Chapter Eight Upper cervical spine
Combined movements and the upper cervical spine
Clinical anatomy and biomechanics
Innervation; pain generators and pain patterns of the cranio-cervical region
Stability of the cranio-cervical region
Assessment of the upper cervical spine
Testing mechanical stability of the cranio-cervical spine
Assessment of increased tone of cervical muscle tissue
Assessment for Grade IV−: manipulation of the upper cervical spine
Introduction
Due to its unique regional anatomy and complicated biomechanics, the cranio-cervical region presents something of a challenge for manual therapists. It is the most mobile region of the spine; this allows accurate positioning of the main sensory organs but also predisposes the region to a wide range of disorders and increases its vulnerability to trauma and degenerative changes (Konig et al, 2005). In addition, the upper cervical spine has a unique pattern of innervation and a close relationship with the brainstem, spinal cord, cranial nerves and vertebral arteries (Standring, 2008). Consequently, dysfunction in the cranio-cervical region can manifest in a wide variety of unusual symptoms including nausea, headache, dizziness, and head, neck or facial pain (Kerry & Taylor, 2006; Maak et al, 2006; Nguyen et al, 2004; Swinkels & Oostendorp, 1996).
This combination of factors may make clinicians wary of managing conditions originating from the upper cervical spine. However, with carefully directed manual therapy it is possible to alleviate many of the distressing and often debilitating symptoms experienced by patients with neuromusculoskeletal disorders of the cranio-cervical region (Jull et al, 2008).
Combined movements and the upper cervical spine
Owing to the size, shape and orientation of upper cervical joint surfaces and the arrangement of the surrounding soft tissues, cranio-cervical movements occurring in one plane will result in three-dimensional movement patterns (Brolin & Haldin 2004; Catrysse et al, 2008; Panjabi et al, 1988). The combination of movements involved in this pattern is termed the ‘coupling’ or ‘coupled movement’ pattern and refers to all movements that take place alongside the primary movement (Cook et al, 2006; Ishii et al, 2006). Knowledge of the coupling patterns of the cranio-cervical junction forms the basis of examining and treating upper cervical spinal disorders using combined movement theory (CMT).
The pattern of coupled movements in the upper cervical spine is largely opposite to the pattern observed in the lower cervical spine and it has been shown to be affected by the specific cranio-cervical anatomy, the overall upper cervical posture, the initiating movement, the pattern of neuromuscular activation, and the presence of local degenerative changes (Cook et al, 2006; Edmonston et al, 2005; Panjabi et al, 1993). These factors need to be taken into consideration when assessing or treating the upper cervical spine using CMT.
Clinical anatomy and biomechanics
The cranio-cervical region is comprised of the occiput (C0) and the upper two cervical vertebrae known as the atlas (C1) and the axis (C2). Both these vertebrae are markedly atypical (Middleditch & Oliver, 2005).
The atlas
The atlas (Fig. 8.1) is a ring-shaped vertebra without a vertebral body or spinous process. It has two superior and two inferior articulating facets which articulate with the occiput above and the axis below. In addition it has a central facet on the inside of the anterior atlas arch which articulates with the odontoid peg of C2. The atlas has an antero-posterior (A-P) diameter of approximately 3 cm which means that the spinal canal at this level is larger than anywhere else in the vertebral column (Ebraheim et al, 1998). The odontoid peg, transverse ligament and spinal cord occupy two thirds of the A-P diameter, leaving 1 cm of free space. This allows the large ranges of motion (ROM) to occur across the cranio-cervical junction without encroachment onto the spinal cord.
The axis
The axis (Fig. 8.2) is the strongest and the most irregularly shaped cervical vertebra with a large vertical projection arising from the superior surface of the body of the vertebra called the dens or odontoid process. The odontoid process has an anterior and a posterior facet for articulation with the anterior arch of the atlas and the transverse ligament respectively. Laterally, the axis has two large upward and laterally-orientated articulating facets on its superior surface for articulation with the atlas above (Standring, 2008; Taylor & Twomey, 2002).
The occipito-atlanto-axial complex
Approximately one third of the total cervical sagittal plane movement takes place in the upper cervical spine with the movements of flexion/extension predominantly occurring at C0–C1 (Chancey et al, 2007; Jull et al, 2008). These movements are facilitated by the orientation of the joint surfaces between the occiput and the atlas, and are larger in range than at lower cervical levels.
Rotation is the dominant movement at C1–C2 and accounts for over half of the total cervical transverse plane movement. The amount of rotation at the atlanto-axial (A-A) joint is significantly larger than at any other vertebral level and again this is mainly due to the configuration of the articular surfaces (Cattrysse et al, 2008; Ishii et al, 2004; Ordway et al, 1999). Side-flexion occurs across both the atlanto-occipital (A-O) and A-A joints but, in contrast, it is smaller in range than at sub-axial cervical levels (Ishii et al, 2006; Panjabi et al, 2001).
The primary movements of the upper cervical spine are generally coupled with secondary movements or translations (Cattrysse et al, 2007; Ishii et al, 2006). The most significant coupled movement in the cranio-cervical complex is rotation which is coupled with lateral flexion in the opposite direction. This primarily occurs at the A-A joint but also happens to a much lesser extent at the O-A joint (Ishii et al, 2004). The primary movements of flexion/extension are accompanied by small, secondary plane movements of lateral flexion and rotation in a contralateral pattern (Amiri et al, 2003; Bogduk & Mercer, 2000). The main coupled translation which takes place across the occipito-atlanto-axial complex is lateral translation at C1–C2 during side-flexion; this occurs in the same direction as the side-flexion movement (Ishii et al, 2006).
