Technique
Approximate costsa
Sites
Clinical/ research
Radiation dose (μSv)
Precision (CV %)
DXA (including iDXA)
$72–180 K
£39–99 K
€59–147 K
Lumbar spine
Both
0.4–4
<1
Total body
Both
0.02–5
1–2
Proximal femur
Lateral spineb
Both
0.15–5.4c12
0.15–5.4
NA
Axial QCT
Scanner (Software)
$630–900 K (18–22 K)
£345–493 K (10–12 K)
€516–736 (15–18 K)
Spine
Both
3D: 0.59–1.09 mSv
2D: 55 microSv
0.8–1.5 [126]
Femur
Research
3000 [127]
<1 [128]
Peripheral QCT
$76–251 K
£42 K
€37 K
Radius
Research
<1.5–4 per scan
0.8–1.5
Tibia
Research
3.6–7.8 3–5 year olds [61]
0.5–2.8 12 year olds [129]
Femur
Research
1.2–4 [130]
HRpQCT
$450–500 K
£250 K–280 K
€370–420
Radius
Research
<3 per scan
Not available
Tibia
Research
MRI
Scanner (Software)
$1.8–2.7 million (18–22 K)
£1–1.5 million (10–12 K)
€1.5–2.2 million (15–18 K)
Tibia
Humerus
Femur
Research
None
0.12–1.02 [97]
0.55–3.63
Table 2.2
An overview of radiation exposures for comparison with bone densitometry techniques
Effective dose (μSv) | |
---|---|
Return (round trip) transatlantic flight [131] | 80 μSv |
Annual naturally occurring background radiation [132] | |
North America | 3000 μSv |
UK | 2000 μSv |
Australia | 1500 μSv |
Hand radiograph [133] | 0.17 μSv |
Chest radiograph [134] | 12–20 μSv |
Lumbar spine radiograph [134] | 700 μSv |
Radioisotope bone scan [135] | 3000 μSv |
Table 2.3
An overview of reference data currently available with machines
Technique | Reference data | Source | N = | Age range (years) |
---|---|---|---|---|
DXA | Spine | 218c, 6661444 >1100 778 | 1–19, 8–17, 3–20 3 month – 19 2–20 | |
Proximal femur | 892, 1047 >1100 778 | 8–17, 5–20 4–27 2–20 | ||
Total body | 977, 1948 >1100 778 | 8–17, 3–20, 5–19 4–27 2–20 | ||
Forearm | ||||
QCT | Spine | |||
Radius | ||||
Tibia | • Stratec XCT-2000 [75] |
Table 2.4
A summary of what each technique ‘ameasures’ in relation to the bone’s biological organisation [2]
Method | BMDmaterial | BMDcompartment | BMDtotal | |
---|---|---|---|---|
Cortical | Trabecular | |||
DXA | No | Yes | No | Yes |
QCT central, peripheral | No | Yes | Yes | Yes |
HR pQCT | Yes (cortical) | Yes | Yes | Yes |
Table 2.5
A summary of the main advantages and limitations of each of the bone measurement techniques in children discussed in this chapter
Technique | Advantages | Limitations |
---|---|---|
DXA | 1. Speed 2. Cost 3. Precision 4. Availability of reference data 5. Low-radiation dose 6. Clinical applications established 7. Body composition measurement | 1. Size dependence 2. Sensitive to body composition changes 3. Software and reference data changes 4. Integral measurement of trabecular and cortical bone |
QCT | 1. Size independent 2. Separate measure of cortical and trabecular bone 3. Measurements of bone geometry 4. Imaging of trabecular bone structure feasible 5. Measurement of muscle and fat 6. Applicable to central and peripheral sites | 1. Radiation dose 2. Cost 3. Access for bone densitometry 4. Skilled staff 5. Specialist acquisition and analysis software limited 6. Limited reference data |
Peripheral QCT | 1. 1–5 as axial QCT above 2. Low-radiation dose 3. Lower cost than axial | 1. Scan time 2. Only applicable to peripheral sites 3. Difficulties in re-positioning in follow-up scans |
HR pQCT | 4. 1–4 as axial 5. Assessment and estimation of trabecular and cortical microstructure 6. In-vivo assessment of cortical BMDmaterial | 4. Cost 5. Susceptibility to movement artefact 6. Only applicable to peripheral sites |
MRI | 1. Non-ionising, non invasive 2. Size independent 3. Imaging in multiple planes 4. Applicable to axial and peripheral sites 5. Measurement of muscle and fat | 1. Noisy 2. Long scan time 3. Claustrophobia in ~ 10 % people 4. Parents cannot be in room with children |
What is Being Measured by Bone Densitometry?
