Ideally, two-foot (toe-heel) landings should be performed with even distribution of forces between the feet that are spaced roughly shoulder width apart, with actively controlled hip, knee, and ankle dorsiflexion with the knees over the toes.
Muscles serve as the primary active stabilizers during functional loading conditions, protecting against musculoskeletal injury. Active muscle stiffness contributes to musculoskeletal behavior and is essential for the maintenance of joint stability. Musculoskeletal stiffness regulates the storage and reuse of elastic energy and is defined as the collective ability of muscles, tendons, ligament, cartilage, and bone to resist deformation from an applied force. Lower limb joint stiffness (resistance to movement) is the relationship between the deformation of the leg and the force attenuated. While some lower limb stiffness may be necessary for performance, either too much or too little stiffness may lead to injury. Too much stiffness may lead to bony injuries and ankle pathology whereas too little stiffness may lead to soft tissue injuries and knee pathology. Stiffness is well suited to quantifying the behavior of musculoskeletal components (i.e., joints, ligaments, tendons, cartilages, muscles, and bones) because it describes the resistance of a body to applied forces.
Increased lower limb musculoskeletal stiffness, as measured during self-paced repeat jump and hop tasks (15 contacts of continuous bent-knee and continuous straight-leg hops with one foot placed on each of two portable force plates), was related to an increased incidence of overuse injury in 39 adolescent female athletes involved in high-impact sports, including 17 high-level athletes (Moresi et al., 2010). Screening for musculoskeletal stiffness may allow “at risk” athletes to be identified and potential athlete monitoring or intervention programs to be implemented.
Analysis of ankle stiffness measures of self-paced double-legged hopping in gymnasts has shown a potential safe zone for ankle plantarflexion stiffness. Bradshaw and Hume (2012) reported that two gymnasts from an original study by Bradshaw, who were still training 8 years later, showed increased ankle plantarflexion stiffness by 10.8 and 13.9 kN/m. Both gymnasts reported Sever’s disease (calcaneal apophysitis due to overuse and repetitive microtrauma of growth plates of the calcaneus in the heel) in one or both heels and lower lumbar spine stress such as pars defect. The altered ankle stiffness of these two gymnasts in combination with the reported injuries indicates that biomechanical tests of stiffness may have potential for monitoring injury risk, especially during peak growth years.
Risk factors and injury prevention strategies to reduce biomechanical load
Injury prevention programs should place emphasis on the landing phase of gymnastics skills, as this phase seems to be critical to injury causation. Impact attenuation describes how efficiently the energy from an impact during landing is absorbed. Insufficient impact attenuation is linked to an increased injury risk as a result of overloading in tissues.
Landing techniques
Uncontrolled or repetitive landings can result in acute or overuse injuries. Therefore, there are important principles (e.g., segment alignment, attenuating forces over time, and using a large base of support) related to landings that gymnastics coaches can teach to young gymnasts. An upright trunk posture, reduced knee and hip flexion, unusual foot placement, and increased leg stiffness are potential contributing factors for injury during landings. Aiming for a “soft” (or quiet) landing means that attenuation of energy will help to reduce injury risk. Both technique and physical preparation for controlled landings should be taught at a young age, practiced often, and continued throughout athletes’ participation in the sport such as described in all editions of Canadian Level 1 coach certification manuals since 1975 and more recently in the 2009 FIG Foundation’s coach education manual. Injury prevention strategies should focus on neuromuscular training and core stability programs in the off-season and preseason conditioning to enhance proper landing biomechanics.
Safety equipment
Improved gymnastics equipment, in the form of sprung floors, sprung beams, thicker landing mats, and fiberglass rails has offset the expected decrease in injury incidence by enabling the performance of increasingly complicated and risky performance routines. Poorly maintained or positioned safety equipment is implicated in some injuries such as spraining an ankle by landing between badly aligned mats.
