Radiologic Physics, Radiobiology, and Radiation Safety
RADIOLOGIC PHYSICS
What is radiation? What are x-rays? How are x-rays produced? How do x-rays interact with matter?
Basic Concepts
Radiation is defined as energy that is emitted and transferred through space. Examples include radio waves, visible light, and x-rays. Matter is exposed or irradiated when it intercepts and absorbs radiation. Some forms of radiation can remove an orbital electron from an atom with which it interacts; this process is known as ionization, and the radiation is called ionizing radiation.
There are two units of radiation measurement: the US customary system and the International System (SI); the latter has been officially adopted by all other countries. Although the United States does not use SI units for the public, all scientific inquiries use the SI system (Box 1-1). The unit for radiation exposure measured in air is the Roentgen or air kerma. The unit for the radiation absorbed dose is the rad or gray (Gy). The unit of effective dose and radiation received by radiation workers is referred to as rem (“roentgen-equivalent-man”) or sievert (Sv). A quantity of radioactive material is referred to as curie (Ci) or becquerel (Bq).
BOX 1-1 Radiation Units
Traditional Unit | SI Unit | Quantity of |
Roentgen (R) | Air kerma (Gya) | Exposure |
Rad | Gray (Gyt) | Absorbed dose |
Rem | Sievert (Sv) | Effective dose equivalent (E) |
Curie (Ci) | Becquerel (Bq) | Radioactivity |
Radiation can be classified in three ways: as particulate (alpha and beta particles) or nonparticulate (electromagnetic radiation—light, x-rays, radio waves); charged (alpha particles) or uncharged (x-rays); and ionizing (x-rays, gamma rays) or nonionizing (visible light, radio waves). Based on these three classifications, x-rays are nonparticulate, uncharged, and ionizing.
Radioactivity is defined as the emission of energy and particles by unstable atoms, radionuclides, to become stable. A radionuclide is an atom with a nucleus that undergoes radioactive decay. Radioisotopes have the same atomic number but a different atomic mass number (neutrons); most are artificially produced. Radioactive decay results in the emission of beta particles (a neutron converts into a proton and an electron-like particle is ejected), alpha particles (which consists of two protons and two neutrons bound together), and gamma rays (which are usually emitted simultaneously with a particle). Gamma rays, however, are a form of electromagnetic (nonparticulate) radiation that is useful in nuclear medicine.
Radioactive half-life is the time required for a quantity of radiation to be reduced to one-half of its original value. Seven half-lives are required before a quantity of radioactive material has decayed to less than 1%.
When discussing electromagnetic (nonparticulate) radiation, it is important to understand the relationship between the radiation’s wavelength and its energy (Box 1-2). Wavelength and energy are inversely proportional: the longer the wavelength, the lower its energy, and the shorter the wavelength, the greater its energy. Radio waves have the longest wavelength on the electromagnetic spectrum and, therefore, have the lowest energy. Magnetic resonance imaging uses radio waves. Visible light is important in diagnostic radiology because light is necessary in order to view the image on a view box or on a computer display. X-rays, used in both computed tomography and conventional radiography, have very short wavelengths and high energy. Lastly, gamma rays are used in nuclear medicine and have the highest energy.
BOX 1-2 The Electromagnetic (EM) Spectrum Applicable to Diagnostic Imaging
Ionizing radiation is either particulate or electromagnetic. Box 1-3 lists the types of ionizing radiation, where they originate, and whether or not they are charged. Sources of ionizing radiation are either natural or man-made. Natural sources represent 82% of human exposure and include cosmic rays, terrestrial (radon is the largest component), and radionuclides (such as potassium-40). The primary source of man-made ionizing radiation (18% of human exposure) is medical x-rays. Other man-made sources include nuclear medicine and consumer products.
