Framework and support: the connective tissues
The overall function of connective tissue is to unite or connect structures in the body, and to give support. Bone is a connective tissue which provides the rigid framework for support. Where bones articulate with each other dense fibrous connective tissue, rich in collagen fibres, surrounds the ends of the bones, allowing movement to occur while maintaining stability. Cartilage, another connective tissue, is also found associated with joints, where it forms a compressible link between two bones, or provides a low-friction surface for smooth movement of one bone on another. Connective tissue attaches muscles to bone, in the form of either a cord (tendon) or a flat sheet (fascia). The connective tissues may be divided into:
- dense fibrous tissue;
- cartilage;
- bone.
Dense fibrous tissue
Dense fibrous connective tissue unites structures in the body while still allowing movement to occur. It has high tensile strength to resist stretching forces. This connective tissue has few cells and is largely made up of fibres of collagen and elastin that give the tissue great strength. The fibres are produced by fibroblast cells that lie in between the fibres (Figure 1.1). The toughness of this tissue can be felt when cutting through stewing steak with a blunt knife. The muscle fibres are easily sliced, but the covering of white connective tissue is very tough. Examples of this tissue are as follows:
Figure 1.1 Dense fibrous connective tissue seen covering bone as periosteum, and forming the tendon of a skeletal muscle.
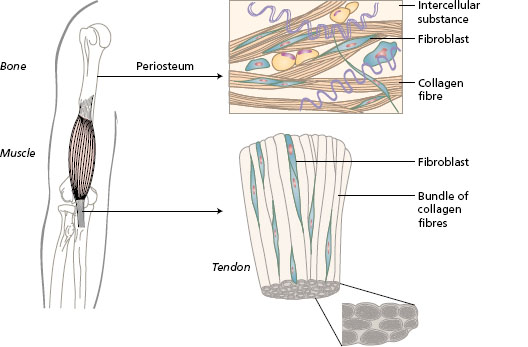
- The capsule surrounding the movable (synovial) joints which binds the bones together (see Figure 1.7).
- Ligaments form strong bands that join bone to bone. Ligaments strengthen the joint capsules in particular directions and limit movement.
- Tendons unite the contractile fibres of muscle to bone.
In tendons and ligaments, the collagenous fibres lie in parallel in the direction of greatest stress.
- An aponeurosis is a strong flat membrane, with collagen fibres that lie in different directions to form sheets of connective tissue. An aponeurosis can form the attachment of a muscle, such as the oblique abdominal muscles, which meet in the midline of the abdomen (see Chapter 10, Figure 10.6). In the palm of the hand and the sole of the foot an aponeurosis lies deep to the skin and forms a protective layer for the tendons underneath (see Chapter 8, Figure 8.21).
- A retinaculum is a band of dense fibrous tissue that binds tendons of muscles and prevents bowstring during movement. An example is the flexor retinaculum of the wrist, which holds the tendons of muscles passing into the hand in position (see Chapter 6, Figure 6.15).
- Fascia is a term used for the large areas of dense fibrous tissue that surround the musculature of all the body segments. Fascia is particularly developed in the limbs, where it dips down between the large groups of muscles and attaches to the bone. In some areas, fascia provides a base for the attachment of muscles, for example the thoracolumbar fascia gives attachment to the long muscles of the back (see Chapter 10, Figure 10.6).
- Periosteum is the protective covering of bones. Tendons and ligaments blend with the periosteum around bone (see Figure 1.3).
- Dura is thick fibrous connective tissue protecting the brain and spinal cord (see Chapter 3, Figure 3.21).
Cartilage
Cartilage is a tissue that can be compressed and has resilience. The cells (chondrocytes) are oval and lie in a ground substance that is not rigid like bone. There is no blood supply to cartilage, so there is a limit to its thickness. The tissue has great resistance to wear, but cannot be repaired when damaged.