Marshall et al. (2007) reported from analysis of collegiate women’s gymnastics injuries over 16 years, the rate of injury in competition was more than two times higher than in practice (15.19 versus 6.07 injuries per 1000 athlete-exposures; rate ratio = 2.5, 95% confidence interval [CI] = 2.3, 2.8). A gymnast was almost six times more likely to sustain a knee internal derangement injury in competition than in practice (rate ratio = 5.7, 95% CI = 4.5, 7.3). It is understood that USA college female gymnastics represents a unique population in the world of gymnastics and these results therefore should not be generalized to other gymnastics populations. These high rates of injury during competition have led to some suggestions of increasing the thickness of landing mats used in competitions. Identification of the most important characteristics of gymnastics mat design for injury reduction has been gained from modeling impact energy absorption of surfaces and quantifying the response of gymnastic sports surfaces to dynamic loading. A seven-link wobbling mass model was used (Mills et al., 2010) to investigate how changes in the material properties of a multilayer model of a gymnastics competition landing mat could minimize ground reaction forces and internal loading of a gymnast during landing. Minimal changes to the mat’s stiffness (0.5%) but increased damping (272%) compared to a competition landing mat resulted in reduced peak vertical and horizontal ground reaction forces (12%) and reduced bone bending moments (6%) in the shank and thigh compared to a matching simulation. Design of landing mats to allow better damping of forces whilst retaining landing stability should be a focus for future research.
Training conditions
There is epidemiological evidence that sudden onset injuries occur more frequently relatively early or late in training sessions or when doing familiar skills (i.e., possibly due to inattention) (Caine et al., 1996). Training sessions should therefore involve more frequent apparatus changes to decrease the likelihood of a gymnast becoming inattentive. A number of studies across the gymnastics sports have reported increased injury rates for specific training periods. Coaches need to be aware of increased risk of injury when there is an increase in training intensity, increased anxiety (e.g., during periods immediately prior to competition, during competition, when learning new skills, or performing underprepared routines), increased levels of fatigue, or when there is less protection (competing without spotting and extra safety landing pads).
There is some controversy in the literature regarding the effect of skill level on injury. Caine et al. (2003) reported that the relative risk of injury was 1.47 times greater in the advanced versus beginning level American gymnasts. However, Kolt and Kirby (1999) reported that the rate of injuries per 1000 training hours was increased for subelite Australian gymnasts (4.11 per 1000 hours) compared with elite gymnasts (2.63 per 1000 hours). It is possible that reported injury rate differences are due to data collection differences used in the Australian and American studies. As gymnasts reach a higher level of competition, they perform more complex (and risky) maneuvers. Elite gymnasts may be more at risk of being pressured to return to full training too early after injury because of the level they are performing at, and where there are greater implications for time loss from training and competition. Those gymnasts are therefore at heightened risk of reinjury or compensatory injuries from altered biomechanics due to their incomplete rehabilitation.
Research on men’s and women’s gymnastics events show that most injuries occur on the floor, however, studies are required that include event-specific exposure time as the injury rate denominator. It is unclear whether the number of injuries on the floor is due to the nature of floor exercises themselves (e.g., repetitive trauma from tumbling) or because more time is spent on the floor, or a combination of the two.
Physique
Biomechanical efficiencies may be gained with particular physiques. Decreased height and weight elicit a greater ratio of strength to weight, greater stability, and a decreased moment of inertia. Body fat adds to mass without adding to power producing capability, therefore fat mass is detrimental to the gymnast. There can be increased risk of injury during periods of rapid body growth due to increased moments of inertia, increased muscle-tendon tightness, and decreased physis strength. Female artistic gymnasts who are mature and have a physique characterized as relatively tall with high lean body mass are at a greater risk for developing positive ulnar variance in the wrist (Claessens et al., 1996).
