34 Biologic Markers
Uniform definitions of disease are essential for biomarker validation and comparison among studies.
The validation process of a biomarker depends on the specific purpose of its use.
Panels of biomarkers or biomarker profiles are potentially more powerful than single biomarkers.
Biomarkers are anatomic, physiologic, biochemical, or molecular parameters associated with the presence and severity of specific diseases and are detectable by a variety of methods including physical examination, laboratory assays, and imaging. Here the focus is on biomarkers in senso stricto: markers that can be measured in patient samples such as blood, urine, synovial fluid, and synovial tissue. Such molecules often first appear in studies on disease mechanisms, their application as biomarkers being secondary. Realizing the importance and potential of biologic markers, the identification, characterization, and validation of novel biomarkers is often a primary objective in many current studies. Biomarkers are applied for various purposes including diagnosis, prognosis, monitoring of disease progression, selection of patient populations in clinical trials, assessment of the efficacy of treatment, or unraveling of the pathobiology of a disease in the clinical and in a preclinical setting. More recently, biomarker validation level-of-evidence schemes have been proposed to optimize efficient use of biomarkers in these diverse research areas.1,2
For osteoarthritis and rheumatoid arthritis (RA), the most important common feature is progressive destruction of articular tissues, resulting in impaired joint function and pain. Diagnosis is based on clinical symptoms and laboratory tests in combination with radiography, to visualize often irreversible degenerative and destructive changes in the joint. Radiologic evaluation of joints mainly images bone and is relatively insensitive: A follow-up period of at least 1 year is necessary to assess disease progression and effect of therapy. Magnetic resonance imaging (MRI) has the ability to visualize all joint tissues simultaneously; it is currently being optimized3 but has yet not reached its full potential due to its relatively high cost and still limited availability.4 Except for imaging modalities such as positron emission tomography scans, which use labeled tracers that may be used to image an ongoing biologic process, most imaging techniques provide a cumulative historical view of damage that has already occurred, rather than assessing the current rate of disease progression (Figure 34-1).
Alternative methods that detect changes in the joints in an early stage of the disease in a quantitative, reliable, and sensitive manner are necessary. The World Health Organization in its report Priority Medicine for Europe and the World states that biomarkers are essential for arthritis in general and osteoarthritis research in particular, and that the lack of adequate markers constitutes a major hurdle for osteoarthritis drug development.5 No osteoarthritis biomarker underwent the formal approval procedure of the U.S. Food and Drug Administration (FDA) to be allowed as qualifying end point in a phase III clinical trial.6 Molecular markers (i.e., markers that are used to monitor molecular events occurring during disease) are well suited for this purpose. A good marker is disease specific, reflects actual disease activity, is sensitive to change after therapy, and can predict disease outcome. Most likely, all of these requirements are not met by a single marker: Different (combinations of) markers would have to be applied for different purposes. The fact that imaging techniques often focus on one joint (e.g., knee or hip) or joint group (e.g., hands, lumbar spine), whereas urine-derived or blood-derived biomarkers are determined by all of the joints in the body, further underscores the importance of using imaging and biomarker approaches as complementary tools. The molecular markers that are described for joint diseases can be arbitrarily classified as follows:
1. Immunologic and inflammatory markers (including acute-phase proteins)
2. Markers that reflect extracellular matrix remodeling (Table 34-1)
3. Markers present in synovial tissue biopsy specimens
4. “Omics”-based biomarkers (e.g., genomics, transcriptomics, proteomics, metabolomics)
5. Genetic markers that have been shown to provide important information on risk factors for development and progression of osteoarthritis and RA; these are reviewed elsewhere7–10 and are not included in this chapter.
Table 34-1 Molecular Markers That Reflect Extra Cellular Matrix Remodeling
Marker | Joint Tissue | References |
---|---|---|
Synthesis | ||
Collagen Type I | ||
N-propeptide (PINP) | Bone, soft tissues | 58 |
C-propeptide (PICP) | Bone, soft tissues | 58 |
Collagen Type II | ||
N-propeptide (PIINP; PIIANP) | Cartilage | 75, 102, 103 |
C-propeptide (PIICP; chondrocalcin) | Cartilage | 95, 100, 101 |
Collagen Type III | ||
N-propeptide (PIIINP) | Soft tissues | 74, 104–106 |
Proteoglycans and Glycosaminoglycans | ||
Chondroitin sulfate (epitopes 846, 3-B-3, 7-D-4) | Cartilage | 88, 95, 96, 112–116 |
Miscellaneous | ||
Bone-specific alanine phosphatase | Bone | 58 |
Osteocalcin | Bone | 59 |
YKL-40 (CYLK-40, gp-39, chondrex) | Cartilage | 58, 59 |
Hyaluronan | Cartilage, synovium | 77, 116, 122–127 |
Degradation | ||
Collagen Type I | ||
Cross-linked N-telopeptide (NTx) | Bone | 58, 59, 63, 64, 68–71 |
Cross-linked C-telopeptide (CTx) | Bone | 59, 64, 67–71 |
Cross-linked C-telopeptide (ICTP) | Soft tissues | 64, 66 |
Collagenase cleavage neoepitope (C1, 2C) | Bone, soft tissues | 89, 90 |
Collagen Type II | ||
Cross-linked C-telopeptide (CTx-II; 2B4 epitope) | Cartilage | 59, 73–83 |
Collagenase cleavage neoepitope (9A4, C2C, C1, 2C) | Cartilage | 86–92 |
Pyridinolines | ||