Hyaline cartilage is commonly called gristle. It is smooth and glass-like, forming a low-friction covering to the articular surfaces of joints. In the elderly, the articular cartilage tends to become eroded or calcifies, so that joints become stiff. Hyaline cartilage forms the costal cartilages which join the anterior ends of the ribs to the sternum (Figure 1.2). In the developing foetus, most of the bones are formed in hyaline cartilage. When the cartilaginous model of each bone reaches a critical size for the survival of the cartilage cells, ossification begins.
Figure 1.2 Microscopic structure of hyaline and fibrocartilage, location in the skeleton of the trunk.
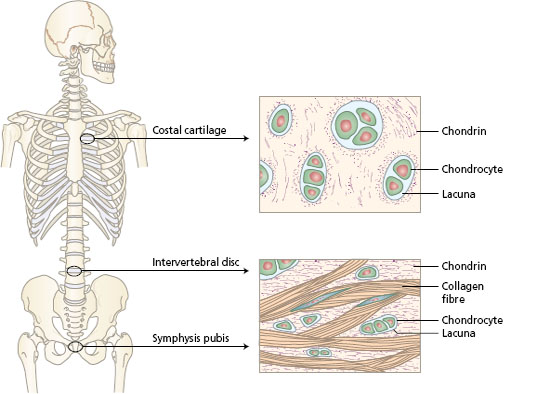
Fibrocartilage consists of cartilage cells lying in between densely packed collagen fibres (Figure 1.2). The fibres give extra strength to the tissue while retaining its resilience. Examples of where fibrocartilage is found are the discs between the bones of the vertebral column, the pubic symphysis joining the two halves of the pelvis anteriorly, and the menisci in the knee joint.
Bone
Bone is the tissue that forms the rigid supports for the body by containing a large proportion of calcium salts (calcium phosphate and carbonate). It must be remembered that bone is a living tissue composed of cells and an abundant blood supply. It has a greater capacity for repair after damage than any other tissue in the body, except for blood. The strength of bone lies in the thin plates (lamellae), composed of collagen fibres with calcium salts deposited in between. The lamellae lie in parallel, held together by fibres, and the bone cells or osteocytes are found in between. Each bone cell lies in a small space or lacuna, and connects with other cells and to blood capillaries by fine channels called canaliculi (Figure 1.3).
In compact bone, the lamellae are laid down in concentric rings around a central canal containing blood vessels. Each system of concentric lamellae (known as a Haversian system or an osteon) lies in a longitudinal direction. Many of these systems are closely packed to form the dense compact bone found in the shaft of long bones (Figure 1.3).
Figure 1.3 A section of the shaft of a long bone.
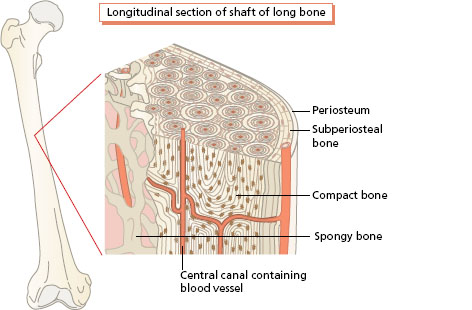
In cancellous or trabeculate bone, the lamellae form plates arranged in different directions to form a mesh. The plates are known as trabeculae and the spaces in between contain blood capillaries. The bone cells lying in the trabeculae communicate with each other and with the spaces by canaliculi. The expanded ends of long bones are filled with cancellous bone covered with a thin layer of compact bone. The central cavity of the shaft of long bones contains bone marrow. This organisation of the two types of bone produces a structure with great rigidity without excessive weight (Figure 1.4). Bone has the capacity to remodel in shape in response to the stresses on it, so that the structure lines of the trabeculae at the ends of the bone follow the lines of force on the bone. For example, the lines of trabeculae at the ends of weight-bearing bones, such as the femur, provide maximum strength to support the body weight against gravity. Remodelling of bone is achieved by the activity of bone-forming cells known as osteoblasts, and bone-destroying cells known as osteoclasts; both types of cell are found in bone tissue. The calcium salts of bone are constantly interchanging with calcium ions in the blood, under the influence of hormones (parathormone and thyrocalcitonin). Bone is a living, constantly changing connective tissue that provides a rigid framework on which muscles can exert forces to produce movement.