Musculoskeletal dynamic screening
Incorrect biomechanics during movement patterns can increase inappropriate loading. There are a number of screening protocols that can help identify movement dysfunction or technique faults. A whole-body functional movement competency screen that challenges fundamental movement patterns of the BW squat, the BW lunge and twist, the push-up, the BW bend and pull, and the single-leg squat, has been developed for use by sport and health professionals. The coach video records the athlete performing five repetitions of each movement task from the front and side, and then rates segment movement quality for the head, shoulders, lumbar spine, hips, knees, ankles, and feet, then rates quality of balance and joint range of movement. Initial evidence has shown movement competency screen scores can predict trunk injury for male and female elite athletes, however there is no evidence yet for gymnasts. Having screening movement tasks that can help predict performance or injury will enable coaches to monitor and prescribe exercises for gymnasts to enhance their performance and reduce their injury risk.
The importance of lower extremity dynamic malalignment in many lower extremity injuries (Willson & Davis, 2009) emphasizes the need to be able to screen gymnasts at least annually (more regularly during peak growth periods). There is an increasing body of research that is showing the use of biomechanics of functional movements to improve musculoskeletal screening. Dynamic alignment of the lower limb resulting in knee valgus may predispose the gymnast to ACL injury. Patella femoral syndrome is initiated by factors including weakness in the medial most quadriceps muscle, tightness in the lateral most quadriceps muscle, and weakness within the hip flexor, extensor, and adductor muscles. Other technologies beside video analysis may be used by biomechanists for screening. Achilles strain measures using ultrasonography during a ramped protocol with three different loads of isometric heel raises may provide a potential screening tool. Aside from profiling physical performance qualities, biomechanical (kinetic) testing has the potential to also aid in the assessment and management of the athlete’s functional musculoskeletal health (rehabilitation, injury risk). Investigation is needed to determine reliable functional tests that closely mimic gymnastics movements.
Further research
Although theoretically biomechanics can be used to help prevent injury via monitoring training forces, or predicting injury risk via musculoskeletal screening and technique analysis, there have been few studies that actually provide evidence of effectiveness of injury prevention intervention strategies based on biomechanical analysis (Bradshaw & Hume, 2012). Biomechanics research on gymnastics has predominantly focused upon the high-performance participation levels, with quantitative descriptions of specific skills. Greater focus for injury prevention is required on the elementary years of gymnastics when the fundamental motor skills of jumping and landing are being formed and also on the lower to middle competitive levels that involves the bulk of participants and similar rates of injury. Key strategies that maximize technique and safety need to be identified. Biomechanics can potentially help improve gymnastics performance and reduce injury risk to provide positive participation and competition experiences.
Computer simulation forward modeling exhibits promise for influencing coaching and training practice. This is because coaches with gymnasts seeking the epitome of competition performance are always seeking to know how to push skills further, such as the number of somersaults and/or twists that can be successfully performed on floor or vault. Simulation of the aerial skiing twisting performance is a relevant example of how coaches may be assisted by modeling research in gymnastics. Knowledge of take-off velocity and technique required to accomplish a quadruple twist for gymnastics skills would allow a coach to develop a gymnast safely through skill progressions to ensure that the final technique could be achieved.
From our review of the literature we can say that monitoring training loads should help decrease the risk of injury. Small, portable, and cost-effective means of assessing biomechanical loads on gymnasts are promising. Until practical validated methods are available, it is suggested that given the high magnitude of impact load (both acute and accumulative), coaches should monitor impact loads via the number of impact landings per training session, taking into consideration the height from which the landing is made and the control of body rotation during landing. Peak growth should be identified by tracking the gymnast’s peak height velocity given the biological versus chronological age differences and understanding how biological age can affect biomechanics and skill development. Prospective multifactorial risk analyses for elite gymnasts aged 10–14 years should be conducted. Studies could include assessment of strength and power and training loads, musculoskeletal stiffness and alignment, growth and development, bone health, and a nutrition profile. The effectiveness of gymnasts with a previous history of ankle sprain using an ankle brace to decrease the risk of recurrent injury should be determined. There is evidence from other sports that ankle taping is not effective after a very short period of intensive training or competition (Hume & Gerrard, 1998), therefore this issue needs to be researched in gymnasts.