Hydroxylysylpyridinoline (HP, PYR) | Bone, cartilage | 94–96 |
Lysylpyridinoline (LP, D-PYR, DPD) | Bone | 95, 96 |
Glucosylgalactosyl-hydroxypyridinoline (GGHP) | Synovium | 75, 97 |
Proteoglycans and Glycosaminoglycans | ||
Aggrecan core protein (fragments) | Cartilage | 58, 118–121 |
Keratan sulfate (epitope 5-D-4, AN9P1) | Cartilage | 58, 88, 95, 96, 107–116 |
Chondroitin sulfate | Cartilage | 58 |
Miscellaneous | ||
Matrix metalloproteinases | Cartilage, synovium | 27, 30, 75, 138, 140–152 |
Aggrecanases | Cartilage | 117, 153–159 |
Cartilage oligomeric matrix protein (COMP) | Cartilage, possibly synovium | 74, 128–139 |
Bone sialoprotein (BSP) | Bone | 57, 96 |
Inflammatory Biomarkers and Signal Molecules
Extensively studied markers in inflammatory arthritis that are routinely used in clinical practice include acute-phase proteins such as C-reactive protein (CRP) and measurements such as the erythrocyte sedimentation rate (ESR), both of which provide information about the systemic inflammatory process. Although such markers of inflammation are neither disease specific nor tissue specific, they may reflect disease activity,11 reflect the effects of immunosuppressive and immunomodulatory therapies,12 and, to a certain extent, predict disease progression in RA.13 A more disease-specific marker for RA is the presence of anticitrullinated protein/peptide antibodies.14 It predicts with high probability the development of RA in patients with no clinical symptoms, distinguishes between RA and other rheumatic diseases, and is a valuable tool for prognostic prediction of joint destruction.15 Although synovial inflammation is often regarded as a secondary process in osteoarthritis, cytokines and other signal molecules have also been proposed as markers in this disease. In clinical studies, often highly sensitive analysis of CRP is included because it is associated with osteoarthritis of the knee16,17 and hip,18 but validation of this biomarker to monitor treatment efficacy is hampered by the lack of effective treatments (as is the difficulty with all osteoarthritis biomarkers).
Cytokines and Chemokines
RA patients often show elevated levels of a variety of cytokines, chemokines, and their receptors (for details see Chapter 26). Many of these molecules are subsequently used as disease biomarkers or studied as targets for intervention, analogous to the experience with tumor necrosis factor (TNF). This cytokine has been shown to play a pivotal role in arthritis and has emerged as a major therapeutic target. TNF inhibitors (neutralizing antibodies or soluble receptors) strongly reduce clinical symptoms, joint inflammation, and biomarkers of inflammation and bone destruction in RA patients.12,19,20 Neither the serum levels of the TNF receptors nor those of interleukin (IL)-10 were reduced, however, in response to treatment of RA patients with methotrexate and anti-TNF treatment, despite a clear improvement in clinical disease parameters and a reduction of CRP and ESR.21 In contrast, in synovial tissue, treatment with these drugs did reduce the local TNF level, indicating that for biomarker analysis it is important to select the right compartment.22–24 The observation that anti-TNF treatment may block joint destruction in patients who do not show a clinical response (i.e., ACR20 nonresponders) suggests that, similar to osteoarthritis, in RA uncoupling of inflammation and joint destruction may occur, which may affect the selection of biomarkers to monitor treatment effects. High levels of soluble receptors of TNF are associated with reduced physical function and worse radiologic knee osteoarthritis.25
IL-6 is another cytokine that has gained interest in the past few years as a therapeutic target in RA. Anti-IL-6 receptor antibody therapy in combination with methotrexate results in decreased RA disease activity, improved function, and reduced levels of joint destruction biomarkers.26,27 Synovial fluid IL-6 levels in RA patients are correlated with infiltration of inflammatory cells in the synovial membrane and with radiographic joint destruction.28,29 In a clinical trial of cytokine blockade, baseline serum IL-6 levels predicted radiographic progression, but not the ACR response, again indicating that inflammation and joint destruction are not necessarily coupled in RA.30 Systemic circulating IL-6 levels are well correlated with CRP levels, and therefore the added value of this biomarker seems to be limited for monitoring RA disease activity and response to treatment.31,32
Adipokines
The role of leptin in the pathogenesis of rheumatoid arthritis is still under debate.33 Increased systemic levels of leptin in RA patients compared with controls were observed, and a positive correlation among serum leptin concentration and disease activity, ESR, and the number of tender joints was demonstrated.34–36 In contrast, other studies demonstrated a link between leptin and body mass index and not with disease activity.37–40 In line with this observation is the fact that anti-TNF therapy did not reduce plasma leptin concentrations while inflammation decreased in the patients.41
Adiponectin is another well-known adipokine secreted by adipose tissue, and serum concentrations are inversely correlated with body mass index. In contrast to its protective role in obesity-linked diseases, adiponectin seems to fulfill a proinflammatory role in RA.42 Serum and synovial fluid adiponectin levels are significantly higher in RA patients than in healthy controls, and serum levels correlate with the severity of joint destruction.35,43 However, inconsistent data on the effect of anti-TNF therapy on circulating adiponectin levels exist, showing an increased level of adiponectin after therapy or no effect at all.41,44–48 As such, the value of circulating adiponectin for assessing anti-inflammatory therapy seems to be limited.