Atlanto-occipital joint
The atlanto-occipital (A-O) joint (Fig. 8.3) is essentially a uni-planar joint consisting of a pair of synovial condyloid joints between the two convex occipital condyles on the base of the skull and the two large reciprocally concave superior facets on the lateral masses of the atlas (Standring, 2008).
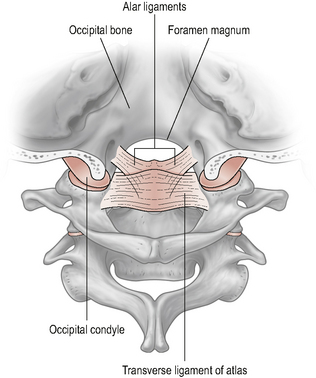
Figure 8.3 • The atlanto-occipital joint.
(Reproduced from Drake et al (2005) with permission from Elsevier)
The joint surfaces are relatively congruous. They are long in a posterior–anterior (P–A) direction and narrow mediolaterally. This, together with the sagittal plane orientation of the joint and the reciprocally curved articular surfaces, primarily facilitate the movements of flexion and extension, or nodding, of the head on neck (Bogduk & Mercer, 2000). In addition, the shape of the joint surfaces is such that the anterior ends project higher than the posterior ends; this configuration permits a greater range of extension than flexion (Chancey et al, 2007; Panjabi et al, 2001).
Kinematics of atlanto-occipital flexion and extension
Movement analysis studies of the upper cervical spine (in vitro and in vivo) report a variable range of flexion–extension at the A-O joint. Combined findings would suggest that the range of flexion is approximately 3.5°–7.0° and the range of extension is between 16.5° and 21.0°. This gives a total sagittal plane ROM at the C0–C1 segment of approximately 20°–28° (Amiri et al, 2003; Ordway et al, 1999; Panjabi et al, 1988, 2001). Given that the total range of flexion–extension at the C5–C6 segment is reported to be approximately 6°–10° (Goel et al, 1984; Goel & Clausen, 1998; Moroney et al, 1988; Panjabi et al, 2001) the C0–C1 segment demonstrates one of the largest amounts of combined flexion–extension available at any one vertebral segment.
During upper cervical flexion, the occipital condyles roll forwards and slide backwards on the atlas (Bogduk & Mercer, 2000). This movement tightens and is limited by tension developed in the posterior part of the joint capsules, the posterior neck muscles, the posterior A-O membrane, and the ligamentum nuchae. The opposite occurs during upper cervical extension. The occipital condyles roll backwards and slide forwards on the atlas (Bogduk & Mercer, 2000). This movement is mainly limited by approximation of the occiput with the sub-occipital muscles and the posterior arches of the atlas and axis but may also be restrained by tension in the anterior A-O membrane and joint capsules (Bogduk & Mercer, 2000; Palastanga et al, 2006) (Fig. 8.4).
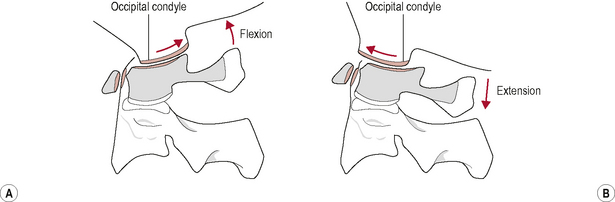
Figure 8.4 • (A) Kinematics of the atlanto-occipital joint in flexion and (B) extension.
(Reproduced from Palastanga et al (2006) with permission from Elsevier)
Flexion–extension at the A-O joint occurs during flexion–extension of the entire cervical spine but has been found to be at a maximum during the movements of upper cervical retraction (a posterior translation of the entire head, or ‘chin in’ position) and protraction (a forward translation of the entire head, or ‘chin out’ position) respectively (Ordway et al, 1999). Maximum stretch to the posterior peri-articular tissue of the A-O joint will occur in upper cervical retraction and to the anterior peri-articular structures in protraction. Clinically, it would seem appropriate to use these upper cervical movements in the assessment of the A-O joint and surrounding peri-articular structures.
Kinematics of atlanto-occipital lateral flexion and rotation
Studies suggest that the available range of lateral flexion to each side is in the region of 1.9–4.5° and the amount of rotation to each side is approximately 1.7–4.9° (Bogduk & Mercer, 2000; Goel et al, 1988; Ishii et al, 2004, 2006; Panjabi et al, 2001). Results from in vivo studies generally produce measurements towards the lower end of the reported range whilst in vitro studies provide greater values for side-flexion and rotation; this is likely to be due to the contribution of physiological muscle tone and the effect of posture on ROM. For clinical purposes it would appear reasonable to consider that the available range of planar side-flexion and rotation at the A-O joint is approximately 2–3° to each side.
Both these movements are checked by the contralateral alar ligament and the A-O joint capsules (Bogduk & Mercer, 2000; Brolin & Halldin, 2004; Crisco et al, 1991b; Krakenes & Kaale, 2006).
Coupling of movements at the atlanto-occipital joint
Planar movements of the A-O joint have been considered individually but it is perhaps of greater clinical relevance to consider these movements in combination as they would occur during normal upper cervical movement patterns. It is generally agreed that the coupling pattern of movement in the upper cervical spine is predominantly contralateral, particularly if rotation is the initiating movement (Amiri et al, 2003; Cook et al, 2006; Ishii et al, 2004). This is in contrast to the ipsilateral coupling of side-flexion and rotation predictably observed in the sub-axial cervical spine (Cook et al, 2006).