Summary
Large impact forces, in combination with poor lower limb geometry during landings, in gymnastics are resulting in injuries predominantly to the lower limbs. Using functional movement screening, biomechanically instrumented equipment (e.g., beat board, rings, and bars), or instrumentation on the gymnast (e.g., accelerometers) to monitor gymnasts’ motion, and equipment and techniques to help reduce biomechanical loading should be useful. Given the high magnitude of impact load, both acute and accumulative, coaches should monitor impact loads in training, considering quality and quantity such as control of rotation and heights from which landings are executed. Evidence-based injury prevention interventions should be developed for gymnastics focused on reducing biomechanical loading during landings.
References
Bahr, R. and Krosshaug, T. (2005) Understanding injury mechanisms: a key component of preventing injuries in sport. British Journal of Sports Medicine, 39, 324–329.
Beatty, K.T., McIntosh, A.S., and Frechede, B.O. (2005) Measurement of impact during gymnastics skills. British Journal of Sports Medicine, 39, 374.
Bradshaw, E. and Hume, P.A. (2012) Biomechanical approaches to identify and quantify injury mechanisms and risk factors in women’s artistic gymnastics. Sports Biomechanics, 11 (3), 324–341.
Brueggemann, P. (2010) Neuromechanical load of biological tissue and injury in gymnastics. In: R. Jensen, W. Ebben, E. Petushek, C. Richter, and Roemer, K. (eds), XXVIII International Symposium of Biomechanics in Sports 2010. Department of Health Physical Education and Recreation, College of Professional Studies, Northern Michigan University, International Society of Biomechanics in Sports, Marquette, MI.
Burt, L., Naughton, G., and Landeo, R. (2007) Quantifying impacts during beam and floor training in pre-adolescent girls from two streams of artistic gymnastics. In: H.J. Menzel and M.H. Chagas (eds), XXV International Symposium on Biomechanics in Sports 2007. Federal University of the State of Minas Gerais in Belo Horizonte, International Society of Biomechanics in Sports, Ouro Preto, Brazil.
Caine, D., Knutzen, K., Howe, W., Keeler, L., Sheppard, L., Henrichs, D., and Fast, J. (2003) A three-year epidemiological study of injuries affecting young female gymnasts. Physical Therapy in Sport, 4, 10–23.
Caine, D.J., Daly, R.M., Jolly, D., Hagel, B.E., and Cochrane, B. (2006) Risk factors for injury in young competitive female gymnasts. British Journal of Sports Medicine, 40, 91–92.
Caine, D.J., and Lindner, K.J. (1985) Overuse injuries of growing bones: the young female gymnast at risk?. Physician and Sports Medicine, 13, 51–64.
Caine, D.J., Lindner, K.J., Mandelbaum, B.R., and Sands, W.A. (1996) Gymnastics. In: D.J. Caine (ed.), Epidemiology of Sports Injuries. Human Kinetics Publishers, Champaign, IL.
Claessens, A.L., Lefevre, J., Beunen, G., De Smet, L., and Veer, A.M. (1996) Physique as a risk factor for ulnar variance in elite female gymnasts. Medicine and Science in Sports and Exercise, 28, 560–569.
DiFiori, J., Caine, D., and Malina, R. (2006) Wrist pain, distal radial growth plate injury, and ulnar variance in the young gymnast. American Journal of Sports Medicine, 34, 840–849.
Emery, C.A. (2010) Injury prevention in paediatric sport-related injuries: a scientific approach. British Journal of Sport Medicine, 44, 64–69.
Hawkins, D. and Metheny, J. (2001) Overuse injuries in youth sports: biomechanical considerations. Medicine and Science in Sports and Exercise, 33, 1701–1707.