Other adipokines such as visfatin and resistin have been implicated in the pathogenesis of RA as well.49 Visfatin expression is not limited to adipose tissue, but synovial fibroblasts from RA patients also exhibit visfatin expression, especially in the synovial lining and at sites of invasion.50 A positive correlation between visfatin serum levels and clinical disease activity has been demonstrated; however, in another clinical study such a relation was not observed.50,51 Resistin expression is found in the sublining layer of the synovium and is more intensive in RA than in osteoarthritis patients. Increased circulating resistin levels in RA patients correlate with both CRP and disease activity.52,53
In osteoarthritis patients, serum nitrate and nitrite (reflecting nitric oxide levels) are increased compared with healthy controls, but not as much as in inflammatory conditions such as RA.54 In addition, using a genomics approach, chondrocytes from osteoarthritis cartilage have been shown to upregulate the transcription of a variety of inflammatory genes.55 Synovial tissue from osteoarthritis patients also shows signs of hyperplasia and inflammation.56 Although osteoarthritis is traditionally viewed as a noninflammatory arthropathy, an osteoarthritic joint could be considered a mildly inflamed organ.
Extracellular Matrix Remodeling Biomarkers
Because of the aforementioned issues, the focus of many biomarker studies is on markers that mirror disease-related changes in the joints, especially markers for remodeling (i.e., degradation and synthesis) of the extracellular matrix.57 Concomitant changes may occur in all joint tissues (cartilage, bone, and synovium), and for a comprehensive assessment of these changes molecular markers are necessary for each of these tissues.58,59 For the markers derived from these different tissues, there can be a substantial difference in which biologic fluid biomarker levels are determined.
The concentration of markers in body fluids reflects not only the dynamics of the disease but also the rate of clearance and the amount of remaining tissue. Cartilage-derived markers diffuse out of the tissue and enter the synovial fluid, which may vary in volume depending on the severity of ongoing inflammation. Synovial fluid urea levels may be used to correct for this “dilution.”60 Clearance from the synovial fluid predominantly occurs via lymphatic drainage, and partial degradation may occur in the lymph nodes, depending on the marker studied. Synovial clearance may depend on the severity of the ongoing synovitis and be determined by the permeability of the synovial membrane microvasculature.61 When the marker enters the systemic circulation, dilution occurs (e.g., 5 mL of synovial fluid vs. approximately 5 L of blood), and marker levels are confounded by molecules from other affected, or nonaffected, joints or even from nonarticular cartilage.
Collagen Markers
The main constituent of the extracellular matrix of connective tissues is collagen, which plays an essential role in the maintenance of tissue shape, strength, and integrity. Fibrillar collagen types I, II, and III are synthesized as propeptide-containing α chains that are post-translationally modified by hydroxylation of lysyl and prolyl residues and by glycosylation of hydroxylysyl residues. Triple helical collagen molecules are secreted from the cell, the propeptides are cleaved off, and fibrils that are stabilized by intermolecular cross-links are formed. This unique sequence of events provides a variety of tools that are used to study collagen synthesis (especially propeptides) and degradation (especially cross-links) in rheumatic diseases (Figure 34-2).62
In bone, type I collagen constitutes 90% of the organic matrix and markers of bone collagen turnover have proven valuable in monitoring diseases such as osteoporosis.63 For some bone markers such as NTx (bone degradation [see later]) and osteocalcin (bone formation), assays have been approved by the FDA to monitor efficacy of antiresorptive therapies or bone formation, sometimes even as a point-of-care test. For these bone metabolites, there is a significant menopausal effect that requires properly matched control groups and careful interpretation of the data.
Several assays have been used to assess collagen type I degradation in RA and osteoarthritis. The cross-linked C-terminal telopeptide (ICTP) is released by matrix metalloproteinase (MMP) cleavage of type I collagen, and its levels reflect MMP-mediated soft tissue degradation.64 The NTx/CTx-I assay and ICTP assay detect type I collagen degradation, but by different proteases. Cathepsin K–mediated osteoclastic bone resorption destroys ICTP antigenicity.64 Slightly elevated serum ICTP levels are found in RA compared with controls and are associated with disease activity measured by ESR, CRP levels, and swollen joint counts.65 In osteoarthritis, fourfold increased ICTP levels have been detected in patients with rapid progressive hip osteoarthritis compared with patients with slowly progressive disease.66
When the C-terminal or the N-terminal telopeptide of type I collagen (CTx or NTx) is released from bone degraded by cathepsin K, an epitope that is different from the MMP-mediated ICTP epitope is generated. In osteoarthritis patients, serum and urinary CTx levels are decreased compared with controls, which suggests decreased bone remodeling in osteoarthritis.59,67 In RA, urinary NTx and CTx levels are increased compared with healthy controls and are sensitive to change after treatment.68–70
Although on initial examination CTx and ICTP seem to provide identical information, closer inspection reveals that these two markers, although based on the same principle of detecting type I collagen telopeptides, may provide valuable complementary information. CTx and NTx levels are low in patients with pyknodysostosis, which is caused by a deficient activity of cathepsin K, whereas ICTP levels are elevated in this condition. It has also been shown that in postmenopausal women, anti–bone resorption therapy by hormone replacement reduced serum CTx levels, whereas ICTP levels did not change.71 In cartilage, type II collagen constitutes 80% of the dry weight of the tissue. Damage to the collagen network (collagen degradation and subsequent denaturation) is one of the first features of osteoarthritis and contributes significantly to the decreased mechanical properties of osteoarthritic cartilage.72 Using monoclonal antibodies, release of cross-linked type II collagen telopeptides (C-telopeptide, CTx-II) was shown to reflect cartilage degradation with high tissue specificity.73 Urinary CTx-II levels were significantly increased in RA and osteoarthritis patients compared with healthy controls, although the ranges overlapped considerably.73–75 In a population-based study, subjects with a CTx-II level in the highest quartile had a 4.2-fold increased risk of having radiographic osteoarthritis of the knee and hip (compared with subjects in the lowest quartile) and a 6-fold (knee) or 8.4-fold (hip) increased risk for progression of osteoarthritis.76 In osteoarthritis patients, CTx-II levels correlated with radiologic joint space narrowing and joint surface area but did not correlate with WOMAC indices of clinical status in these osteoarthritis patients.59 In patients with hip osteoarthritis, urinary CTx-II levels greater than 346 ng/mmol creatinine were associated with a twofold increase in radiographic disease progression compared with patients whose levels were less than 346 ng/mmol creatinine.77 Treatment of these patients with a candidate antiosteoarthritis drug (diacerein) seemed to modulate the CTx-II levels consistent with the effects on disease progression.77,78 In a study of patients with radiographic osteoarthritis in multiple joints, there was a significant association between the total radiographic OA score and urinary CTx-II levels. Subsequent multivariate analysis showed that the joint site–specific ROA score at all joint sites except for spinal disk degeneration contributed independently to this association.79
In RA patients, increased baseline CTx-II levels were associated with progression of joint damage, which was independent of baseline damage, treatment, and disease activity.75 Other studies also reported that urinary CTx-II is a strong, independent predictor of progressive joint destruction in RA.80,81
In patients with active RA, the decrease in urinary CTx-II levels that was observed 3 months after initiation of treatment predicted the radiographic disease progression over 5 years, suggesting that urinary CTx-II levels may be used as an early marker of treatment efficacy.82 Anti-TNF treatment of patients with active RA for more than 1 year resulted in a significant reduction of CTx-II levels in the progressive group but not in the nonprogressive group, although the levels were not significantly different from baseline.83 Additional data on the influence of intervention therapy on CTx-II levels are required to judge whether this biomarker is valuable for assessing efficacy of treatment to halt further joint destruction.