The primary movements of the A-O joint of flexion–extension are accompanied by negligible amounts, approximately 2–3°, of rotation and side-flexion coupled in a contralateral pattern (Amiri et al, 2003). Clinically, these findings suggest that when the A-O joint is examined using combined movements a more consistent outcome will be achieved if the primary movements of flexion and extension are combined with rotation first and then with contralateral lateral flexion. In addition, in order to ‘lock’ the A-O joint the segmental position opposite to the coupled motion will be required, i.e. a combined position of flexion–extension plus rotation and ipsilateral side-flexion.
Atlanto-axial joints
The A-A joint (Fig. 8.5) is comprised of three articulations between the atlas and the axis: two lateral and one central articulation (Palastanga et al, 2006; Standring, 2008).
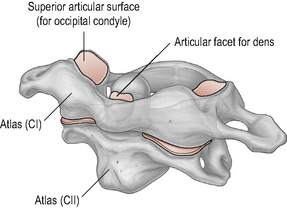
Figure 8.5 • The atlanto-axial joint.
(Reproduced from Drake et al (2005) with permission from Elsevier)
The central component of the A-A articulation is a synovial pivot joint where the odontoid peg of the axis articulates within an osseoligamentous ring formed by the anterior arch of the atlas anteriorly and the strong, thick transverse ligament posteriorly. Articular cartilage covers the parts of the odontoid peg that articulate with the atlas and the transverse ligament and surrounding these areas are two separate synovial cavities (Middleditch & Oliver, 2005; Palastanga et al, 2006).
The arrangement of the three A-A joints predominantly facilitates the movement of rotation, where the head and atlas move together as a single unit rotating around the axis. In addition, due to the biconvex nature of the facet joints, small amounts of flexion–extension and lateral flexion also occur at this level (Bogduk & Mercer, 2000; Palastanga et al, 2006).
Analysis of the amount of motion and coupling patterns that occur at this joint is challenging due to the large rotational component involved. Many motion analysis studies have used two-dimensional or in-vitro study designs which, for different reasons, are not fully able to report accurate amounts of rotation or coupling patterns (Ischii et al, 2004). More recently, three-dimenisonal in-vivo studies have been carried out which provide a better picture of the range and kinematics involved during A-A rotation.
Kinematics of atlanto-axial rotation (Fig. 8.6)
Combined findings from in vivo and in vitro movement analysis studies report a unilateral range of A-A rotation between 38° and 56° (Iai et al, 1993; Ishii et al, 2004; Panjabi et al, 2001). This large range of segmental rotation is in part made possible by the configuration of the A-A joint surfaces, the lack of an IVD and the loose joint capsules, and is in contrast to the smaller ranges of unilateral rotation of 5–7° observed sub-axially (Iai et al, 1993; Mimura et al, 1989). The total amount of unilateral cervical rotation from C0 to C7 is reported to range from 60° to 70° (Edmonston et al, 2005). This means that approximately 60% of the total range of cervical rotation occurs at the A-A joint (Ishii et al, 2004). As a consequence, degenerative changes are seen at this joint with a much higher frequency than at the A-O joints (Konig et al, 2005).
In addition to its major contribution to cervical rotation the A-A joint has also been shown to play a key role in the initial phase of head rotation, with rotation in the sub-axial spine occurring only after the movement at C1–C2 is completed (Hino et al, 1999; Ishii et al, 2004). This may be clinically helpful in that if pain is produced on cervical rotation early in range it is likely that the dysfunction lies within the upper cervical spine; conversely, pain arising on cervical rotation later in range is likely to originate from sub-axial structures. This sequential motion pattern, however, becomes disorganized in patients with cervical segmental instability where motion in the unstable segments has been observed to precede motion in intact upper segments (Hino et al, 1999).
Rotation in the sub-axial spine is limited by the IVD disc and articular facets. The A-A joint, however, lacks an IVD and has relatively flat joint surfaces. Rotation at this level therefore is predominantly limited by ligamentous structures, in particular the contralateral alar ligament (Crisco et al, 1991a). Damage to these ligaments may permit rotational hypermobility of the A-A joint and subsequently threaten closely associated structures such as the vertebral arteries and spinal cord (Crisco et al, 1991b; Goel et al, 1990; Kerry & Taylor, 2006). Motion analysis studies suggest that 56° is the upper limit of normal unilateral rotation in the cranio-cervical region. Movement beyond this may indicate rupture of the contralateral alar ligament and thus a rotational instability of the upper cervical spine (Dvorak et al, 1987).
Kinematics of atlanto-axial flexion–extension (Fig. 8.7)
There are few current studies reporting on the movements of flexion–extension at the A-A joint. Values for these ROMs have mainly been obtained from in vitro studies. Overall, there is consensus that the total range of sagittal plane movement is greater at the A-O segment than at the A-A segment. Analyzing the movements separately, however, shows that flexion is greatest at the A-A joint and extension is greatest at the A-O joint (Bogduk & Mercer, 2000). Approximate ranges for A-A flexion are from 6°–12° and extension from 5°–10° with in vivo studies generally recording smaller ROMs (Chancey et al, 2007; Ordway et al, 1999; Panjabi et al, 2001). A greater range of flexion–extension at the C1–C2 joint is produced through the upper cervical movements of retraction and protraction respectively (Maeda et al, 2004; Ordway et al, 1999).
Kinematics of atlanto-axial lateral flexion
When lateral flexion is the initiating movement at the C1–C2 segment, it has a range of approximately 5°–10° and is coupled with extension and contralateral rotation, although this has not been shown to be a fully consistent pattern (Bogduk & Mercer, 2000; Cattrysse et al, 2008; Cook et al, 2006; Iai et al, 1993).