Hume, P.A. and Gerrard, D.F. (1998) Effectiveness of external ankle support: bracing and taping in rugby union. Sports Medicine, 25, 285–312.
Koh, T.J., Grabiner, M.D., and Weiker, G.G. (1992) Technique and ground reaction forces in the back handspring. American Journal of Sports Medicine, 20, 61–66.
Kolt, G.S. and Kirby, R.J. (1999) Epidemiology of injury in elite and subelite female gymnasts: a comparison of retrospective and prospective findings. British Journal of Sports Medicine, 33, 312–318.
Kovacs, R., Santha, A., Balajti, N., Martos, E., and Balogh, E. (1998) Overuse changes of distal radial epiphysis in professional gymnasts. Hungarian Review of Sports Medicine, 39, 171–180.
Kujala, U.M., Taimela, S., Oksanen, A., and Salminen, J.J. (1997) Lumbar mobility and low back pain during adolescence: a longitudinal three-year follow-up study in athletes and controls. American Journal of Sports Medicine, 25, 363–368.
Marshall, S.W., Covassin, T., Dick, R., Nassar, L.G., and Agel, J. (2007) Descriptive epidemiology of collegiate women’s gymnastics injuries: National Collegiate Athletic Association Injury Surveillance System, 1988-1989 through 2003–2004. Journal of Athletic Training, 42, 234–240.
McNitt-Gray, J.L., Hester, D.M.E., Mathiyakom, W., and Munkasy, B.A. (2001) Mechanical demand and multijoint control during landing depend on orientation of the body segments relative to the reaction force. Journal of Biomechanics, 34, 1471–1482.
Meeusen, R. and Borms, J. (1992) Gymnastic injuries. Sports Medicine, 13, 337–356.
Meeuwisse, W.H., Tyreman, H., Hagel, B.E., and Emery, C.A. (2007) A dynamic model of etiology in sport injury: the recursive nature and risk of causation. Clinical Journal of Sports Medicine, 17, 215–219.
Mills, C., Yeadon, M.R., and Pain, M.T.G. (2010) Modifying landing mat material properties may decrease peak contact forces but increase forefoot forces in gymnastics landings. Sports Biomechanics, 9, 153–164.
Moresi, M., Bradshaw, E., Greene, D., and Naughton, G. (2010) Jump kinetics, bone health and nutrition in elite adolescent female athletes. 28th International Conference on Biomechanics in Sport, Marquette, MI.
Willson, J.D. and Davis, I.S. (2009) Lower extremity strength and mechanics during jumping in women with patellofemoral pain. Journal of Sport Rehabilitation, 18, 76–90.
Recommended reading
Butler, R.J., Cowell, H.P., and McClay Davis, I. (2003) ‘Lower extremity stiffness: Implications for performance and injury’. Clinical Biomechanics, 18, 511–517.
Kolt, G., and Caine, D. (2010) Gymnastics. In: D. Caine, P. Harmer, and M. Schiff (eds), Epidemiology of Injury in Olympic Sports. Encyclopaedia of Sports Medicine. Wiley-Blackwell (England) and the International Olympic Committee (IOC) Medical Commission.
Krosshaug, T. and Verhagen, E. (2010) Investigating injury risk factors and mechanisms. In: E. Verhagen and van Mechelen (eds), Sports Injury Research. Oxford University Press.
Norcross, M.F., Blackburn, J.T., Goerger, B.M., and Padua, D.A. (2010) ‘The association between lower extremity energy absorption and biomechanical factors related to anterior cruciate ligament injury’. Clinical Biomechanics, 25, 1031–1036.
Soriano, P.P., Belloch, S.L., and Alcover, E.A. (2006) Partial implementation of the Q.F.D. methodology for the identification of the most important characteristics and features of Gymnastics mats design. International Journal of Applied Sports Sciences, 18, 65–77.