Apart from telopeptide fragments, neoepitopes resulting from the cleavage of type II collagen by collagenases (MMP-1, MMP-8, and MMP-13) have been used to monitor cartilage collagen damage.84–86 Urinary excretion of collagen fragments containing the C-terminal neoepitope (TIINE assay, using antibody 9A4) was increased in osteoarthritis patients compared with age-matched healthy controls.87 In synovial fluid, levels of a similar C-terminal neoepitope (C2C epitope, using the Col2-3/4Clong antibody) were significantly higher in osteoarthritis than in RA.88 In RA patients, serum levels of these biomarkers (C2C and C1,2C, a similar epitope present in types I and II collagen) were associated with progression of radiographic joint damage.89 In a randomized, double-blind, placebo-controlled glucosamine discontinuation trial of 137 subjects with knee osteoarthritis, neither C2C level nor C1,2C level, or their ratio, was affected by the treatment.90 Urinary levels of the 622-632 peptide of type II collagen (also known as HELIX-II) were increased in patients with primary knee osteoarthritis (281 ng/mmol creatinine) and RA patients (409 ng/mmol creatinine) compared with healthy controls (180 ng/mmol creatinine).86 However, this and other reports86,91 applying the HELIX-II assay have to be interpreted with care because due to an apparent database error in the sequence, the epitope used to generate the HELIX-II antibody does not occur in cartilage type II collagen.92 Consequently, based on collagen sequences and HELIX-II epitope properties, type III collagen is one of several candidate sources of the cross-reacting signal in body fluids, but not type II collagen.92 This example illustrates an intrinsic potential deficit of all enzyme-linked immunosorbent assays (ELISAs), whether in competitive or sandwich format. These assays measure all immune reactivity but do not characterize which peptide binds to the ELISA antibody. Results are therefore potentially confounded by the contribution of additional peptides containing that same epitope but derived from other proteins. Liquid chromatography–tandem mass spectrometry (LC–MS/MS) assays are ideal to quantify unique peptides from a mixture of peptides. Therefore immune affinity LC–MS/MS assays that capture relevant peptides using an antibody and quantify the peptide of interest using mass spectroscopy combine the best of both assays.93
Collagen Cross-Links
Degradation of fibrillar collagen types I, II, and III results in the quantitative excretion of the cross-links hydroxylysylpyridinoline (HP), derived from bone and soft tissue including cartilage, and lysylpyridinoline (LP), derived from bone, in the urine. In RA patients who received combination therapy (sulfasalazine, methotrexate, and prednisolone in the COBRA trial), time-integrated urinary HP levels correlated with the progression of radiographic joint damage (Sharpe/van der Heijde score) and bone mineral density.94 In osteoarthritis patients, urinary HP and LP levels were increased compared with age-matched controls.95 In the same patient population, followed for 1 year, only the cluster of baseline bone metabolism markers (comprising HP, LP, and serum bone sialoprotein)—out of the 14 molecular markers measured—significantly correlated with baseline clinical scores for pain, stiffness, and disease activity.96 None of the baseline cartilage or bone metabolism markers correlated with disease progression after 1 year of follow-up.96 In these patients, HP that is not derived from bone (extraosseous HP) showed a highly significant correlation with the acute-phase response, suggesting that in osteoarthritis, cartilage degradation is related to the degree of inflammation.95
The glycosylated analogue of HP, glucosyl-galactosyl-pyridinoline (GGHP), is present in human synovial tissue and is released during its degradation in vitro. GGHP is virtually absent in bone, whereas low levels have been detected in muscle and liver and intermediate levels have been detected in cartilage. Methodological problems such as the stability of HP during the alkaline hydrolysis that is necessary to liberate glycosylated molecules from tissues have so far prevented solid data on tissue distribution.97 Urinary GGHP levels were increased in early RA patients versus controls, and baseline levels correlated with disease progression, albeit weakly.75 Pending definite information on its tissue distribution, urinary GGHP might prove to be a marker for synovial tissue degradation.