The main restraints to lateral flexion between C1–C2, and indeed across the whole complex, are the contralateral alar ligaments (Krakenes & Kaale, 2006). It has been suggested that, since axial rotation is consistently coupled with contralateral side-flexion, upper cervical rotation to one side is sufficient to tighten the alar ligaments on both sides and that the alar ligaments may be adequately tested using the movement of upper cervical rotation only (Crisco et al, 1991a). This theory has yet to be substantiated.
Coupling of movements at the atlanto-axial joint
In basic terms, during right rotation of the A-A joint the right inferior articular surface of C1 moves backwards on the right superior facet of C2. The reverse happens on the left, the left inferior articular surface of C1 moves forward on the left superior facet of C2. The effect of this is to increase the stretch on the anterior and posterior peri-articular structures of the left and right facet joints (Edwards, 1999).
However, due to the biconvex nature of the A-A joint surfaces, axial rotation at C1–C2 is not a pure movement, but exhibits a convex on convex behaviour. In the neutral joint position, the A-A joint surfaces contact at the height of the convexity. During right rotation, the right lateral mass of C1 will move backwards and medially as it slides down the posterior slope of its atlantial facet whilst the left lateral mass of C1 will move forward and medially as it slides down the anterior slope of its facet. This results in a double-threaded screw-like mechanism producing a vertical drop or translation at the A-A joint. This action slackens the alar ligaments and delays their limiting effect on rotation. Accompanying this vertical translation is a contralateral lateral bending of approximately 2–3° together with either flexion or extension (Cook et al, 2006; Ishii et al, 2004; Iai et al, 1993; Panjabi et al, 1993).
Whether flexion or extension occurs at C1–C2 during axial rotation depends on the position of the upper cervical spine. If the upper cervical spine is in an extended or protracted position, axial rotation will be coupled with extension; conversely if the upper cervical spine is held in a flexed or retracted position the coupled motion with axial rotation will be flexion (Edmonton et al, 2005; Panjabi et al, 1993). This key feature occurs due to the passive nature of C1 under axial loads from the head. The atlas is not bound to the axis by any ligaments and there are few muscles that act directly upon it; for this reason the atlas has frequently been likened to a passive ‘washer’ between the occiput and C2. As a result, the secondary movements occurring at the A-A joint are, in part, related to where the occiput sits on the atlas with reference to the line of gravity. These movements are termed ‘paradoxical’ movements (Bogduk, 2002a; Bogduk & Mercer, 2000; Iai et al, 1993). Head position therefore significantly affects movement patterns of the upper cervical spine and should be considered when testing segmental motion or treating joint limitations in this region.
Coupling in the upper cervical spine and posture
It has been suggested by several authors that the contralateral coupling pattern seen in the upper cervical spine is, in part, a compensatory mechanism for the ipsilateral coupling pattern seen in the lower cervical spine in order to keep the head upright and facing forwards (Cook et al, 2006; Edmonston et al, 2005; Iai et al, 1993).
The available ROM and also the coupling pattern of movements in the occipito-atlanto-axial complex have been shown to be affected by the overall position of the head and neck (Cook et al, 2006; Panjabi et al, 1993). Available ROM in the upper cervical spine is decreased if movements are carried out in positions of protraction or retraction rather than in neutral head positions and patterns of secondary movements, particularly at the C1–C2 segment, are altered. Both these factors have a consequential effect on coupling patterns in the lower cervical spine (Edmonston et al, 2005). Clinicians should therefore carefully consider the position of the cranio-cervical spine during assessment and treatment of the cervical spine as this may affect the outcome.
SUMMARY
Cranio-cervical anatomy and biomechanics
• Upper cervical extension occurs predominantly at C0–C1.
• Upper cervical rotation occurs predominantly at C1–C2.
• Flexion of the craniovertebral junction is almost equally distributed between C0–C1 and C1–C2.
• When assessing flexion–extension in the upper cervical spine, manual examination should be focused on the A-O joint.
• When assessing rotation in the upper cervical spine, manual examination should be focused on the A-A joint.
• Full range of upper cervical flexion occurs during retraction.
• Full range of upper cervical extension occurs during protraction.
• Movements of retraction and protraction should be included in an upper cervical assessment in order to fully stress the surrounding soft tissues.
• The coupling pattern in the upper cervical spine is predominantly contralateral.
• Using the coupling pattern of flexion–extension and rotation will most effectively examine the A-O joint using CMT.
• Using the coupling pattern of rotation and flexion–extension will most effectively examine the A-A joint using CMT.
• Pain on cervical rotation early in range is likely to be the result of an upper cervical dysfunction.
• Pain on cervical rotation beyond 45° is likely to be a sub-axial cervical dysfunction.
• Unilateral rotation between C1–C2 of greater than 56° suggests that the structures limiting rotation (predominantly the alar ligaments) are incompetent.
• Upper cervical posture affects cervical ROM and coupling pattern and should be considered prior to cervical spine examination.
Innervation; pain generators and pain patterns of the cranio-cervical region
It is widely accepted that pain can be sub-classified based on the hypothesized mechanism of production into peripheral nocioceptive, peripheral neurogenic, central sensitization or affective categories (Butler, 2000; Gifford & Butler, 1997; Loeser & Treede, 2008). This classification system can be applied to pain arising from the head, neck or face region. An understanding of the characteristic patterns of presentation for the different sub-categories of pain will allow the manual therapist to identify the dominant pain mechanism in operation and will thus help direct appropriate assessment and management strategies.
For a detailed review of the different pain mechanisms, the reader is referred to alternative texts (Butler, 2000; Gifford & Butler, 1997; Loeser & Treede, 2008). In brief, cranio-cervical or facial pain can be classified into:

(Central sensitization and affective mechanisms of pain generation will not be considered here.)