Bone densitometry offers a tool with which pediatric bone status can be assessed. As the child grows, the skeleton will increase in size and mineral content, and bones will change in shape. When interpreting measurements from bone densitometry scanners, it is imperative that these changes in bone size, shape and mass are taken into account [1]. For example, changes in bone mineral density over time could reflect changes in bone size, mineral content or a combination of these. The quantitative measures that can be obtained from most two-dimensional densitometry techniques include bone area (BA, cm2), bone mineral content (BMC, g) and areal bone mineral density (BMD, g/cm2).
A model based upon the biological organisation of bone was proposed by Rauch to help in understanding and interpreting the measurements obtained from bone densitometry and relating these changes to the physiological changes that occur during bone development [2]. The model describes separate definitions for the ‘material’, ‘compartment’ and ‘total’ densities of bone (Fig. 2.1) and each of these will be discussed briefly:


Fig. 2.1
A model based on the biological organisation of bone proposed by Rauch and Schonau [2] to help in understanding and interpreting the measurements obtained from bone densitometry and to relate these changes to the physiological changes that occur during bone development. The model describes separate definitions for the material, compartment and total densities of bone
- 1.
Material mineral density: This reflects the degree of mineralization of the organic bone matrix. Material density can be determined only within a very small volume occupied only by bone matrix exclusive of marrow spaces, osteonal canals, lacunae and canaliculi. Until recently, the resolution required to measure BMDmaterial was not possible with current non-invasive densitometric techniques. The spatial resolution of high-resolution peripheral quantitative computed tomography makes possible the measurement of cortical BMDmaterial at distal, peripheral sites. BMDmaterial can be determined from specimens taken at bone biopsy, which is an invasive procedure. These specimens can be analysed by mineral/ash weight, contact radiography, backscatter electron microscopy or laser ablated mass spectrometry. Measurement of BMDmaterial is not routinely assessed in clinical practice.
- 2.
Compartment mineral density: The BMDcompartment is the amount of mineral contained within the trabecular, or the cortical, compartments i.e. the mass of mineral per unit volume of trabecular or cortical bone. Quantitative computed tomography (QCT) measures cortical and trabecular bone separately and can therefore measure BMDcompartment in both types of bone. DXA measurements are a composite of integral (trabecular and cortical) bone, and so the technique is not able to separate the two components at most sites. BMDcompartment can be determined by DXA in skeletal sites such as the diaphyses of the femur or radius which are comprised of cortical bone.
- 3.
Total mineral density: BMDtotal is the mineral density of all the material contained within the periosteal envelope and articular surfaces of a bone. QCT and DXA measure BMDtotal. Calculations are required to estimate bone volume from DXA scans since this technique measures areal density only. Bone mineral apparent density (BMAD) is an example of a volumetric density calculated using BMDtotal [3–5]. This density is sometimes inappropriately referred to in the literature as ‘true bone density’.
Table 2.4 summarises which of the aforementioned BMD measurements can be determined using the densitometry techniques discussed in this chapter; QUS and MRI do not measure BMD.
Dual Energy X-Ray Absorptiometry (DXA)
Dual energy X-ray absorptiometry (DXA) has been available since the late 1980’s and is now used extensively for diagnosis and monitoring of osteoporosis in adults (Tables 2.1 and 2.2) [6–8]. The fundamental principle of DXA is to measure the transmission of X-rays through the body at high and low energies. The use of two energies allows discrimination between soft tissue and bone; low-energy photons are attenuated by soft tissue and the high-energy photons by bone and soft tissue. By subtracting the soft tissue from soft tissue and bone, it is possible to quantify the amount of bone within the X-ray scan path. Pixel-by-pixel attenuation values are converted to ‘areal’ bone mineral density (aBMD [g/cm2]) by comparison to a bone mineral phantom. Bone area (BA [cm2]) is calculated by summing the pixels within the bone edges as defined by software algorithms . Bone mineral content (BMC [g]) is calculated by multiplying mean aBMD by BA. DXA may be applied to the whole body or skeletal regions of interest, for example the spine, proximal femur and radius.