Overall, collagen cross-links seem useful in measuring bone and cartilage catabolism and contribute to understanding of the pathophysiology of osteoarthritis and RA. In this respect, age-related changes in articular cartilage may significantly affect its susceptibility to proteinase-mediated degradation98,99; this underscores the use of proper age-matched control groups. Results obtained in studies with young animals cannot be extrapolated to the adult human situation.
Collagen Synthesis
In an attempt to repair tissue damage, collagen synthesis is increased in osteoarthritis cartilage, leading to increased tissue levels of the C-terminal propeptide of type II collagen (PIICP, chondrocalcin; also referred to as CPII).100 The rate of PIICP release is proportional to the rate of current collagen synthesis because the propeptide has a half-life of approximately 18 hours.100 Synovial fluid PIICP levels are also increased and correlate with osteoarthritis severity and body mass index.101 PIICP levels were lower in serum of osteoarthritis patients than in that of healthy controls.95 Similarly, the serum N-propeptide of collagen type IIA (PIIANP, an alternative splice variant that is expressed in embryonic and osteoarthritic cartilage) is lowered in osteoarthritis serum versus controls. Preliminary studies indicate that the balance between cartilage synthesis (PIIANP) and cartilage degradation (urinary CTx-II) could be used to discriminate between patients with rapid compared with slow progression.75 More recently, a competition ELISA was developed for the N-propeptide of the type II collagen isoform that is expressed by mature adult chondrocytes (PIINP).102 Urine levels of PIINP were twofold higher than plasma levels in persons without radiographic signs of osteoarthritis. In patients with radiographic knee and/or hip osteoarthritis, plasma PIINP levels were fivefold lower than in the reference group without osteoarthritis.102 A comparable fivefold decrease was observed in RA patients, using a different ELISA.103 Absolute values of nanograms PIINP per milliter plasma were comparable (≈18 ng/mL) for both assays and control groups.102,103
In inflamed synovial tissue, the synthesis of type III collagen is upregulated, resulting in the production of its N-terminal propeptide. In osteoarthritis patients and RA patients with knee involvement, serum PIIINP levels are increased compared with age-matched controls.74,104,105 In RA patients, prednisolone treatment that resulted in clinical improvement also reduced serum PIIINP levels by 25% and levels remained suppressed until treatment was withdrawn.106 Thus far, collagen type III–specific degradation markers have not been described, and because type III collagen has a broad distribution in soft tissues and blood vessels, its potential as a specific biomarker seems limited.
Proteoglycan Markers
The main noncollagenous constituent of articular cartilage is aggrecan, a large proteoglycan composed of a core protein to which glycosaminoglycan chains (e.g., keratan sulfate, chondroitin sulfate) are attached. Researchers have described various assays to measure aggrecan metabolism, but the available information is not always consistent. Depending on the antibodies used, serum keratan sulfate levels were reported to be either increased (antibody 5D4)107–109 or decreased (antibody AN9P1)110 in osteoarthritis patients compared with controls. Additionally, previous work has suggested that serum 5D4-reactive keratan sulfate levels are either similar109 or higher in osteoarthritis than in RA.88 In one study, the serum keratan sulfate levels (antibody AN9P1) were 30% lower in osteoarthritis patients than in age-matched controls.95,96 For RA patients, a negative correlation between serum keratan sulfate levels and inflammation has been found.111
The aggrecan epitope 846, which reflects the synthesis of proteoglycans in an attempt to repair, was increased in cartilage of osteoarthritis patients,112 and synovial fluid levels correlated with other markers such as cartilage oligomeric matrix protein (COMP), PIICP, tissue inhibitor of metalloproteinases 1 (TIMP-1), MMP-1, and MMP-3, as well as with the degree of radiologic damage.113 The epitope 846 levels in serum were lower, however, in osteoarthritis patients than in healthy controls96,114 and RA patients.88,96 In the RA patients, elevated levels of epitope 846 could predict a benign course of the disease.115 Taken together, none of the aggrecan-derived markers has shown sufficient power to discriminate between patients and controls or to provide consistent information that can be used in clinical studies. This effect may be partly caused by diurnal variation of these biomarkers, which may obscure relevant differences between study groups, especially when serum or urine sampling is not standardized.116 Similarly, increased motility of patients after initiation of successful treatment may affect circulating proteoglycan biomarker levels.
The identification of two members of the ADAM-TS family of proteases (ADAM-TS4 and ADAM-TS5) as aggrecanases117 supplied new tools to develop aggrecan-based markers for cartilage destruction. Antibodies directed at aggrecanase and MMP-generated neoepitopes in aggrecan core protein have been produced and applied in a variety of in vitro studies aimed at unraveling mechanisms of cartilage destruction in osteoarthritis. In synovial fluid from patients with a variety of joint diseases, MMP and aggrecanase-released aggrecan fragments have been detected.118–120 These studies have also shown that these two groups of proteases may have distinct roles in articular cartilage catabolism.121
Hyaluronan
The glucuronic acid chain hyaluronan (hyaluronic acid) is a constituent of cartilage and synovium and is synthesized by many cell types. It functions as the anchor for proteoglycans such as aggrecan, allowing the formation of the large aggregates that are responsible for the resilience of cartilage. In the synovial membrane, hyaluronan is synthesized by synovial fibroblasts and secreted into the synovial fluid to provide lubrication of the joint and to facilitate joint movement. Synovial fluid hyaluronan levels are decreased in osteoarthritis patients122 and may partly explain impaired joint function and pain. This provides the rationale for visco-supplementation therapy in osteoarthritis patients, consisting of intra-articular injections with hyaluronan derivatives.