Cranio-cervical somatic tissue as a pain generator
Somatic structures of the cranio-cervical spine are innervated by dorsal (posterior) and ventral (anterior) branches of the first three cervical spinal nerves. Studies carried out on symptomatic and asymptomatic subjects have collectively demonstrated that irritation or dysfunction of any structure innervated by the three upper cervical nerves can potentially generate local pain or referred pain to the head, face, occipital and sub-occipital region (Bogduk, 2001; Dreyfuss et al, 1994; Dwyer et al, 1990).
On exiting, the intervertebral foramina spinal nerves divide into dorsal and ventral rami. Generally, the dorsal rami innervate structures that lie posterior to the intevertebral foramina and nerve roots (neuraxis) and the ventral rami innervate structures that lie anterior to the neuraxis (Bogduk, 2002b) (Fig. 8.8). Table 8.1 provides a summary of the structures supplied by the dorsal and ventral rami.
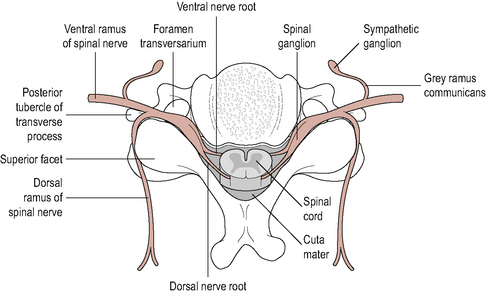
Figure 8.8 • A cross section through a vertebral level to show the spinal cord, spinal nerves, ventral and dorsal rami
(Reproduced from Middleditch & Oliver (2005) with permission from Elsevier)
Ventral rami of C1–C3 supply | Dorsal rami of C1–C3 supply |
---|---|
Craniocervical flexors | Suboccipital muscles |
Scalenes | Posterior neck muscles |
Levator scapulae | Skin over craniocervical spine and occiput |
Trapezius | Ligamentum nuchae |
Atlanto-occipital joint | |
Atlanto-axial joint | |
Transverse ligament; alar ligament; tectorial membrane | |
Upper cervical dura mater | |
Skin around ear and anterolateral part of neck |
Ventral rami innervation (Fig. 8.9)
The cervical plexus is formed by the ventral rami of the upper four cervical nerves and consists of deep muscular branches which supply the muscles and superficial branches which supply the skin (Standring, 2008). The deep branches of the cervical plexus innervate the pre-vertebral muscles (cranio-cervical flexors) as well as the scalenes; levator scapulae; trapezius and sternocleidomastoid. The A-O and A-A joints which, unlike the facet joints in the lower cervical spine, lie anterior to the intervertebral foramina, are also supplied by the cervical plexus, more specifically the ventral rami of the C1 and C2 spinal nerves respectively (Dreyfuss et al, 1994). The ligaments of the A-A region (transverse, alar and tectorial membrane) and the dura mater of the upper cervical cord are innervated by the upper three sinuvertebral nerves (SVN) (Johnson, 2004). SVNs are branches from the ventral rami which re-enter the intervertebral foramina and ascend or descend as many as four vertebral segments within the vertebral canal. As a consequence of this, they can produce varied pain patterns, making it difficult to localize the source of the pain.
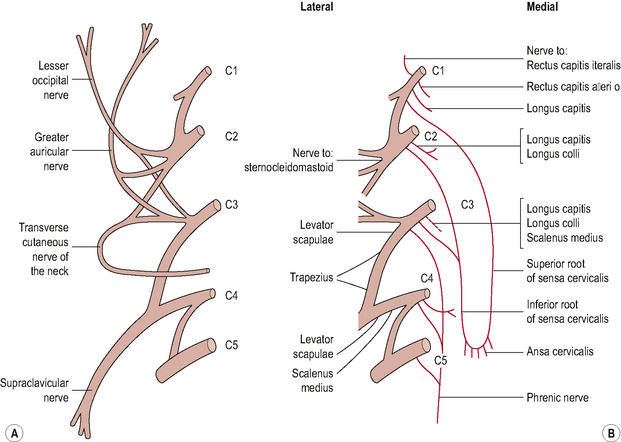
Figure 8.9 • (A) The cervical plexus superficial branches and (B) deep branches.
(Reproduced from Palastanga et al (2006) with permission from Elsevier)
The superficial cutaneous branches of the cervical plexus form the following nerves:
• Lesser occipital nerve. Formed from a branch of the C2 ventral ramus. It supplies the skin of the neck and scalp posterior to the ear.
• Greater auricular nerve. Formed from branches of the C2 and C3 ventral rami. It supplies skin around the ear and mastoid area.
• Transverse cervical nerve. Formed from branches of the C2 and C3 ventral rami. It supplies skin on the lateral and anterior part of the neck.
• Supraclavicular nerve. Formed from branches of the C3 and C4 ventral rami. It divides into three nerves (medial, intermedian and lateral) and supplies the skin over the sternocleidomastoid; the sternoclavicular joint, the upper chest wall,the acromion process and the upper and posterior parts of the shoulder.
Dorsal rami innervation
The dorsal rami of the upper three cervical spinal nerves innervate the: sub-occipital muscles, the posterior neck muscles, the skin over the cranio-cervical spine and the occiput, and the ligamentum nuchae (Bogduk, 2001) (Fig. 8.10). Branches of the dorsal rami form the following nerves:
• The sub-occipital nerve (C1). The dorsal ramus of the first cervical nerve is called the sub-occipital nerve. It exits the spinal cord between the occiput and the posterior arch of C1. It enters the sub-occipital triangle where it supplies the sub-occipital muscles (SOMs) (Bogduk, 2001; Middleditch & Oliver, 2005; Standring, 2008). (Any changes to the SOM can potentially affect the sub-occipital nerve giving rise to a peripheral neuropathy.)