BOX 1-3 Types of Ionizing Radiation
PARTICULATE (ORIGINATES FROM NUCLEUS, CHARGED)
• Alpha particles
– 4 atomic mass number, positive charge
– Emitted from nuclei of heavy elements
– High energy, short range
• Beta particles
– No mass, positive or negative charge
– Lower energy and longer range
ELECTROMAGNETIC (NO CHARGE, NO MASS)
• x-rays (originate in electron cloud, outside nucleus)
• gamma rays (originate in nucleus of radioisotope)
The photon is the smallest quantity of any type of electromagnetic radiation. It is also referred to as a quantum or bundle of energy. It travels at the speed of light, has no mass, and has no charge. Its frequency and wavelength are inversely related. In particular, it has a wave–particle duality, meaning it may behave as either a wave or a particle.
X-rays are similar to light waves by two of three phenomena: x-rays can transmit through matter and can be absorbed by matter, but matter does not reflect them. The most important interaction that the x-ray has with matter is its ability to be partially absorbed, known as attenuation. If x-rays totally penetrated matter, the resultant image would be black. If x-rays were totally absorbed by matter, the resultant image would be white. Partial absorption or attenuation of x-rays by matter is what gives the different shadows or shades of gray that are the actual image itself. Matter that absorbs x-rays will appear white in the image and is referred to as radiopaque; matter that attenuates x-rays will be radiolucent and appear darker in the radiograph.
Light intensity emitted from a source decreases rapidly as the distance from the source increases. The inverse square law defines this principle, where intensity is inversely proportional to the square of the distance between the object and the source (Box 1-4). Three of the four variables must be known in order to apply the inverse square law.
Unlike light, which is identified by wavelength, x-rays are identified by energy in electron volts (eV). Examples of x-ray energies used in medicine include 10 to 20 kVp (dermatology), 30 to 150 kVp (diagnostic imaging), and 200 to 1000 kVp (deep tissue therapy). X-rays travel in straight lines and can travel through a vacuum. Remember that photon energy is directly proportional to photon frequency and is inversely proportional to photon wavelength. Nonionizing radiation has low energy and long wavelength. Ionizing radiation has high energy and short wavelength.
BOX 1-4 Inverse Square Law
Example: If x-ray intensity is 1 rad at 2 ft, what would the intensity be at 4 ft?
Therefore, I2 = 0.25 rad
Photons interact with matter most easily when matter is approximately the same size as the photon’s wavelength. For example, the television antenna measures in meters, which is the approximate wavelength of radiofrequencies. The wavelength of visible light is measured in micrometers, which is the size of the rods and cones in the eye. The wavelength of x-rays is similar to the size of atoms and electrons.
Wilhelm Roentgen discovered x-rays in 1895 while investigating cathode rays (electrons) in a Crookes tube. A plate coated with barium platinocyanide, a fluorescent material, glowed while he experimented in a dark room, which he named “X-light.” He then interposed materials, including his hand, between the Crookes tube and the glowing plate. Roentgen was able to describe the properties of this unknown light in 1 month’s time and published the first medical radiograph in 1896. He received the first Nobel Prize for Physics in 1901.
The X-ray Machine
Diagnostic lower extremity–specific x-ray units are available either from dealers or directly from the manufacturer. Two types of lower extremity x-ray units are currently available: stationary and mobile.
The stationary lower extremity x-ray unit (Figure 1-1A) is more common and connects to a platform known as an orthoposer. The tube head is attached to the orthoposer by an arm or a track.
The mobile x-ray unit (Figure 1-1B) has a stand with wheels. The tube head is attached to a sliding track with counterbalance that is mounted to a movable stand instead of an orthoposer. The mobile unit can be moved easily from room to room; however, the wheels must be locked to prevent movement during the exposure.
The components of the x-ray machine include the control console, high-voltage generator, and the x-ray tube head.
Control Console
The operating or control console of a typical lower extremity x-ray unit either can be a separate box that is mounted inside or outside the x-ray room (Figure 1-1A) or may be incorporated as part of the tube head itself (Figure 1-1B). Three factors are under the control of the limited x-ray machine operator (LXMO): kVp (kilovoltage peak), mA (milliamperage), and exposure time (s). There also is an on/off control. kVp is a unit of electric potential and relates to the energy (quality) of the x-ray beam. A kVp between 55 and 65 is typically used for most foot and ankle applications. One can select between 50 and 70 kVp on most lower extremity x-ray units; higher kVp units are also available. mA represents the tube current and controls the number of photons that are produced. The mA of lower extremity x-ray units is quite low, between 10 and 30 mA. Some units may not have optional tube currents and are preset at the factory. The total quantity or output intensity of x-rays is known as the mAs, that is, milliamperage × time of exposure. A timer controls the length of exposure. Timers may be either synchronous or electronic in nature. Synchronous timers can be set as low as 1/60th of a second; electronic timers are even more accurate, to 1/100th of a second. Whereas mA and/or time manipulate the quantity of x-rays produced, kVp controls the energy or quality of the x-ray beam.