One of the important technical developments in DXA over the past decade is the ability to image the whole spine for Vertebral Fracture Assessment (VFA) [9] at considerably lower radiation doses (40 microSv) than is the case with spinal radiographs (700–2000 microSv). Because of the high-radiation dose paediatricians were reluctant to perform spinal radiographs and so the prevalence of vertebral fractures in children with some chronic diseases was consequently underestimated. Recent studies confirm the prevalence of vertebral fractures in children with diseases treated with glucocorticoids ranged between 7 and 16 % [10–12]. DXA VFA is used increasingly in adults and children to identify vertebral fractures [13, 14] and so improve management of osteoporosis and reduce future fracture risk [15]. Both major DXA companies (Hologic Inc. Bedford MA and General Electric Healthcare: Lunar, Madison, WI) have improved image quality generally by the use of more sensitive detectors and small increases in radiation dose. Dual energy images give superior visualisation of vertebrae in the thoracic region. To acquire lateral VFA images the major DXA manufactures use different techniques : GE Lunar acquire single- (SE) and dual-energy (DE) images simultaneously and rapidly (less than 2 min) and Hologic acquire such images separately, with a rapid 10 s SE scan which is what is usually performed but the DE acquisition which is superior for visualisation of vertebrae in the thoracic spine may take up to 5–8 min depending on the size of the patient. The latter DE scan acquisition is impractical in children due to respiratory movement artefact. The GE-Lunar iDXA makes more feasible the assessment of lateral spine images for fracture identification in children (Fig. 2.2), which improves diagnosis in children with chronic conditions [11, 16] in which such fractures are more common than was thought previously [17, 18].


Fig. 2.2
Lateral DXA vertebral Fracture Assessment (VFA) image (dual energy) in a child of 16 years with osteogenesis imperfect obtained on a GE Lunar iDXA scanner . Vertebrae from T3 to L5 are visualised with vertebral fractures at T6 and L1. The vertebral endplates are dense as occurs with bisphosphonate therapy. The jagged edge to the field of view is due to the use of SmartScan which minimises ionising radiation dose as appropriate in children
DXA is the most widely available bone densitometry technique for measurement of bone status in children [19]. The advantages and limitations of the technique are discussed more extensively in subsequent chapters. The advantages of DXA include rapid scan times (less than a minute, low-ionising radiation dose and the availability of pediatric reference data). The cost of running a DXA service is also relatively inexpensive. DXA can be used to assess body composition and is currently the only technique that can be applied to the hip region in children.
Whilst DXA has many advantages, the limitations of the method must be considered. BMD measurements provided by DXA are size dependent, since they are based on two-dimensional projections of three-dimensional structures which do not adjust for the depth of the bone. As a consequence BMD in small children will be under-estimated and over-estimated in children with large bones. Growth between scans should be taken into account when interpreting longitudinal data. There are several methods to correct DXA data for size dependence [3, 4, 20–25] as discussed further in Chaps. 3 and 6. DXA measurements are also influenced by changes in body composition and due consideration must be given to such changes when interpreting data. Overall, DXA remains the primary bone densitometry tool for clinical pediatric bone assessments and an important research tool.
Quantitative Computed Tomography (QCT)
Central
Central quantitative computed tomography (QCT) of the spine was first described in the late 1970s [26], and became more widely used during the 1980s [27]. With the introduction of dual energy X-ray absorptiometry (DXA) in 1988 the use of QCT declined. However, there has been renewed interest in QCT as investigators recognise the importance of bone size and geometry in assessing pediatric bone status. QCT is particularly useful in children since it measures volumetric density (g/cm3), which is not as size dependent as areal density (g/cm2) measured by DXA. Recent technical developments (such as spiral and spiral multi-detector MDCT) add to the potential information available from QCT. While QCT for clinical and research purposes may increase in the future, it should be noted that the radiation dose from QCT is substantially higher than that of DXA, but lower than in CT of the torso used for other clinical indications [28–32].
QCT of the spine requires that the patient lie supine on the scanner table with the legs flexed and supported on a pad to flatten out the natural lumbar lordosis (Fig. 2.3a). The height of the scanner table should be kept constant. A bone mineral equivalent phantom is placed under the patient in the site to be scanned (Fig. 2.3b). A water or soft tissue equivalent pad should be placed between the patient and the phantom if there is a significant air gap (Fig. 2.3b). Solid hydroxyapatite calibration phantoms are currently used with regions of varying density that allow the transformation of measurements in Hounsfield units (HU) to bone mineral equivalents in mg/cm3. Some CT manufacturers provide their own software and phantoms (e.g. Siemens Healthcare, Erlangen, Germany); alternatively software and phantoms can be purchased (e.g. Mindways, Austin, Texas, USA). For comparable results in longitudinal studies the same phantom (and scanner) should be used. Similar to DXA instrumentation [33, 34] if scanners or phantoms have to be changed during longitudinal studies then cross-calibration with patients and a phantom, such as the European Spine Phantom (ESP) [35], must be undertaken to make results comparable, although the ionising radiation involved makes duplicate measurements in children problematic (Table 2.2 ).