• The greater occipital nerve (C2). The medial branch of the C2 dorsal ramus is called the greater occipital nerve (GON). It emerges between the atlas and axis, passes through the A-A joint capsule and ascends to innervate the skin over the posterior part of the scalp as far forward as the vertex of the skull (Middleditch & Oliver, 2005; Standring, 2008). It is closely related to the posterior aspect of the A-A joint and, as a result, is susceptible to any changes occurring at this joint. Owing to its large ROM, the A-A joint is particularly prone to osteoarthritic changes, such as osteophytic growth, which may irritate the GON and result in a peripheral neuropathy frequently referred to as greater occipital neuralgia (Comley, 2003; Ehni & Benner, 1984). Additionally, the C1–C2 level is commonly affected by rheumatoid arthritis and may become unstable as a result. In this situation the GON may be the source of occipital pain due to chemical irritation from the rheumatoid disease or to mechanical impingement secondary to increased upper cervical movement.
• The third occipital nerve. The dorsal ramus of C3 divides into several branches. The superficial medial branch is called the third occipital nerve. It passes posteriorly around the articular pillar of C3, innervates the C2–C3 facet joint and supplies the skin over the sub-occipital region (Fukui et al, 1996; Middleditch & Oliver, 2005). Fukui et al (1996) demonstrated that electrical stimulation of the C3 dorsal ramus produces pain over the occiput and upper posterior cervical regions. Close association of the third occipital nerve with the C2–C3 facet joint means that any dysfunction of this joint has the potential to irritate the nerve and generate pain within its cutaneous field of distribution. The third occipital nerve has also been implicated in cervicogenic headache (Bogduk & Marsland, 1985; Lord et al, 1994).
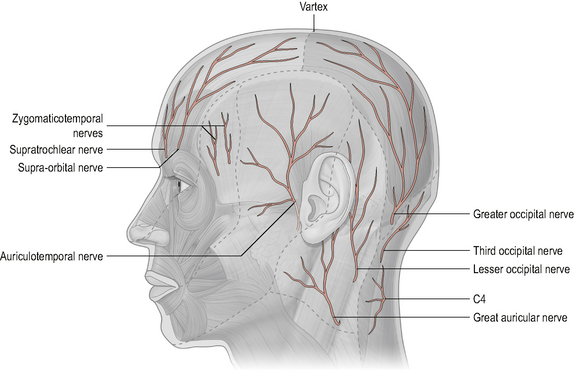
Figure 8.10 • Innervation of the scalp by the dorsal rami innervation of the upper three cervical spinal nerves.
(Reproduced from Drake et al (2005) with permission from Elsevier)
Knowledge of which structures are supplied by the ventral or dorsal rami is largely a means of being systematic and whilst it may be the case that structures innervated by the dorsal rami will refer pain differently to those structures innervated by the ventral rami, essentially what is of clinical importance is the segmental level of innervation of the structure e.g. C1/C2 or C3 and the region into which the structure can refer pain (Bogduk, 2008).
Pain pattern from upper cervical somatic structures
Few studies, however, have been carried out to map individual referral patterns from each somatic structure. Referral patterns from facet joints have been the most commonly studied. Irritation of the A-O and A-A facet joints by injection of contrast medium has demonstrated that these joints refer pain into the upper posterolateral cervical region 100% of the time and into the occipital region 30% of the time. Irritation of the C2/C3 facet joint has been shown to produce pain more or less equally in the upper posterior cervical region, upper posterolateral cervical region and in the occipital region (Dreyfuss et al, 1994; Fukui et al, 1996) (Fig. 8.11).
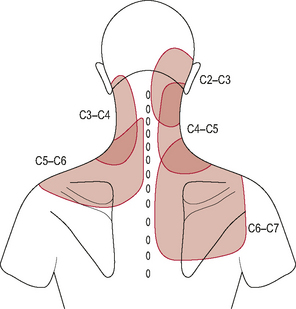
Figure 8.11 • Pattern of referred pain from the cervical facet joints.
(Reproduced from Bogduk (2002b), p. 68, Fig. 4.5, with permission from Elsevier)
Pain referral patterns of upper cervical ligaments have not been mapped out but recent MRI studies on patients following whiplash trauma have confirmed that these ligaments are an important causative factor in the neck pain associated with syndrome (Kaale et al, 2005).
Referred pain from upper cervical somatic structures
In addition to producing local occipital, suboccipital and upper posterolateral neck pain, somatic structures of the craniocervical spine have been shown to refer pain to the head and face (Bogduk, 2001).
Because the boundary of innervation of the upper cervical spinal nerves does not extend much beyond the vertex of the skull (see Fig. 8.12) pain felt in the forehead and facial region as a result of upper cervical dysfunction is not transmitted via the first three cervical nerves. Consequently, fundamental to the understanding of referral of craniocervical pain to the head and face is the overlap between trigeminal nerve afferents and upper cervical afferents through the trigeminocervical nucleus (TCN). This theory of somatic pain referral is referred to as the ‘convergence model’ and is the most likely explanation for pain referral from the neck to the head and face (Bogduk, 1992). The trigeminal nerve (fifth cranial nerve) is the main sensory nerve of the head and face; its sensory distribution includes the scalp, forehead, eyes, nose, cheeks, lips and teeth. It consists of three branches: the ophthalmic nerve; the maxillary nerve and the mandibular nerve which all converge on the TCN (Fig. 8.13). The TCN is a second-order neuron consisting of the spinal nucleus of the trigeminal nerve and the dorsal horns of the upper three cervical segments. It extends from the brain stem to the C3 level and receives afferent fibres from the trigeminal nerve and the upper three cervical spinal nerves. As a result of this connection, nociceptive information from the upper cervical segments may be perceived as originating from the trigeminal field of innervation, a phenomenon referred to as convergence or ‘crosstalk’. Clinically this means that, via the TCN, dysfunction in the upper cervical spine may be felt as head or face pain and therefore the cranio-cervical region should be considered as a potential source of symptoms in patients with these pain referral patterns (Bogduk, 2001; Busch & Wilson, 1989). The convergence model, however, may not fully explain somatic referred pain; other pain mechanisms such as central sensitization may also be involved although this has not yet been established (Jull et al, 2008).