FIGURE 1-1. A: Stationary x-ray unit. The tube head is attached to the orthoposer at a fixed SID. (Courtesy of X-Cel X-Ray Corp., Crystal Lake, IL.) B: Mobile x-ray unit. (Courtesy of MinXray Inc., Northbrook, IL.)
An exposure switch is attached to the control panel by a long, coiled cord. It allows the operator to stand a minimum of 6 ft away from the x-ray source. The exposure button operates as a “dead man” switch: the exposure only occurs as the button is depressed and will shut off at the selected time or if the button is released, whichever occurs first.
High-Voltage Generator
Lower extremity–specific x-ray units plug into a standard 110-V electrical outlet and are shockproof. A transformer is used to convert this relatively low electrical voltage to the high voltage (kilovolts) required by the x-ray tube to produce x-rays. In addition, the current from the wall is alternating and flows in two directions; a rectifier converts the alternating current (AC) into direct current (DC), which is required by the x-ray tube (electrons in the tube can only travel in one direction, from cathode to anode). The electric current frequency (in the United States) is 60 Hz.
The high-voltage generator may be single phase, three phase, or high frequency. With single-phase power generation, the voltage waveform pulsates between zero and its maximum value from 60 to 120 times per second, depending on whether the rectification is half wave or full wave, respectively. (No x-rays are produced at zero.) This is also referred to as 100% voltage ripple (i.e., the variation between the maximum and minimum voltage is 100%). In contrast, the voltage across the x-ray tube is nearly constant and never drops to zero with a three-phase power generation. The resultant voltage ripple is less than 15%. A high-frequency generator is found in newer lower extremity–specific x-ray units; its voltage waveform is nearly constant and has less than 1% ripple, maintaining constant high voltage. They are smaller, less expensive, and more efficient than the single- and three-phase power options.
The X-ray Tube Head
Components of the x-ray tube head include the supporting arm, x-ray tube, and beam limitation device.
The x-ray tube head is held firmly in place by a supporting arm. Any movement of the tube head during the exposure can impair the quality of the final image. Depending on the type of x-ray unit one owns or operates, the arm may originate from an orthoposer or from a mobile stand (Figure 1-1). Arms that originate from an orthoposer are convenient to use because the distance between the x-ray tube and the image receptor, the source-to-image distance (SID), is fixed or limited. (The fixed SID aids in preventing technical errors that can result in poor-quality images.) In contrast, this distance must be determined manually when using a mobile unit; these units may be equipped with a measuring tape or a telescoping antenna-like device for determining the SID. Positioning of the tube head with the mobile unit is not as simple a task as positioning the stationary units with fixed SID. Inconstant SID can have a profound effect on the optical density of the final image if other technical factors are not calculated and adjusted accordingly. According to the NCRP (National Council on Radiation Protection and Measurements), the minimum distance allowable between the patient and the x-ray tube is 12 inches.
The x-ray tube is contained within a protective housing. The protective housing is filled with oil that surrounds the x-ray tube and provides electrical insulation and absorbs heat. The x-ray tube itself is enclosed in glass or metal and maintains a vacuum. There is a small window in the glass or metal tube enclosure that is thinner, which is where the x-rays escape and are directed toward the patient’s area of concern. Accordingly, the housing also has a small window where the x-rays for the study are emitted after exiting the tube enclosure; these x-rays form the useful beam.
