In Artistic Gymnastics, mechanical load acts on the biological response matrix in a single or a multiple series over seconds, hours, days, weeks, and years. The mechanical exercise stimulus (MES) is characterized by the loading amplitude, the loading rate, the frequency and the duration of loading, and also by the pauses between the loading sequences. The biological tissue response matrix (TRM) is determined by the genotype, the tissue architecture, the tissue age, gender, pre-injuries, mechanical tissue properties, the tissue fatigue, the hormonal tissue status, and other factors. Due to the early onset of training in gymnastics and therefore the increased mechanical loading at an early age, the tissue is extremely sensitive to overuse in young gymnasts. During growth spurts the potential of tissue to withstand load bearing is temporarily decreased, which makes an appropriate load management most difficult in gymnastics.
If the MES is excessive, such loading has the potential for acute morphological and physical tissue damage (ATD), which means an acute failure of the tissue involved. If the MES is within the limits of the response matrix potential, the mechanical stimulus results in a signal transduction (ST) at the cellular level or—if the loading stimulus is not appropriate in terms of amplitude, frequency, or even direction—in an increased cell death (CD) within the tissue under mechanical load. Assuming that the input signal to the response matrix is appropriate in terms of amplitude, direction, frequency, and intensity, ST will increase the cellular activation, which leads to tissue adaptation (TAD) on the cellular level as well as of the extracellular matrix. This is of relevance because the extracellular matrix defines and determines the mechanical properties of the tissue and is strongly related to mechanical strength of the given tissue. From this basis one can derive that tissue strength adaptation (TAD) is a function of the mechanical stimulus (MES), the response matrix (TRM), and the ST process. TAD is the source of tissue matrix change and therefore of tissue strengthening.
Tissue strength enhancement is the major concern in the physical preparation of the young gymnast allowing the tissue to withstand the extreme forces and torques in modern gymnastics. The key factor for minimizing the risk of long-term overuse is the appropriate loading of the biological structures guaranteeing TAD and strengthening. Consequently, prerequisites of load management in gymnastics’ training are a sound knowledge of mechanical loading in gymnastics (MES) and a profound background of the TRM, which determines the tissue’s critical strength limits. The weakness of the model is the limited knowledge of the individual characteristics of the determinants of the response matrix.
Acute and overuse tissue failure in gymnastics
ATD in Artistic and Trampoline Gymnastics results from falls on the ground, on the mats, and on the apparatus (trampoline, horizontal bars, asymmetric and parallel bars). Catastrophic injuries from direct falls with severe consequences to the spinal cord are infrequent. However, according to reports from the US National Center for Catastrophic Sports Injuries, the occurrence of this type of injury appears to be high in comparison to other sports. Acute injuries from direct falls affect the biological structures of feet, the ankle and the knee joints, the spine, the thoracic cage, and the elbows, wrists, and fingers. The mechanical energy applied to these biological structures at the collision instantaneously exceeds the actual tissue strength limits and leads to failure.
The main concern in gymnastics is overuse injury or overuse tissue failure, which is the result of frequent mechanical stimuli close to the ultimate tissue strength limits. In comparison to other sports, overuse injury rates in elite gymnastics and especially in artistic gymnasts are relatively high (Froehner, 2000). The foot, ankle, and knee joints and the spine are the most commonly injured locations. In male gymnastics the shoulder and the wrist are also ranked high in the injury frequency tables.
Studies of risk of injuries in gymnastics typically represent a broad range of performance levels, age groups, training systems, and related mechanical loading. Few retrospective studies on former elite athletes report chronic injuries and especially spinal radiological deformities of elite gymnasts (Pollaehne, 1991). Other retrospective studies (e.g., Tertti et al., 1990) did not identify any major differences in early tissue degeneration between gymnasts and nongymnasts. However, many of the retrospective studies do not demonstrate results from top or elite subjects but those from subelite or recreational athletes. Froehner (2000) reported deformities and disorders of the spinal structures and the peripheral joints of 42 female and 27 male former top German gymnasts (all participated in Olympic Games, World Championships, or European Championships) 8.9 (±5.2) years after retirement from competitive Artistic Gymnastics.