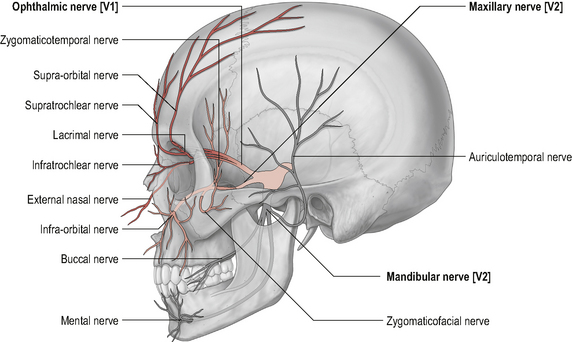
Figure 8.12 • Cutaneous distribution of the trigeminal and ventral and dorsal branches of the upper cervical nerves.
Upper cervical spinal nerve roots and peripheral nerves as pain generators
Mechanical irritation (compression or traction) or chemical irritation of the upper cervical spinal nerve roots can cause radicular pain. Radicular pain is often described as a sharp shooting pain characteristically referred into the area innervated by the particular nerve root (dermatome) and can be associated with diminished sensation into the same area. Upper cervical nerve root irritation can therefore generate referred pain into the C1–C3 dermatomal zones (Fig. 8.14).
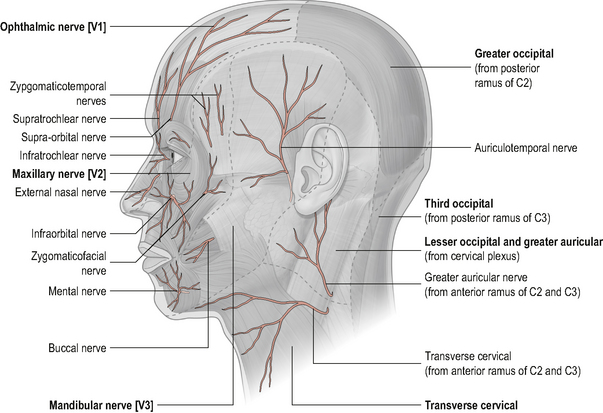
Figure 8.14 • Dermatomes of the head and neck.
(Reproduced from Drake et al (2005) with permission from Elsevier)
The first and second cervical spinal nerve roots, however, are unlikely to be affected by direct compression since they do not exit the spinal cord through intervertebral foramina and do not have any structural relations that make them susceptible to compression; nevertheless they may be vulnerable to other forms of irritation (Comley, 2003; Jull et al, 2008).
Irritation of peripheral nerves can give rise to pain and/or sensory impairment in the cutaneous distribution of the nerve (Greening & Lyn, 1998; Nee & Butler, 2006). The peripheral nerves, for example the sub-occipital nerve, lesser occipital nerve, greater occipital nerve and third occipital nerve, arise from the upper cervical nerve roots and supply the skin over the sub-occipital, occipital and posterior skull regions. As previously mentioned, it is possible that irritation of these nerves can give rise to a peripheral neuropathy and hence be a source of head and neck pain. Irritation of a peripheral nerve will give a different pain referral pattern than the dermatomal pattern associated with irritation of a nerve root. Knowledge of the cutaneous distribution of the upper cervical peripheral nerves as well as knowledge of the upper cervical dermatomes will help the manual therapist differentially diagnose the origin of cranio-cervical neurogenic pain (Figs 8.15 and 8.16).
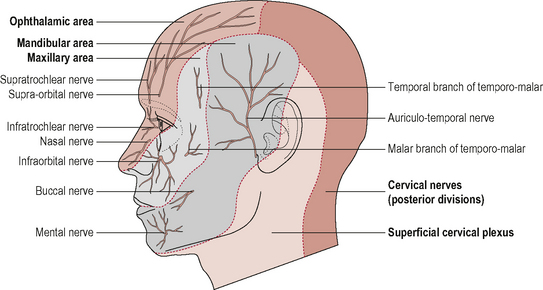
Figure 8.15 • Cutaneous distribution of the trigeminal and upper cervical nerves
(Reproduced from Drake et al (2005) with permission from Elsevier)
The vertebral artery as a pain generator
The vertebral artery is another structure that can potentially generate upper cervical pain. It is innervated by the vertebral nerve, which is sympathetic in nature. Of particular clinical interest is the connection between the vertebral nerve in the upper cervical spine and the ventral rami of the upper cervical nerves via the grey rami communicantes (Johnson, 2004). Clinically, this means that any nociceptive information from the vertebral artery can be relayed via the vertebral nerve and ventral rami to the dorsal root ganglia of C1–C3, thus the vertebral artery can potentially refer pain into the C1–C3 field of innervation.
Arterial dissection of the vertebral artery is rare; most lesions occur at the C1–C2 level as a result of the unique vascular anatomy at this point (Johnson, 2004; Kerry & Taylor, 2006). Due to the functional neural connectivity between the artery and the upper cervical spine, arterial dissections can present similarly to occipital headache. Presenting symptoms may be described as a sudden onset of severe, sharp pain localized to the ipsilateral cranio-cervical junction and occipital region. Thus in cases of acute onset of headache ‘unlike any other’, especially in those that precede trauma, the clinician must be suspicious of cervical vascular pathology and thus vertebral artery dissection must be included in the differential diagnosis (Kerry & Taylor, 2006).