< div class='tao-gold-member'>
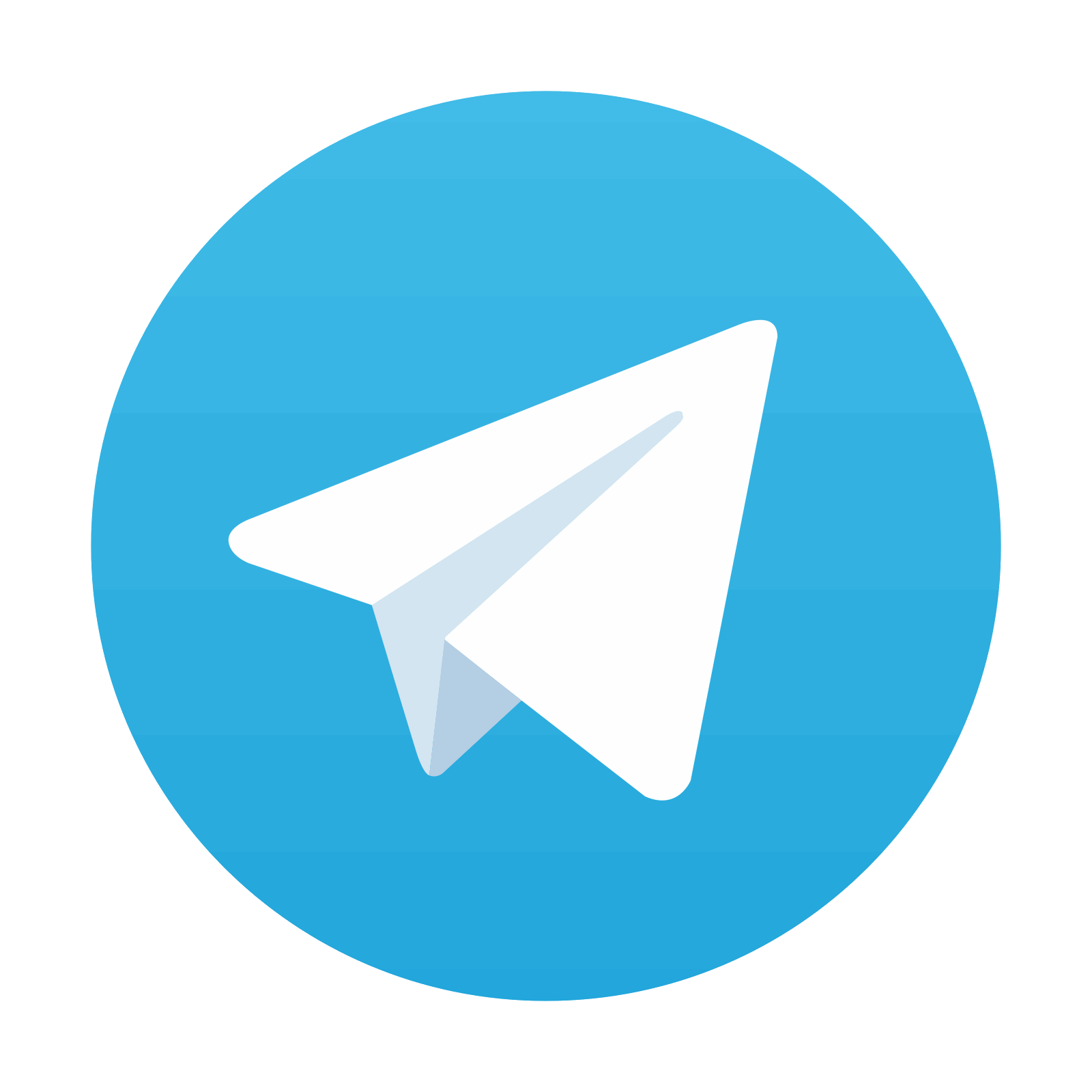