Figure 1.4 Gross structure of long bone: longitudinal and transverse sections.
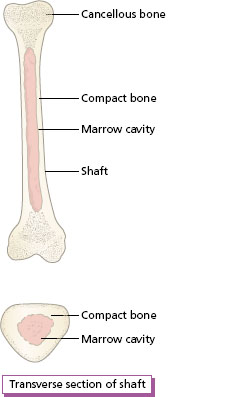
Articulations
Where the rigid bones of the skeleton meet, connective tissues are organised to bind the bones together and to form joints. It is the joints that allow movement of the segments of the body relative to each other. The joints or articulations between bones can be divided into three types based on the particular connective tissues involved. The three main classes of joint are fibrous, cartilaginous and synovial.
Fibrous joints
Here, the bones are united by dense fibrous connective tissue.
The sutures of the skull are fibrous joints that allow no movement between the bones. The edge of each bone is irregular and interlocks with the adjacent bone, a layer of fibrous tissue linking them (Figure 1.5a).
A syndesmosis is a joint where the bones are joined by a ligament that allows some movement between the bones. A syndesmosis is found between the radius and the ulna (Figure 1.5b). The interosseous membrane allows movement of the forearm.
A gomphosis is a specialised fibrous joint that fixes the teeth in the sockets of the jaw (Figure 1.5c).
Figure 1.5 Fibrous joints: (a) suture between bones of the skull; (b) syndesmosis between the radius and ulna; (c) gomphosis: tooth in socket.
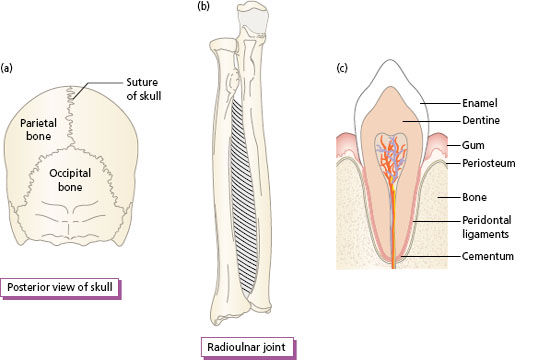
Cartilaginous joints
In these joints the bones are united by cartilage.
A synchondrosis or primary cartilaginous joint is a joint where the union is composed of hyaline cartilage. This type of joint is also called primary cartilaginous. The articulation of the first rib with the sternum is by a synchondrosis. During growth of the long bones of the skeleton, there is a synchondrosis between the ends and the shaft of the bone, where temporary cartilage forms the epiphyseal plate. These plates disappear when growth stops and the bone becomes ossified (Figure 1.6a).
A symphysis or secondary cartilaginous joint is a joint where the joint surfaces are covered by a thin layer of hyaline cartilage and united by a disc of fibrocartilage. This type of joint (sometimes called secondary cartilaginous) allows a limited amount of movement between the bones by compression of the cartilage. The bodies of the vertebrae articulate by a disc of fibrocartilage (Figure 1.6b). Movement between two vertebrae is small, but when all of the intervertebral discs are compressed in a particular direction, considerable movement of the vertebral column occurs. Little movement occurs at the pubic symphysis, the joint where the right and left halves of the pelvis meet. Movement is probably increased at the pubic symphysis in the late stage of pregnancy and during childbirth, to increase the size of the birth canal.
Figure 1.6 Cartilaginous joints: (a) synchondrosis in a child’s metacarpal bone, as seen on X-ray; (b) symphysis between the bodies of two vertebrae.
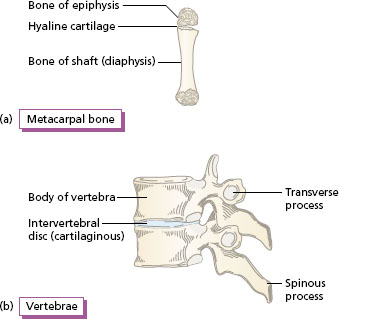
Figure 1.7 Typical synovial joint.