The cathode is the negative electrode of the x-ray tube; it consists of a filament and a focusing cup (Figure 1-2A). The filament heats up and glows similar to a light bulb filament. However, instead of producing light, the cathode filament produces electrons. The filament is usually made of tungsten and, because of its high melting point, will not melt at high temperatures under normal circumstances. The electrons that are emitted by the filament are then directed toward the anode by the focusing cup. The tube current, or the number of electrons produced by the cathode, is determined by the temperature of the filament and is measured in milliamperes.
FIGURE 1-2. The x-ray tube. A: Cathode (C) with focusing cup. B: Cathode and anode. (Image B is from Figure 1-2 in Martins B: Radiation physics, biology and safety. Chapter 1 in Christman RA: Foot and ankle radiology, 1st edition, Churchill Livingstone, St. Louis, MO, 2003.)
The anode is the positive electrode of the x-ray tube (Figure 1-2B). Electrons produced at the cathode are directed toward a small tungsten target on the anode. The area on the target from which x-rays is emitted is known as the focal spot. The focal spot of lower extremity x-ray units is approximately 1 × 1 mm. Because a large amount of heat is generated by this bombardment of electrons and subsequent formation of x-rays, the anode (except for the target) is made of copper for efficient heat dissipation.
Anodes can be either stationary or rotating. General-purpose x-ray units operate at high tube currents (up to 1200 mA) and produce excessive amounts of heat at the target. The targets of these particular units are, therefore, located on a disk that rotates at a high rate. Heat can then be transferred across a larger surface area, thereby reducing the chance of damage to the tungsten target. In contrast, lower extremity–specific x-ray units operate at low tube currents (between 10 and 30 mA). Therefore, the targets of these units are attached to a less-expensive fixed, or stationary, anode. Production of x-rays at the anode is extremely inefficient; less than 1% of the kinetic energy from the projectile electrons is converted to x-rays, and the remainder (99%) is converted to thermal energy (heat).
The tube enclosure and protective housing absorb off-focus radiation that does not contribute to the useful x-ray beam. This is called inherent filtration. X-rays that do escape through the housing are referred to as leakage radiation, which can unnecessarily expose the patient and LXMO. As per the NCRP, leakage radiation from the tube housing at 1 m is not to exceed 100 mR/h (1 mGya/h).
The useful x-ray beam is also filtered, since it includes low-energy x-ray photons that do not contribute to the diagnostic image. This filter, added by the manufacturer, is known as added filtration and absorbs the low-energy x-ray photons that would otherwise be absorbed by the patient. Therefore, added filtration selectively absorbs low-energy x-rays that have no chance of reaching the image receptor, thereby increasing the active energy of the x-ray beam. It does not alter the quality of the radiographic image. Lower extremity x-ray units typically are supplied with 1.5- to 2.5-mm aluminum filters, meeting or exceeding the required total filtration for machines operating between 50 and 90 kVp. This thin sheet of aluminum is located between the x-ray tube housing and the collimator.
Beam Limitation Device
Beam limitation devices shape the dimensions of the useful x-ray beam so that only the area of interest is exposed to radiation. This process, known as collimation, also regulates scatter radiation that can fog the film (conventional radiography), degrade contrast resolution (digital radiography), and be absorbed by the patient. At the very least, the primary beam must be restricted to no more than the size of the image receptor being exposed. In the past, cones and diaphragms were used to limit the beam; variable-aperture collimators are now standard equipment on lower extremity x-ray units and allow the LXMO to further limit the x-ray field size.
Variable-aperture collimators use a light source that defines the field of the x-ray beam, hence the name light beam collimator (Figure 1-3). Depressing a small button activates the light source that remains on for a preset period of time. The tube head can then be positioned so that the x-ray beam is directed to the area in question. The area lit by the light source correlates to the area that will be exposed by the useful beam. The center of the light source is marked with crosshairs or a circle so that the central beam can be accurately positioned. Limiting the size of the x-ray beam is easily and accurately accomplished by adjusting shutters that controls the size of the x-ray beam. Variable-aperture collimators provided on lower extremity x-ray units usually consist of two sets of shutters or plates, oriented at 90° to one another, and are controlled by two knobs. A light source projects onto the patient so that the operator can adjust the aperture size and direct the useful beam over the body part of interest.