Elevated blood hyaluronan levels have been reported for osteoarthritis patients and RA patients. In RA, some studies failed to show a relationship between plasma hyaluronan levels and measures of disease activity,123 whereas others showed significant correlations between serum hyaluronan levels and a variety of measures of inflammation and destruction (e.g., CRP, ESR, Ritchie index, radiologic damage).124 In osteoarthritis patients, elevated serum hyaluronan levels correlated weakly with the degree of cartilage degeneration.123 In addition, baseline hyaluronan levels could predict progression of osteoarthritis77,125 and serum levels were shown to increase with disease severity.126 These results suggest that an increase in circulating systemic hyaluronan levels could reflect synovial inflammation rather than cartilage destruction, prompting care in use of hyaluronan as a joint destruction marker. Also, the observation that diet and increased physical activity can influence its serum levels should be considered when using hyaluronan as a biomarker in joint diseases.116,127
Cartilage Oligomeric Matrix Protein
Since its discovery in the early 1990s, COMP has received much attention as a putative cartilage destruction marker. Although its exact function is unclear, COMP has been implicated in collagen fibrillogenesis. Increased COMP levels have been detected in the synovial fluid of osteoarthritis patients.128 In addition, COMP levels are increased in the serum of osteoarthritis patients compared with healthy controls74,129 and are associated with progression of radiographic signs of osteoarthritis.130 In osteoarthritis patients, the serum COMP levels were even higher than in RA patients.131 In early RA patients, increased serum COMP levels were identified as a strong predictor of early large-joint destruction.132–134 These data generated interest in the use of measurement of COMP levels as a selective cartilage destruction marker.
The expression of COMP in joint tissues other than cartilage including synovium, tendons, ligaments, and menisci raised concerns about its tissue specificity. High expression of COMP messenger RNA (mRNA) has been shown in murine osteoarthritis, indicating that synovial fluid COMP levels may reflect not only tissue degradation, but also the rate of new synthesis.135 The concerns about the use of COMP measurement as a specific cartilage degradation marker are fueled further by a study showing that the extent of synovial inflammation is one of the factors determining the serum COMP.136 In concordance, in early RA a positive correlation was found between serum COMP and the inflammatory marker CRP. Circulating COMP levels in this study were statistically higher in patients showing bone erosions on magnetic resonance imaging (MRI) than those without bone erosions.137 Other investigators did not observe a relationship between markers of inflammation and serum COMP levels (using a polyclonal antiserum recognizing all COMP forms) in RA patients.138 In this same study, COMP levels did not have any prognostic value with respect to progression of joint damage.138 Similar to the CTx and ICTP assays for collagen degradation, the use of antibodies or antiserum recognizing different epitopes within (fragments of) the COMP molecule might explain the apparent inconsistent results in the literature.139
Metalloproteinases
In addition to cartilage breakdown products, metalloproteinases (MMPs and aggrecanases) and their endogenous inhibitors (TIMPs) that are involved in the pathologic degradation of joint tissues could serve as useful markers. Data on MMP levels as a predictor for the progression of joint erosion in early RA are rapidly accumulating. Serum and synovial fluid MMP-3 (stromelysin) levels are increased in RA patients compared with controls.75,140 MMP-3 levels correlate with inflammation markers and disease activity in patients with untreated active RA141 and in early RA patients who received nonsteroidal anti-inflammatory drugs only.138,140 In these studies, serum MMP-3 levels were not related to radiographic progression.138 Another study revealed an association between serum proMMP-3 concentrations at disease onset and progression of joint destruction, which was independent of known risk factors such as the presence of the shared epitope and serum levels of rheumatoid factor and CRP.142 Serum MMP-3 also correlated significantly with radiographic progression at entry and longitudinally in a study with early RA patients.143 In addition, it was demonstrated in a prospective study that increased serum MMP-3 is a predictive marker for the progression in disablility.144 Several investigators showed that MMP-3 levels respond to therapeutic intervention with disease-modifying antirheumatic drugs (DMARDs), resulting in decreased MMP-3 level, which is associated with clinical improvement.27,30,143,145–147 Furthermore, baseline serum MMP-3 levels contribute to reaching normal physical function after 2 years of infliximab (anti-TNF) treatment.148
MMP-1 (collagenase) levels have also been shown to indicate joint erosion independent of inflammation. In early RA patients, there was a positive correlation between the area under the curve measurements of MMP-1 serum levels (but not the area under the curve of CRP levels) and the number of new joint erosions after 18 months of follow-up.140 Arthritic patients treated with anti-TNF antibodies (infliximab) for 14 weeks did not show reduced MMP-2 and MMP-9 levels (assessed by zymography, which does not reliably detect the other MMPs) despite clear clinical improvement.149 These data suggest that MMP levels, although they may be correlated with parameters of inflammation, do not reflect exactly the same pathways as do acute-phase reactants and could provide valuable additional information on joint destruction in RA.
Extrapolated to osteoarthritis, in which secondary inflammation is usually mild, these data suggest that MMP levels may provide valuable predictive markers. In a cross-sectional study in osteoarthritis patients, MMP-3 serum levels were similar, however, to levels in healthy controls.150 In a subset (120 patients) of 431 patients participating in a randomized, placebo-controlled trial evaluating the effects of doxycycline treatment on unilateral knee osteoarthritis, baseline plasma MMP-3 levels predicted joint space narrowing.151 In another study, the serum levels of TIMP-1 did not differ significantly between patients with unilateral or bilateral hip osteoarthritis and healthy controls.152 Within the osteoarthritis group, patients with rapid disease progression (joint space narrowing > 0.6 mm/yr; 1-year follow-up) had significantly lower serum TIMP-1 levels than patients with slow progression (joint space narrowing < 0.6 mm/yr).152 Apart from these, comprehensive studies for predictive MMP markers in osteoarthritis are not yet available and information about the use of MMP levels in osteoarthritis is still incomplete.