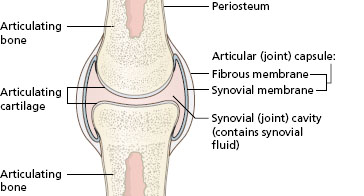
Synovial joints
Synovial joints are the mobile joints of the body. There is a large number of these joints, which show a variety of form and range of movement. The common features of all of them are shown in the section of a typical synovial joint (Figure 1.7) and listed as follows:
- Hyaline cartilage covers the ends of the two articulating bones, providing a low-friction surface for movement between them.
- A capsule of dense fibrous tissue is attached to the articular margins, or some distance away, on each bone. The capsule surrounds the joint like a sleeve.
- There is a joint cavity inside the capsule which allows free movement between the bones.
- Ligaments, bands or cords of dense fibrous tissue, join the bones. The ligaments may blend with the capsule or they are attached to the bones close to the joint.
- A synovial membrane lines the joint capsule and all non-articular surfaces inside the joint, i.e. any structure within the joint not covered by hyaline cartilage.
One or more bursae are found associated with some of the synovial joints at a point of friction where a muscle, a tendon or the skin rubs against any bony structures. A bursa is a closed sac of fibrous tissue lined by a synovial membrane and containing synovial fluid. The cavity of the bursa sometimes communicates with the joint cavity. Pads of fat, liquid at body temperature, are also present in some joints. Both structures have a protective function.
All of the large movable joints of the body, for example the shoulder, elbow, wrist, hip, knee and ankle, are synovial joints. The direction and the range of their movements depend on the shape of the articular surfaces and the presence of ligaments and muscles close to the joint. The different types of synovial joint are described in Chapter 2 where the directions of movement at joints are considered.
Skeletal muscle
Skeletal muscle is attached to the bones of the skeleton and produces movement at joints. The basic unit of skeletal muscles is the muscle fibre. Muscle fibres are bound together in bundles to form a whole muscle, which is attached to bones by fibrous connective tissue. When tension develops in the muscle, the ends are drawn towards the centre of the muscle. In this case, the muscle is contracting in length and a body part moves. Alternatively, a body part may be moved by gravity and/or by an added weight, for example an object held in the hand. Now the tension developed in the muscle may be used to resist movement and hold the object in one position.
In summary, the tension developed allows a muscle:
- to shorten to produce movement;
- to resist movement in response to the force of gravity or an added load.
Further more, muscles may develop tension when they are increasing in length. This will be considered in Chapter 2, in the section on types of muscle work.
Both muscle and fibrous connective tissue have elasticity. They can be stretched and return to the original length. The unique function of muscle is the capacity to shorten actively.
- Hold a glass of water in the hand. Feel the activity in the muscles above the elbow by palpating them with the other hand. The tension in the muscles is resisting the weight of the forearm and the water.
- Li ft the glass to the mouth. Feel the muscle activity in the same muscles as they shorten to lift the glass.
Structure and form
The structure of a whole muscle is the combination of muscle and connective tissues, which both contribute to the function of the active muscle. In a whole muscle, groups of contractile muscle fibres are bound together by fibrous connective tissue. Each bundle is called a fasciculus. Further coverings of connective tissue bind the fasciculi together and an outer layer surrounds the whole muscle (Figure 1.8).
Figure 1.8 Skeletal muscle: the organisation of muscle fibres into a whole muscle, and a sarcomere in the relaxed and the shortened state (as seen by an electron microscope).