FIGURE 1-3. Variable-aperture collimator. (Courtesy of X-Cel X-Ray Corp., Crystal Lake, IL.)
X-ray Production
So far you have learned that electrons are generated at the cathode end of the x-ray tube at the filament; the focusing cup helps direct electrons toward the target, which is part of the anode; and the projectile electrons interact with the atoms of the tungsten target, which is where x-rays are produced.
X-rays are produced by two mechanisms: characteristic radiation and bremsstrahlung radiation. Characteristic radiation is produced when a projectile electron interacts with an atom’s inner-shell electron and removes that electron, resulting in ionization; x-rays are then produced as the outer-shell electrons fill the inner shells.
Bremsstrahlung radiation is produced when a projectile electron slows down or brakes as it passes by the positively charged nucleus. The electrons change course and lose kinetic energy, resulting in the production of x-rays. The word bremsstrahlung is German in origin and means “braking.”
The X-ray Emission Spectrum
X-ray quantity is the number of x-rays, also known as output intensity, x-ray intensity, or radiation exposure. X-ray quality refers to the penetrability of the x-ray beam, that is, how much energy it has. An x-ray beam that has an overall high energy is referred to as a “hard” x-ray beam, whereas a beam that has an overall low energy is considered a “soft” x-ray beam.
The x-ray emission spectrum refers to the range of x-ray energies present in a quantity of x-rays. X-rays produced by the characteristic radiation mechanism are very discreet in their energies. For example, an x-ray produced after interacting with a tungsten atom by ejecting a K-shell electron that is replaced by an L-shell electron is 57 keV. An x-ray produced after an L-shell electron is replaced by an M-shell electron is only 9 keV.
In contrast, x-rays produced by the bremsstrahlung mechanism produce a continuous x-ray energy spectrum. For example, if the kVp setting is at 70, x-rays will be produced between 0 and 70 kVp; therefore, since attenuation of the x-ray beam is responsible for the formation of the diagnostic image, the heterogeneous bremsstrahlung radiation x-rays are going to be much more valuable than those produced by characteristic radiation.
The x-ray emission spectrum is influenced by mA (or mAs), kVp, and added filtration. Increasing mA (or mAs) increases the quantity of the x-ray beam but does not influence its quality. An increase in kVp results in an increase of both the quality and quantity of the x-ray beam. The presence of added filtration results in a decrease of the x-ray beam quantity but an increase in quality.
Half-Value Layer
The half-value layer (HVL) is a measurement of x-ray quality. The HVL of an x-ray beam is defined as the thickness of absorbing material necessary to reduce the x-ray intensity to half its original value. Recall that added filtration removes the low-energy x-ray photons that would otherwise be absorbed by the patient; this increases beam quality (HVL) but decreases the quantity of x-rays. Even though some very low-energy x-rays are absorbed by the tube housing (inherent filtration), the NCRP recommendations and state regulations require the use of added filters (usually made of aluminum) to harden the beam. The minimum amount of total filtration (inherent plus added) and the recommended HVLs are listed in Box 1-5.