In recent years, more data on the role of aggrecanase in degradation of the major proteoglycan of cartilage (aggrecan) became available. Its pivotal role in tissue destruction is now widely accepted.117,153,154 Because of the difficulties in measuring aggrecanase activity in biologic samples, however, their value as a biomarker to monitor joint destruction is not yet fully understood. It is hoped that the more recent development of several aggrecanase assays,155,156 especially the immunoaffinity-based liquid chromatography–tandem mass spectrometry (LC-MS/MS) method, which detects cleavage at the 374ARGS site and the 1820AGEG site,157 will facilitate its use as a biomarker. The development of preclinical models and approaches to develop and evaluate selective aggrecanase inhibitors may contribute to the development of antiosteoarthritis drugs, which concomitantly are tools to validate the biomarkers.93,158,159
Biologic Markers in Synovial Tissue
Because many inflammatory arthropathies including RA primarily involve the synovial tissue, there has been increased interest in investigations of the pathologic changes in synovial biopsy specimens. This development has been stimulated further by technical advances such as the advent of new methods to obtain synovial tissue specimens from actively inflamed joints and clinically quiescent joints under local anesthesia and because of the development of immunohistologic methods, in situ hybridization, quantitative polymerase chain reaction, and microarray technology. Previous work has shown the relationship between the features of rheumatoid synovial tissue on the one hand and arthritis activity160 and joint destruction161 on the other (see also Chapter 53). The importance of evaluation of synovial tissue samples has been underscored by the observation that clinical signs of arthritis activity are associated with histologic signs of synovitis after treatment of RA patients with the monoclonal antibody alemtuzumab (Campath-1H), despite profound depletion of circulating lymphocytes.162 Similarly, rituximab treatment leads to a rapid and significant decrease in synovial B cell numbers in only a subset of RA patients, whereas circulating B cells are completely depleted in nearly all patients (Figure 34-3).163–165
Several methodological questions needed to be answered before serial synovial biopsy could be used to screen for potentially relevant effects after antirheumatic treatment.166 It has been shown in cross-sectional studies that biopsy samples can be acquired by blind needle technique and by miniarthroscopy.167 There are limitations and disadvantages, however, of the use of serial blind needle biopsy in the evaluation of treatment. It is usually restricted to larger joints such as the knee joint; the operator is not able to select the tissue visually, causing potential sampling error; and it is not always possible to obtain adequate tissue samples. This is especially true when clinically quiescent joints are investigated (e.g., after successful therapy). Comparison of the features of synovial inflammation in biopsy samples from inflamed knee joints and paired inflamed small joints of RA patients revealed that inflammation in one inflamed joint is generally representative of the inflammation in other inflamed joints.168 It is possible to use serial samples from the same joint, selecting either large or small joints, for the evaluation of antirheumatic therapy. Sampling error can be reduced by selecting at least six biopsy specimens from multiple regions, resulting in variance of less than 10%.22,169 When the biopsy samples are taken from an actively inflamed joint, there is on average no clear-cut difference in the features of synovial inflammation or the expression of mediators of inflammation and destruction at the pannus-cartilage junction compared with other regions away from the pannus-cartilage junction.170–172 Ultrasound-guided biopsy is a newer technique, which can be performed in both small and large joints, bursae, and tendon sheaths under local anaesthesia.173 Although this method is appealing, further validation is necessary. A recommendation for standardization of various synovial biopsy techniques to be used in clinical trials has recently been published.174
An extensive quality control system is required to allow reliable analysis by immunohistochemistry, tissue enzyme-linked immunosorbent assay, quantitative polymerase chain reaction, or microarray analysis.175 Finally, sophisticated computer-assisted image analysis systems allow reliable and efficient evaluation of the synovial cell infiltrate and the expression of adhesion molecules, cytokines, and MMPs in innovative clinical trials.176
Using this approach, successful treatment with disease-modifying antirheumatic drugs such as gold,177 methotrexate,22,178,179 and leflunomide179 was shown to be associated with decreased mononuclear cell infiltration. Similarly, successful treatment of RA patients with infliximab,23,180,181 rituximab,164,182,183 and abatacept184 results in reduced synovial inflammation. The number of macrophages in the synovium was decreased already 24 to 48 hours after initiation of infliximab treatment.24,185 Similarly, high-dose intravenous methylprednisolone reduced expression of TNF in synovial biopsy samples 24 hours after treatment, a result that correlated with a clinical response to, and subsequent relapse after, methylprednisolone therapy.186
A randomized trial has formally addressed the question of which feature in RA synovial tissue samples could be used as a biomarker for clinical efficacy in relatively small studies of short duration.187 Patients received either prednisolone according to the COBRA regimen or placebo for 2 weeks. This study identified sublining macrophages as an important immunohistologic biomarker associated with the clinical response to corticosteroids. The utility of macrophages in the synovial sublining as a candidate biomarker across discrete interventions and kinetics has also been observed,176 with a correlation between the mean change in disease activity score (Δ DAS28) and the mean change in the number of sublining macrophages. When patients from several actively treated studies were grouped, the standardized response mean, a measure of sensitivity to change, was 1.16 for the change in DAS28 and 0.83 for the change in sublining macrophages. For the patients from the placebo groups, the standardized response mean was −0.23 (for DAS28) and 0.30 (for macrophages), consistent with the notion that the biomarker is less susceptible to placebo effects or expectation bias than clinical evaluation, which includes subjective measures of disease activity.176,188 In addition to its role as a possible marker of clinical response the change in numbers of sublining macrophages could potentially help to distinguish effective from ineffective treatment. Taken together, these studies suggest that analyses of serial biopsy samples can be used as a screening method to test new drug candidates requiring relatively small numbers of subjects. The absence of changes after treatment would suggest that the therapy is probably not effective. The demonstration of biologic changes at the site of inflammation could provide the rationale for larger, placebo-controlled trials, however. Most of the biopsy studies have been performed in RA patients, but it appears likely that the same approach can be used for the evaluation of novel therapies in patients with other rheumatologic disease such as spondyloarthritis.189–192
As an alternative to immunohistologic and in situ hybridization methods, quantitative polymerase chain reaction on small synovial biopsy specimens can be employed to evaluate drug effects in clinical trials.166,169 Using this approach, prednisolone was shown to reduce expression of IL-8 and MMP-1 in synovial biopsy specimens of RA patients after 2 weeks of treatment.193 Because the biopsy specimens contain various cell types, it is important to realize that a change in gene expression level may also reflect a change in cellular composition of the biopsy, not a change in the expression level within a certain cell type.
