Chapter 33 In 1987 Galibert et al1 described a procedure, “vertebroplasty,” in which polymethylmethacrylate (PMMA) cement was injected into the vertebral body for the treatment of painful hemangioma using a percutaneous, transpedicular approach. Deramond et al2 reported acute pain relief in 80% of the patients treated with PMMA vertebroplasty for osteoporotic vertebral compression fractures. Other applications of the procedure included treatment of pain and imminent vertebral collapse in malignancies, myelomas, and metastases. The complication rate was low (1%), but included leakage of cement to surrounding tissue, pulmonary embolism, rib fracture, blood loss, radicular pain, and damage to the spinal cord. The method has been adopted on a larger scale in the early 1990s for the treatment of osteoporotic vertebral compression-type fractures (VCF), producing similar results in prospective studies.3–6 The mechanism of pain relief is not elucidated completely. Both mechanical and chemical properties of the PMMA cement may account for the beneficial effects. The stability provided by the cement prevents the painful micromotion of bone in the fractured vertebral body. Additionally, pain reduction could result from thermal destruction of nerve endings or tumor tissue in the vertebra.7–9 Shortcomings of vertebroplasty are the requirement for high-pressure injection of the cement, thereby increasing the risk of cement leakage, and the inability to restore vertebral body height. These shortcomings have been addressed by the so-called “kyphoplasty,” a technique described in 1998, for the restoration of collapsed osteoporotic vertebral bodies.10 In this technique, inflatable bone tamps are percutaneously inserted transpedicularly in the vertebral body prior to cement injection. By inflating the balloons, fracture reduction and height restoration of the vertebral body can be achieved. After deflating and removing the bone tamps, PMMA cement can be injected. The first prospective studies (maximum follow-up 18 months) showed good clinical outcome with 90% of the treated patients reporting acute pain relief and the same risk for complications as with traditional vertebroplasty.11–13 PMMA is the most used cement for injection in the vertebral body, but the use of this cement as a bone defect filler in vertebroplasty is under discussion. The advantages of this cement are the well-described chemical and mechanical characteristics, the pain reduction ascribed to the exothermal nature of polymerization, and the mechanical stability (resistance to compressive force) provided after vertebroplasty. However, insufficient data exist on the long-term behavior of a large volume of the biologically inert cement in a vertebral body. Several studies suggest that the osteolysis, frequently seen in PMMA-cemented total hip replacement, is caused by a PMMA-induced, inflammatory (increased macrophage/osteoclast activity) response.14,15 With respect to the exothermal polymerization reaction, supraphysiological temperature causes tissue necrosis. Although thermal damage of the spinal cord is a rare complication, Deramond et al6 showed temperatures to rise above 50°C in cadaveric vertebral bodies, underlining the need for prevention of cement leakage. Furthermore, during practice, many physicians found the viscosity of PMMA too high for controlled injection. Deviating from the manufacturer’s preparation instruction in search of less viscosity can significantly impair mechanical stability of PMMA cement.16 VCF, resulting from axial impact, can be treated conservatively or surgically, depending on the severity as assessed by radiological and neurological examination and on the opinion of the surgeon. Patients with osteoporosis, regardless of the cause of the disorder, leading an active life for an extended period are exposed to an increased risk of suffering one or more traumatic VCF during their lifetime (Fig. 33–1).17 Traumatic fractures involving a burst of the vertebral body (A3-type fracture according to AO classification18) are treated preferably by surgical intervention in our center. Posterior stabilization using rods and pedicle screws has gained popularity over the last years, due to the relatively simple procedure, the limited number of motion segments that are fixed, and the good clinical outcome.19 Speth et al20 reported on complications of this treatment, such as failure of the instrumentation during the postoperative period or recurrent kyphosis after removal of the instrumentation (Figs. 33–2A through C). Oner et al21 concluded that narrowing of the intervertebral disc space was characteristic for patients in which treatment failed. From a serial publication by Oner,22 it was also concluded that recurrent kyphosis and narrowing of the disc space are a result of creeping of the disc into a central depression of the bony end plate, rather than degeneration of the disc itself.22 The basis for this mechanism is formed by the anatomy: the disc is contained by the annulus fibrosis