Close to 10 years after their career, more than 90% of the male and female former gymnasts suffered from moderate or severe disorders of the spine, 40% of knee and ankle joints, and more than 30% of the male gymnasts of shoulders, elbows, and wrists. Severe discomfort of the spine was demonstrated in 57% of the female gymnasts (21% cervical spine, 17% thoracic spine, 52% lumbar spine) and in 58% of the male gymnasts (23% cervical spine, 19% thoracic spine, 50% lumbar spine). In 32 female former elite gymnasts Froehner (2000) identified structural changes and deformities in 42 vertebrae of the thoracic spine and in 21 vertebrae of the lumbar spine, which gives an average of two affected vertebrae per gymnast. The numbers for the male gymnasts are even higher (58 thoracic, 21 lumbar) with 3.76 affected vertebrae per gymnast. The highest frequency of structural changes of the former athletes is found in the thoracic-lumbar junction.
Spinal loading above the critical limits in regard to loading amplitude, loading rate, frequency, and duration resulting in deformation and structural changes might appear in Artistic Gymnastics especially in the thoracic-lumbar junction and more in female gymnastics in the lumbar region. Repeated hyperextension of the lumbar spine mainly in female Artistic and Rhythmic Gymnastics is assumed to pose a major risk to the lumbar spine and especially the pars interarticularis. However, a 3-year survey of elite German female gymnasts (Brueggemann & Krahl, 2000) (n = 135; age: 12–21 years) reports a frequency of slip instability (spondylolysis, retrolisthesis, pseudolisthesis) in 15 of 135 (11%) individuals, which is in the range of the normal population. A spondylolythesis was identified in 2.9% of the examined gymnasts. This frequency does not differ from that of a nonathletic population. The difference of these findings to earlier reports could be related to changes in the nature and type of the mechanical loading in female gymnastics. The repeated trunk flexion when landing from various heights or during take-off from the spring floor or the springboard may create compression forces sufficient to damage the anterior aspect of the vertebral endplate in the thoracic-lumbar region and disrupt further growth at this side.
Froehner’s unique data demonstrate the knee and the ankle joint at second highest risk. From the 42 former female gymnasts 28% suffered from ankle joint disorders and 24% from knee-related discomfort. Among male athletes the knee showed 27% disorders and the ankle joint 15%. Upper extremities disorders were more common in the male subjects than in the female gymnasts. This can be explained by the different events and apparatus in male and female gymnastics. The females perform on three (floor exercise, balance beam, vault) of four disciplines that are intuitively prone to load the lower extremities, while the male gymnasts have only two (floor exercise, vault) of six apparatus. The total loading time of the lower extremity and the number of lower extremity loading repetitions are definitively higher in female than in male Artistic Gymnastics. Loading frequency and total loading time appear in combination with the loading amplitude as key determinants of the MES in Artistic Gymnastics.
Mechanical loading of biological structures in gymnastics
Measurement of mechanical loading
Two principal approaches are applied to study mechanical tissue load in gymnastics and other sports: (1) direct measurements and (2) indirect methods. While the direct techniques use sensors and transducers based on different technical principles and provide data describing variables directly connected to mechanical tissue loading, the indirect methods provide a compromise of mathematical models allowing a calculation or an estimation of loading-related variables on or within a biological tissue. Direct measurements are extremely seldom in gymnastics (exceptions are direct tendon force measurements in gymnastic like jumping and hopping) due to ethical and technical restrictions. The latter do not allow unhindered or nonrestricted movements for the gymnast, which is a prerequisite to approach realistic loading data. The modeling techniques applied to gymnastics used multibody models with an inverse dynamic approach and distribution models, which distribute the resultant joint moments and forces to load carrying structures and allow an estimate of mechanical load transmitted to specific tissue (bones and articular cartilage, intervertebral discs, muscle-tendon units, ligaments). Due to the fact that the mathematical models used for estimations of mechanical tissue loading have more unknowns than equations these approaches are underdetermined. Additional assumptions (e.g., tissue material properties, joint surface areas, and lever arms of ligament or tendons) must be taken into account and static optimization has often been used to minimize the solution space. It is obvious that the numerical solution is strongly related to the cost function used to solve the problem. Therefore, model outcomes have to be interpreted carefully and results of different models cannot be (quantitatively) directly compared. The data presented in this chapter are all derived from the same model and therefore comparable. The kinematic model input data are from three-dimensional (3D) motion analyses, while the kinetic data are taken from direct measurements of reaction forces on the ground (ground reaction forces) or on the apparatus (rings, horizontal bar, asymmetric bars, pommel horse, vaulting table, balance beam).