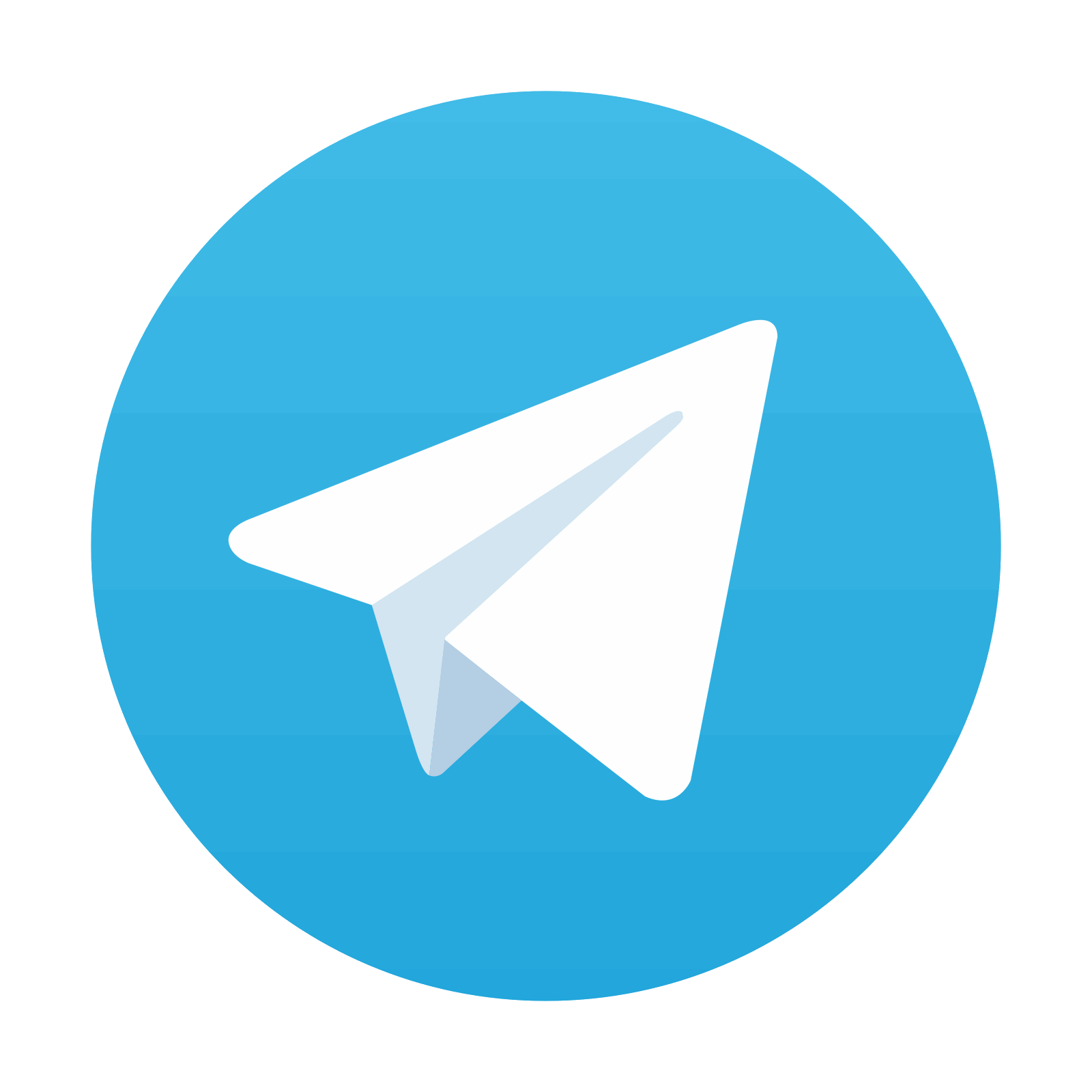
Stay updated, free articles. Join our Telegram channel

Full access? Get Clinical Tree
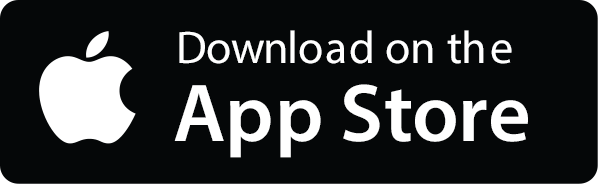
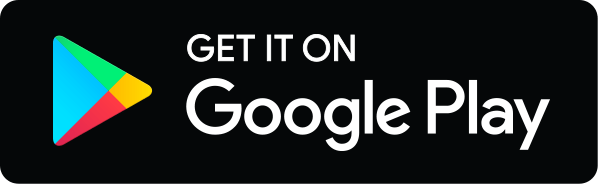