SUMMARY
Innervation; pain generators and pain patterns of the cranio-cervical region
• Pain referring to the face and anterior aspect of the head from somatic structures of the upper cervical spine will involve the trigeminal nerve. It will not directly involve the upper cervical nerves as they do not innervate this area.
• Pain felt in the posterior scalp and neck may be referred from upper cervical somatic structures; it may be radicular pain from C1–C3 spinal nerve roots or it may be a peripheral neuropathy of peripheral branches from C1–C3 spinal nerves. It will not involve the trigeminal nerve since it does not innervate this area.
• Differential diagnosis of head, neck and facial pain will be facilitated by knowledge of the following patterns of pain referral:
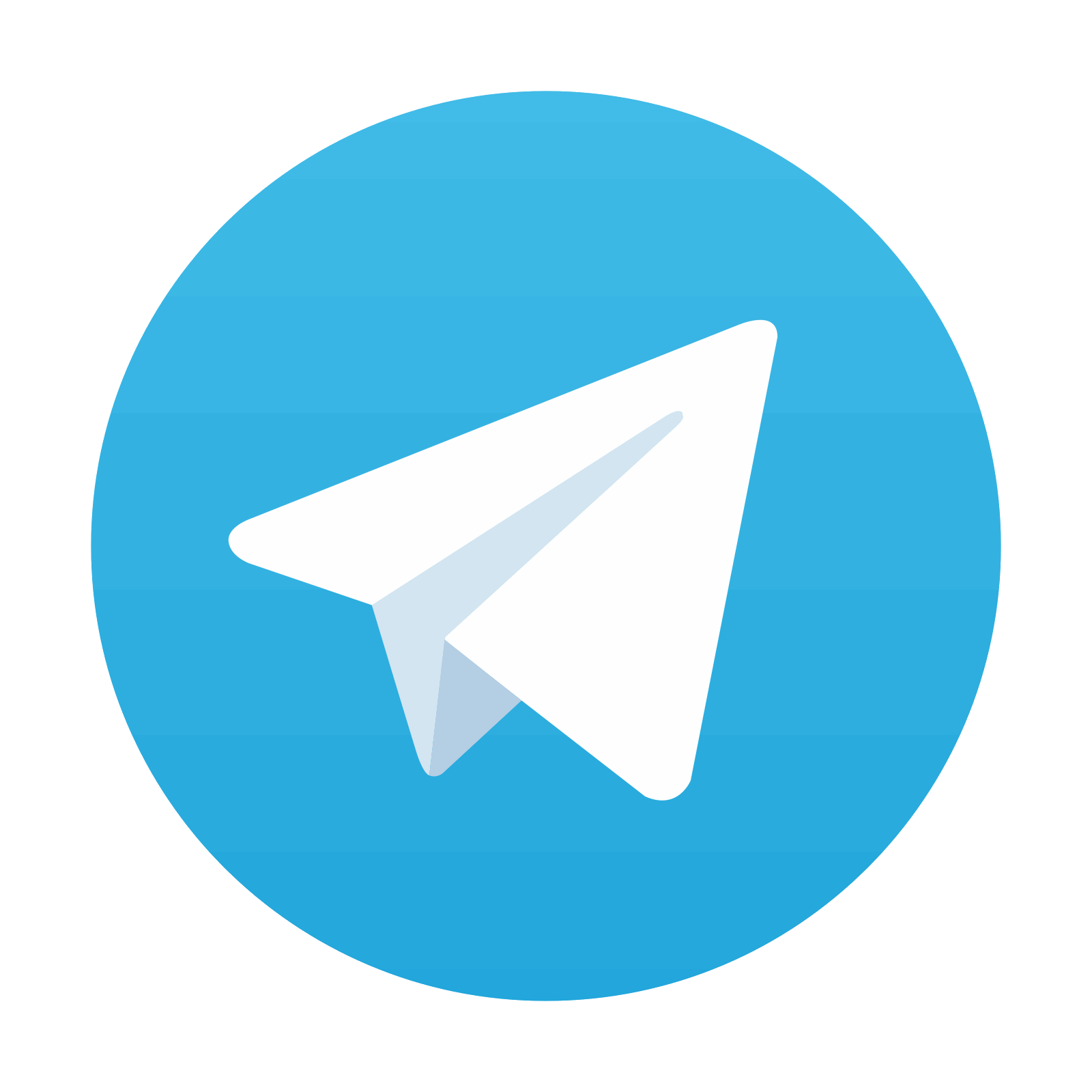
Stay updated, free articles. Join our Telegram channel

Full access? Get Clinical Tree
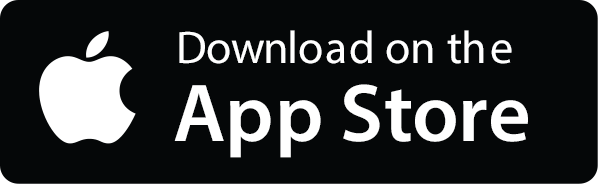
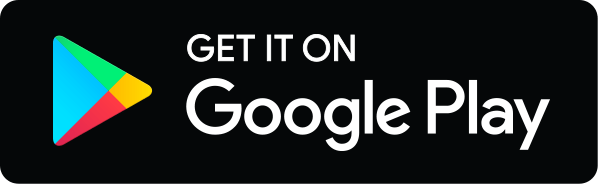