The measurement of the optic fiber transducer, which was also applied in gymnastics hopping and jumping and implanted in the Achilles and Patellar tendons (Brueggemann et al., 2000), is based on modulation of light intensity by mechanical modification of the geometric properties of the plastic fiber that is inserted into the tendon. The structures of optical fibers used in animal and human experiments consist of two layered cylinders of polymers with small diameters. When the fiber is bent or compressed the light can be reduced linearly with pressure. The fiber can be inserted into the tendon or ligament with help of a hollow needle that is first passed through the tendon or ligament. The sterile optic fiber is then passed through the needle. The needle is removed and the fiber remains in situ. Both ends of the fiber are then attached to the transmitter–receiver unit. The calibration usually gives a good linear relationship between external force and optic fiber signal.
The direct methods are invasive or are often restricted to static positioning of the subject and have therefore limited application to dynamic movements and especially high-impact physical activities in gymnastics. In Artistic Gymnastics direct measurements are infrequent, whereas model-based estimations allow a first insight view into tissue loading and at least a relative comparison of loading within the spectrum of skills. Notably, the model-based quantities are conservative estimations by nature due to the chosen cost function. The real mechanical tissue loading will be even higher than the model-based estimates.
Mechanical exercise stimulus in gymnastics
As mentioned above, an increase in gymnastics skill difficulty is associated with the demand for higher mechanical energy. The further increase of mechanical energy results in higher mechanical stress on and in the biological structures. This increasing mechanical loading impacts primarily (a) the most distal joints of the kinematic chain with the hands/elbows/shoulders or the lower extremities (feet, ankle, and knee joints) and (b) the spinal structures. Due to the increased frequency of loading and the more frequent loading schedule one has to consider not only the ultimate tissue tolerances but also the modification of tissue tolerance limits in repeated loading regimes.
Spinal loading
The major and most dramatic clinical and radiological findings in gymnastics concern the thoracic-lumbar junction and the lumbar spine. There is controversy in the discussion about which skill or drill in gymnastics produces the highest loading in regard to the thoracic-lumbar spine. One point discussed as a cause for the growth disturbances in the ring apophysis was the fast changes between flexion and extension of the thoracic-lumbar junction during the swings on the rings and horizontal bar. Some authors considered the damage at the ring apophyses in gymnasts as a flexion trauma and speculated that this could be caused by incorrect landing. This controversy led to the inclusion of gymnastic landings and long swings combined with dynamic flexion and extension of the thoracic-lumbar spine in the data acquisition to quantify spinal loading. The compressive forces at Th12/L1 in gymnastic landings are significantly higher than, for example, in running and showed the highest amplitudes compression forces of all the studied skills. Landing from a dismount (from the rings, horizontal bar, asymmetric bars) results in net compression forces to L5/S1 and therefore to the intervertebral disc of more than 11.6 times bodyweight (average force) in the first 50 milliseconds of ground contact with peak forces up to 20 times bodyweight. These resultant force data are calculated by the distribution model based on ground reaction force measurements and 3D kinematic data. Shear force to the disc at L5/S1 is estimated at 3.5 times body weight. These numbers give a compressive force to the segment L5/S1 (and the disc) for a 65 kg gymnast while landing from a dismount of at least 7500 N and a shear load to the L5/S1 disc and the pars interarticularis of >2000 N.
The few cadaver studies of young specimens loaded in a physiological environment (e.g., Genaidy et al., 1993) documented maximum compressive strength for young male adults of <8000 N and ultimate shear force tolerance of a lumbar motion segment of <3000 N. About the same loading amplitude with a little low loading rate was calculated for the motion segments of the lumbar-thoracic junction. These numbers indicate that landing in gymnastics gives tissue mechanical loading values close to the critical tissue tolerances. Vertebral deformities and growth disturbance of the anterior column of vertebrae at lumbar-thoracic junction shown (Froehner, 2000) can be explained by increased CD due to excessive loading and the related disturbed TAD. This mechanism is of major concern during growth and the higher vulnerability of the musculoskeletal system of the young gymnasts.