and by the end plates. Traumatic changes in the containing structures of the disc space probably result in a redistribution of disc tissue, due to the swelling pressure, into the available space. Following this hypothesis optimal reduction of the fracture, especially the end plate, would prevent this morphologic change. Restoration of the central part of the end plate is performed, ideally after distraction of the fracture with pedicle screws through a transpedicular approach. The void that results in the vertebral body could be augmented with an injectable osteoconductive or osteoinductive material, which supports the intervertebral disc and prevents migration of disc tissue into the vertebral body. Since the material will be used in combination with a posterior fixation device, it will not be under full load bearing during the healing period. FIGURE 33–1 CT scan of a 56-year-old male 7 years after successful treatment of multiple myeloma with chemotherapy, irradiation, and bone marrow transplantation, showing severe osteoporosis and multiple collapsed vertebral bodies. It is our opinion that the patient who suffers a traumatic or osteoporotic VCF and has a long active period ahead benefits when a more biocompatible cement, as compared with PMMA cement, is used as a bone void filler. This bone void filler would ideally augment the vertebral body, conduct new bone formation, and eventually be replaced by bone. Previous studies demonstrated that augmentation of the defect with autologous cancellous bone does not provide sufficient initial stability and can be a potentially dangerous procedure.23,24 Due to vascular insufficiency, the graft becomes ischemic and necrosis may result in time. Furthermore, the harvesting of an autologous graft is associated with well-known donor-site morbidity. The choice for calcium-phosphate bone cement in the experimental procedure is justified by its biomechanical and chemical properties.25 Such bone cements are currently being used in neurosurgery and ENT surgery.26,27 It serves as an osteoconductive and possibly as a biodegradable bone substitute in defects too large to be covered by the natural process of bone healing (“critical size defect”) and where initial mechanical stability (compression forces) is required. In an aqueous environment, the main (powder-form) components of the cement react to form hydroxyapatite as a final product, which is the principal mineral in bone (and teeth). This property makes calcium-phosphate cement (Ca-P) biocompatible with both soft and hard tissues. In animal studies, this cement is resorbed over time by osteoclastic activity and replaced with bone. The cement’s consistency before hardening gives the opportunity to preform the cement before application. Ca-P is isothermal in its hardening phase, thereby decreasing the risk of thermal damage to neural structures in case leakage occurs. The mechanical properties (resistance to compressive force and stiffness) of Ca-P as compared to PMMA did not show significant differences after in vitro vertebroplasty.28 However, shear forces are not very well tolerated by Ca-P, making it more suitable for applications in which it is “contained” and thus is exposed mainly to compressive forces. FIGURE 33–2 (A) Preoperative radiograph of a 32-year-old female with a traumatic vertebral compression fracture due to a car accident. (B) Postoperative radiograph of the same patient after reduction and posterior instrumentation. (C) Recurrent kyphosis following removal of the hardware 1.5 years after initial trauma. A device has been developed in which part of a cadaveric osteoligamentous human spine can be mounted in polyurethane cups. To create type A fractures (according to the AO classification by Magerl et al18), the device allows for an axial impact on the specimen by dropping a weight (10 to 20 kg) from a height of 1.50 m on the top cup. A wedge, bolted to the weight, hits the anterior part of the spine first before axial impaction, to simulate the impact that results in a wedge-formed vertebral compression-type fracture. In a first study, we used a total of five fresh human cadaveric spines (T8 to S1) from donors 60 to 69 years of age. All soft tissue, except the ligaments, was removed. All spines were divided at the L1-L2 level, which resulted in a total of nine specimens (five thoracolumbar and four lumbar) that were used to create compression-type fractures. Magnetic resonance (MR) imaging (T1 and T2 weighted with a 0.5 Tesla scanner) was performed for prefracture assessment of the spine (T1). To confirm and classify the fractures that were created with the device, plain radiographs [anteroposterior (AP) and lateral] and MR images were obtained (T2). Subsequently, all fractures were treated, using the standard surgical procedure in our center, by short-segment posterior fixation and distraction using titanium pedicle screws and rods (BWM system, Stryker-Howmedica-Osteonics, Rutherford, NJ). Again, plain radiographs and MR images were obtained (T3).