BOX 1-5 Minimum Total Filtration and HVLs Recommended by the NCRP
kVp | Total Filtration (mm Al) | HVL (mm Al) |
49 | 0.5 | 0.5 |
50 | 1.5 | 1.2 |
60 | 1.5 | 1.3 |
70 | 1.5 | 1.5 |
71 | 2.5 | 2.1 |
80 | 2.5 | 2.3 |
90 | 2.5 | 2.5 |
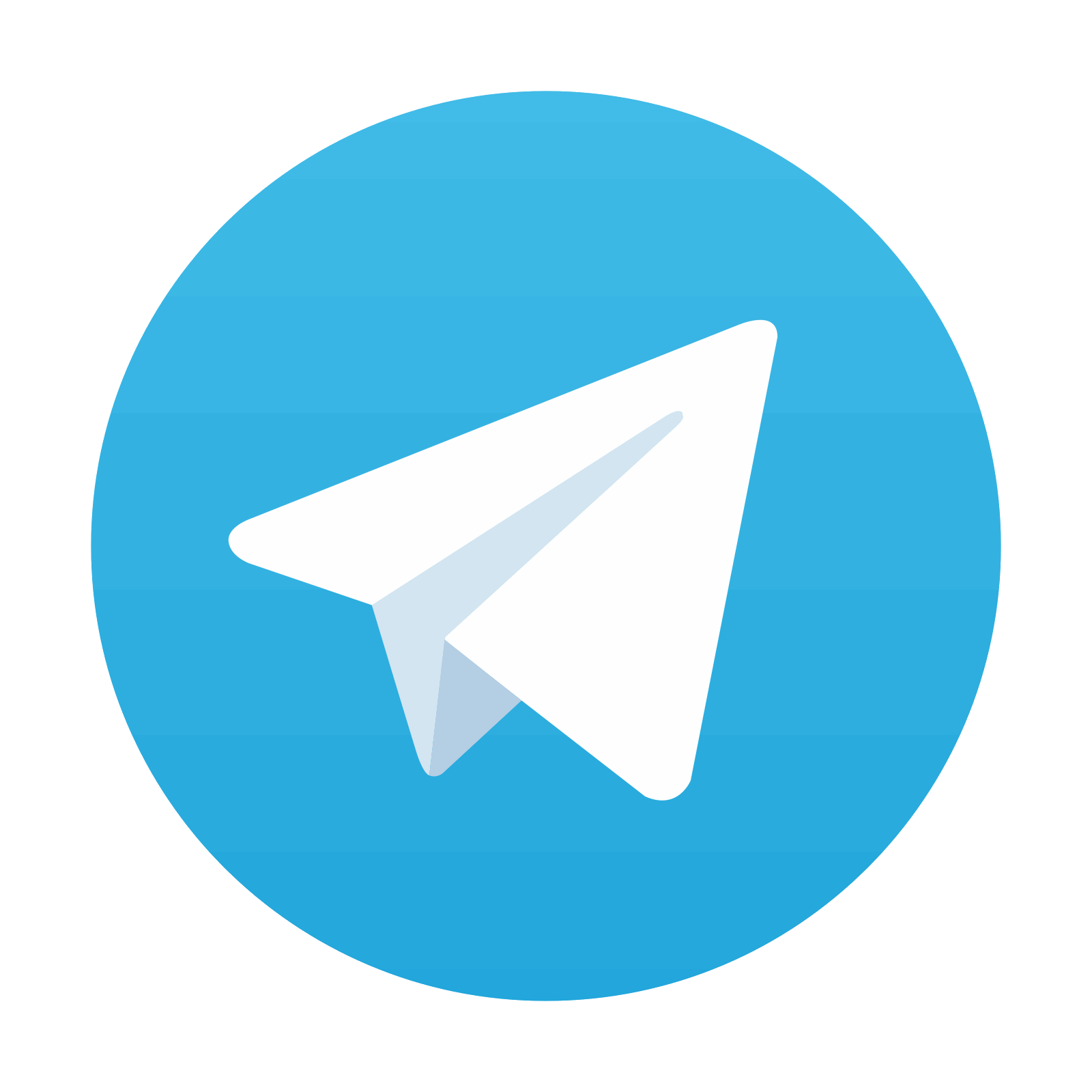
Stay updated, free articles. Join our Telegram channel

Full access? Get Clinical Tree
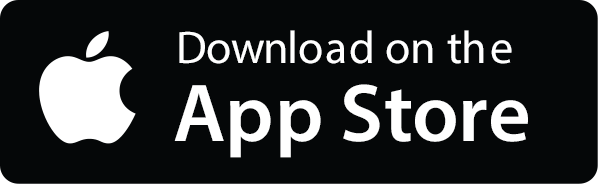
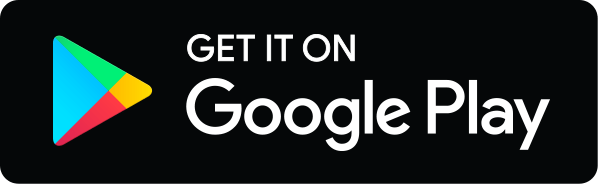