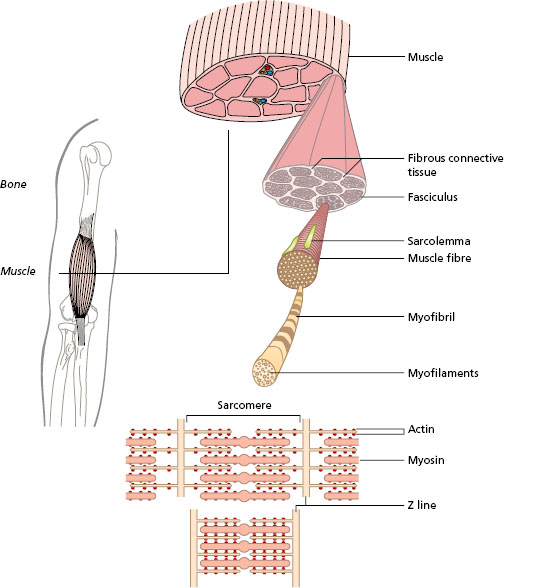
Figure 1.9 Elastic components of muscle.
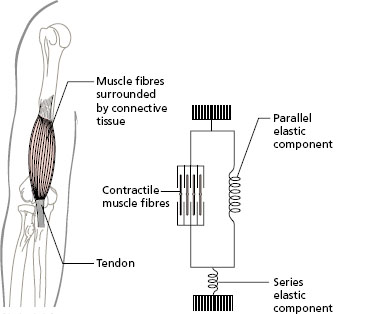
The total connective tissue element lying in between the contractile muscle fibres is known as the parallel elastic component. The tension that is built up in muscle when it is activated depends on the tension in the muscle fibres and in the parallel elastic component. The fibrous connective tissue, for example a tendon, which links a whole muscle to bone is known as the series elastic component. The initial tension that builds up in an active muscle tightens the series elastic component and then the muscle can shorten. A model of the elastic and contractile parts of a muscle is shown in Figure 1.9. If the connective tissue components lose their elasticity, through lack of use in injury or disease, a muscle may go into contracture. Lively splints are used to maintain elasticity and prevent contracture while the muscle recovers.
The individual muscle fibres lie within a muscle in one of the following two ways:
- Parallel fibres are seen in strap and fusiform muscles (Figure 1.10a, b). These muscles have long fibres which are capable of shortening over the entire length of the muscle, but the result is a less powerful muscle.
- Oblique fibres are seen in pennate muscles. The muscle fibres in these muscles cannot shorten to the same extent as parallel fibres. The advantage of this arrangement, however, is that more muscle fibres can be packed into the whole muscle, so that greater power can be achieved.
The muscles with oblique fibres are known as unipennate, bipennate or multipennate, depending on the particular way in which the muscle fibres are arranged (Figure 1.10c, d). Some of the large muscles of the body combine parallel and oblique arrangements. The deltoid muscle of the shoulder (see Chapter 5, Figure 5.9) has one group of fibres that are multipennate and two groups that are fusiform, which combines strength to lift the weight of the arm with a wide range of movement. The form of a particular muscle reflects the space available and the demands of range and strength of movement.
Figure 1.10 Form of whole muscle: parallel fibres (a) strap and (b) fusiform; oblique fibres (c) multipennate and (d) unipennate and bipennate.