VERTEBROPLASTY WITH INFLATABLE
BONE TAMPS AND CALCIUM-
PHOSPHATE CEMENT
TRAUMATIC AND OSTEOPOROTIC VERTEBRAL COMPRESSION-TYPE FRACTURES
INJECTABLE CALCIUM-PHOSPHATE CEMENTS IN VERTEBROPLASTY
STUDIES ON HUMAN CADAVERIC SPINES
EXPERIMENTAL FRACTURE DEVICE AND CREATION OF FRACTURES
DIRECT REPOSITIONING OF
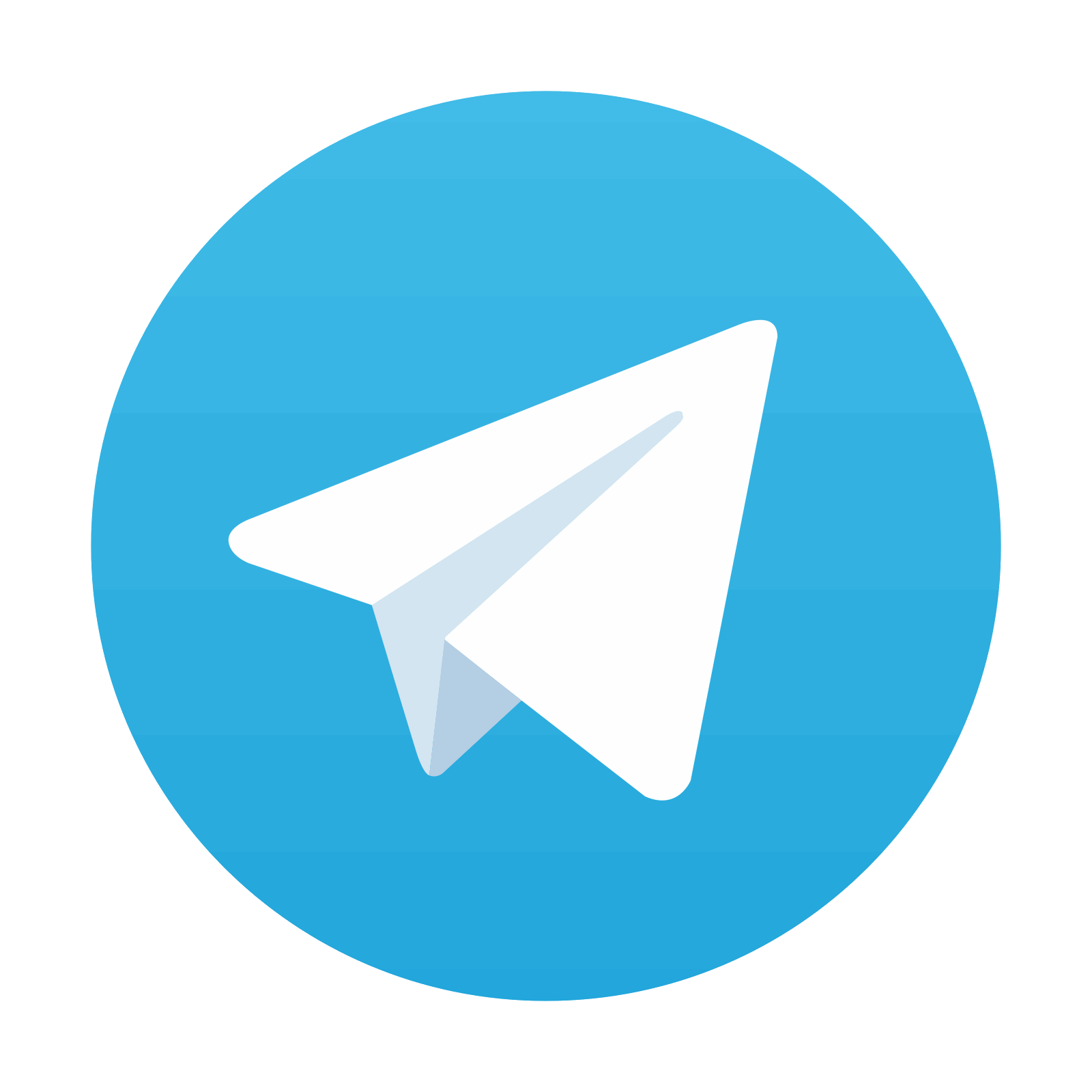
Stay updated, free articles. Join our Telegram channel

Full access? Get Clinical Tree
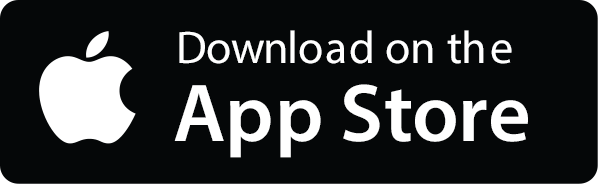
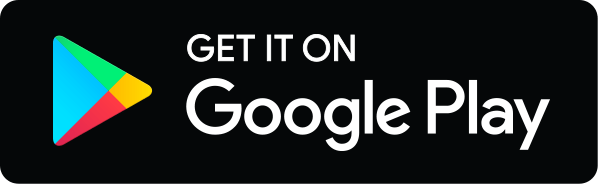