As expected, the compression forces increase significantly with increasing kinetic energy at touchdown and therefore with the height of the flight prior to landing. The shear forces are more or less constant with increasing height of the flight. When landing from a somersault the gymnast has to manage the linear and the angular momentum from the flight. This is related to an increased forward lean and trunk flexion. The resultant compression and shear forces at Th12/L5 gain 130–150%. Initial energy at touchdown, body position at landing, and body’s angular momentum prior to landing determine the compression and shear force at the thoracic-lumbar junction and the lumbar portion of the spine in gymnastic landings.
The use of the supplementary mats demonstrates the impact of technical aids to control maximum compression and shear forces when landing from a dismount. Compressive forces during landing can be reduced to 80% by thin supplementary layers on top of the landing mats or the spring floor. Landing from a vault with a horizontal momentum close to the vertical momentum can be assumed to be related with similar or even higher spinal loading than in landing from an apparatus dismount. During the take-off from the spring floor or the springboard spinal loading appears to be lower than for landing from a flight. Maximum compression forces at L5/S1 level are estimated at 6.5–8.5 times body weight and maximum shear loading at 3.0–3.5 times body weight. This brings the take-offs on second place in the spinal loading listing. On third place of the spinal loading list rank the jumps, landings, and artistic skills on the balance beam. The cumulative spinal loading from training on the balance beam should not be underestimated given the extensive time spent and the high frequency of skills and repetitions on this apparatus.
The giant swings (e.g., in the scooped technique) on the horizontal bar and asymmetric bars, prior to dismount and flight elements with regrasp are expected as high loading demands for the spine. Compression and shear forces were calculated over the entire skills. The data demonstrate that the spinal loading is a compressive type loading during the whole drill. Muscle forces are higher than the inertial forces and compression and shear forces are counterintuitively low in comparison to the above presented activities. The long swings on rings, horizontal bar, and asymmetric bars show only moderate spinal loading. Even scooped giant swings and flight elements with regrasp are related to maximum lumbar-thoracic junction compression load of <4.5 times body weight and shear forces of <3.0 times body weight. Notably, these values represent valid and successfully performed trials. No data are available from failed movements. Health problems due to swings and giants should be explained by different overloading mechanisms rather than compression and shear loading to vertebrae and discs. Hyperextension with a high tensile strain on the anterior vertebral ligament and high tensile stress on the insertion to the vertebra may explain tissue failure.
Foot and ankle loading
The Achilles tendon is paradoxically the strongest tendon of the human body and it is frequently affected by acute and overuse injuries in gymnastics. Using cine phase-contrast magnetic resonance imaging (MRI), it was recently demonstrated that the free Achilles tendon strain is clearly higher than that of the aponeurosis (Finni et al., 2003). This observation suggests that the free Achilles tendon is more compliant than the aponeurosis, which gives the free tendon the higher potential to store elastic energy. At the same time the more compliant free tendon is at a higher risk for injury. In single-leg jumping, peak tendon tensile stress up to 11 MPa have been measured in vivo using buckle transducer or optic fiber techniques. Figure 6.2 demonstrates the Achilles tendon force measured with the optic fiber device in a drop jump take-off for a forward somersault from an elastic gymnastic spring floor. Maximum load is in the mid-stance that corresponds with the peak ground reaction force. Achilles tendon force reaches a maximum tensile force of >5000 N. The loading rate is 100 kNs-1, which is high in relation to single-leg jumping measures.
Figure 6.2 Achilles tendon force (black) and vertical ground reaction force (gray) of a drop jump take-off for a forward somersault from an elastic gymnastic spring floor. The vertical solid line gives the first contact of the feet with the ground; the dashed line indicates the last contact with the ground.