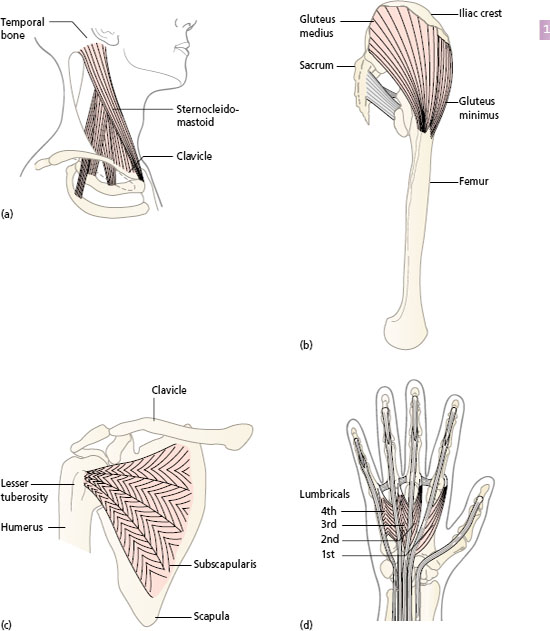
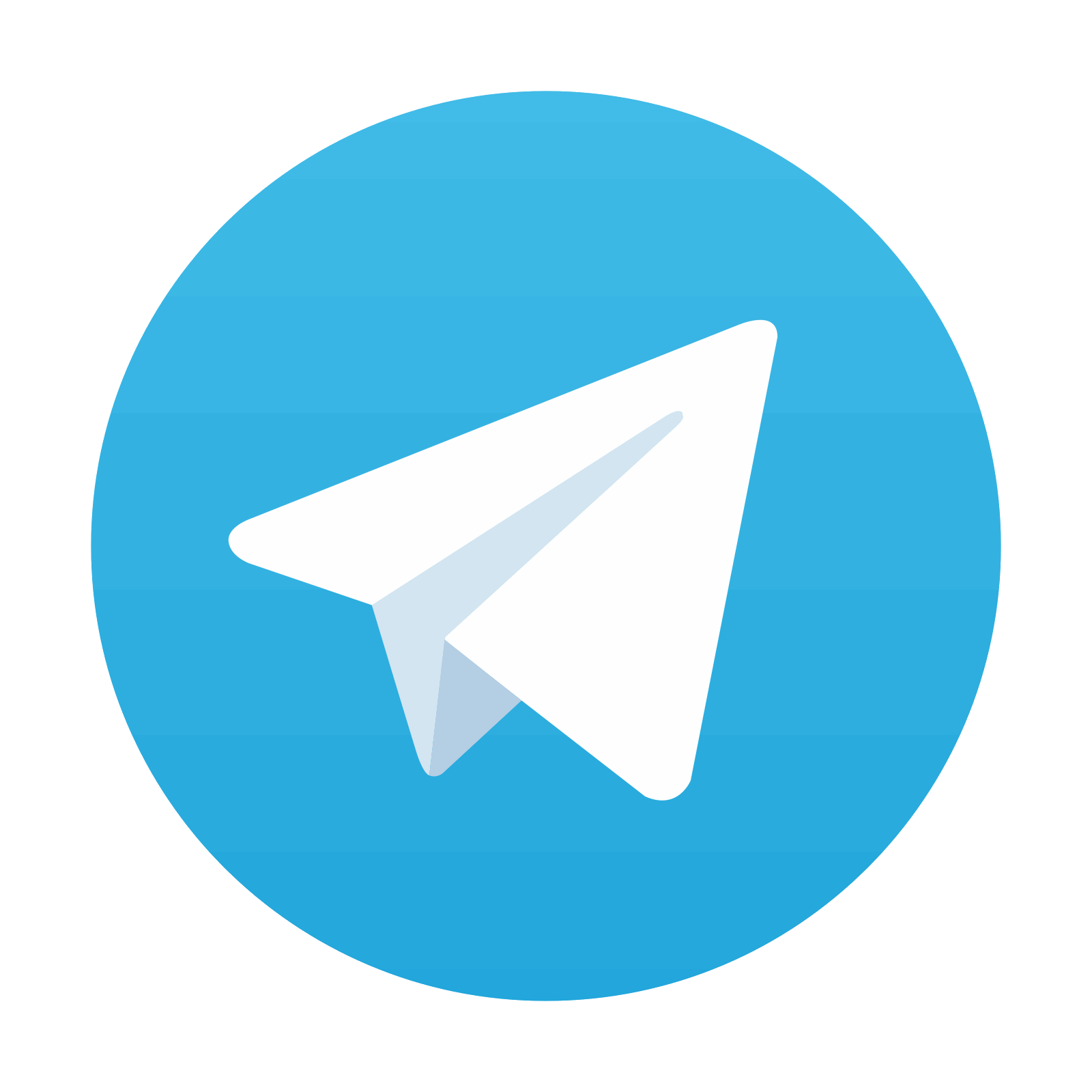