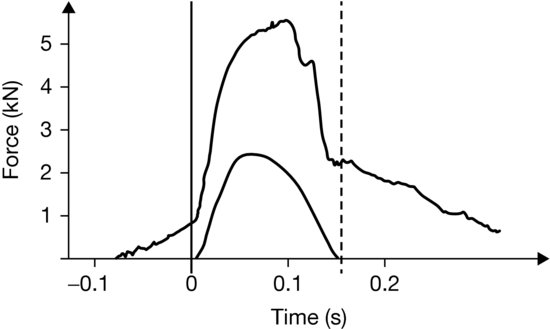
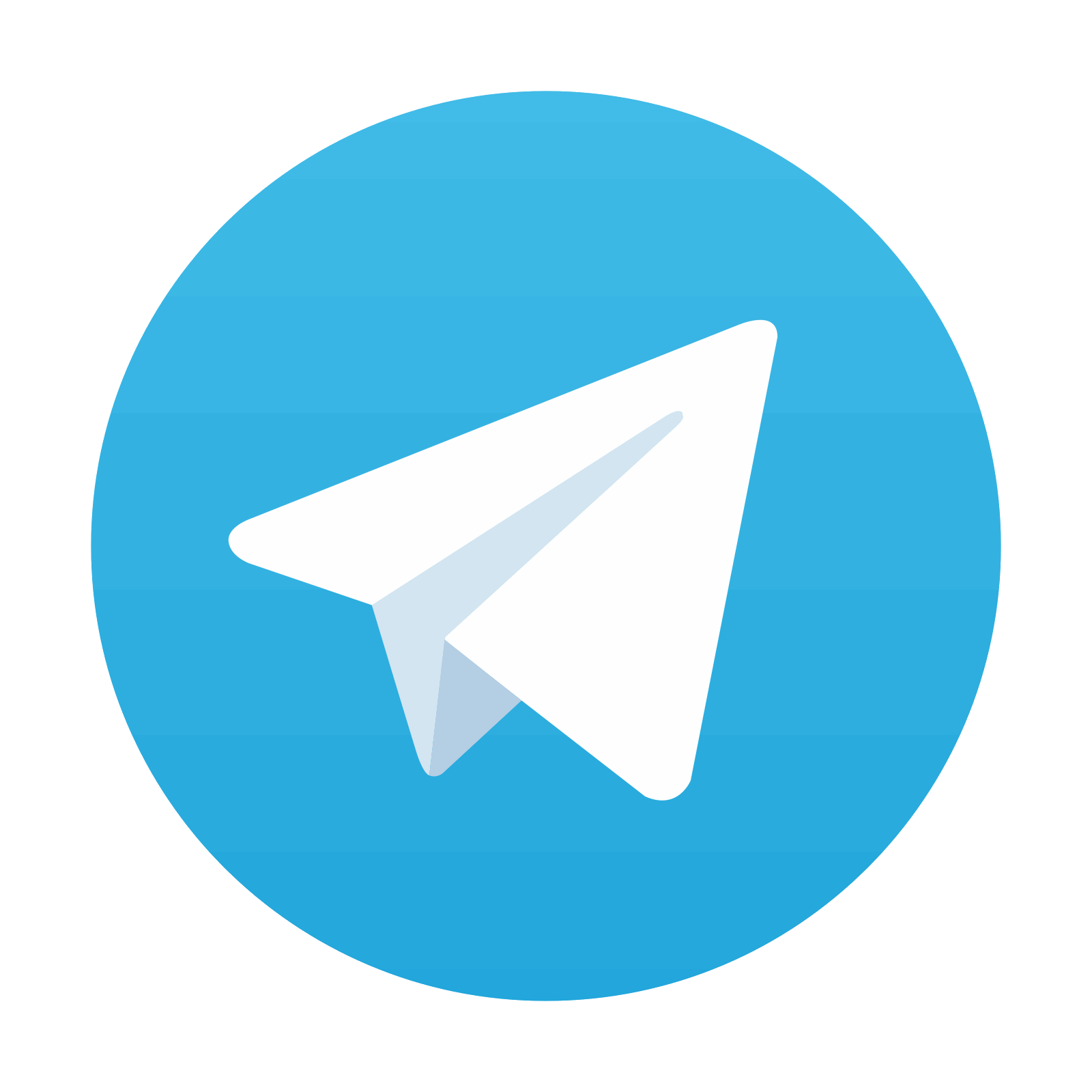