Fig. 2.3
Central QCT: (a) Patient positioned for central QCT with knees slightly flexed over a moulded support to flatten lumbar lordosis . (b) cross section through mid-plane of lumbar vertebra with patient lying on the bone reference phantom from Mindways with cylinders of fat, water and 50, 100, and 200 mg of hydroxyapatite, and gel cushion between phantom and patient to reduce artefacts. The defect in the posterior cortical margin of the vertebral body is the entry of the basi-vertebral vein indicating the middle of the vertebral body. (c) 2D single slice technique: lateral scout projection radiograph (scout view) with the plane of the mid vertebral body sections of L1 to L4 indicated where volumetric BMD will be measured. (d) 3D volumetric technique: acquired with MDCT – midline sagittal (middle images) and coronal (lower images) reformats through L1 to L3 with mid-plane lines through each vertebra and oval region of interest of analysis placed in trabecular region to provide vBMD (upper images). (e) Lateral CT scout view: which should be scrutinised for vertebral fractures; grade 2 moderate vertebral fracture of T12 (arrow)
For two-dimensional (2D) spine measurements , an initial lateral scan projection radiograph is obtained (Fig. 2.3c). A 10 mm section is then performed through the mid plane of the vertebrae to be measured and parallel to the vertebral endplates. The section is confirmed to be in the correct plane when the area of the basi-vertebral vein is identified (Fig. 2.3b). For 2D QCT in adults, generally three vertebrae are scanned (L1 to L3) to ensure that at least 2–3 vertebrae are available for analysis at follow-up scans, should it be necessary to exclude vertebrae that have fractured between measurements. Since vertebral fractures occur less commonly in children and to minimise ionising radiation dose, generally only two adjacent vertebrae (between L1 to L3) are scanned. Vertebrae should be matched to those scanned in the reference database used, because BMD differs between vertebrae. If longitudinal studies are performed it is essential to scan the identical vertebrae examined at baseline.
QCT results are expressed as a mean volumetric bone mineral density (vBMD) in mg/cm3. The trabecular vBMD measured by QCT is a composite of the amount of bone and marrow per voxel. The reason for this is the relatively small size of trabeculae compared to the voxel, resulting in marrow being included in the measurement. Because marrow fat is limited in children, age related marrow changes in fat composition should not confound spinal vBMD measurements in children as significantly as they do in adults [36].
Over the past two decades advances in CT technology and the introduction of multi-detector spiral CT (MDCT) enable rapid scanning so that several vertebral bodies are imaged within less than a minute. This technology has been used for volumetric QCT, which provides 3-D volume analysis of the vertebral bodies (mostly L1-L3). These developments improve precision (coefficients of variation of better than 1 %) and have advantages in children by reducing movement artefacts. In 3D MDCT sagittal midline reformatted images are obtained to define the mid-plane section to be analysed (Fig. 2.3d). Then, on the selected mid-plane cross-sectional image an oval region of interest (ROI) is placed in the central trabecular bone of the vertebral body at the site of the entry posteriorly of the basi-vertebral vein. The ROI should be as large as possible but not include the cortical rim of bone. As quantitative skeletal assessment does not require the optimisation of image quality needed for conventional CT, a low-dose technique can be employed to minimise radiation dose [37, 38] (Table 2.2). The results are expressed as standard deviations (SD) from the mean for appropriate age-, race- and sex-matched reference data (Z-score) . The most frequently used normative data for spinal QCT were reported by Gilsanz and colleagues in 2009 [30, 31, 39, 40] (Table 2.3).
QCT offers several advantages as a densitometric technique . Whereas DXA measures integral (cortical and trabecular) BMD, QCT provides separate measures of cortical and trabecular BMD. As trabecular bone is generally more metabolically active than cortical bone, trabecular vBMD as measured by QCT is more sensitive to change in BMD [41]. The BMD provided is volumetric and less influenced by bone size than DXA, which provides an ‘areal’ density (g/cm2). CT also provides true morphometric dimensions of bones, and in the shafts can measure cross-sectional area of bone, cortical thickness and density, and periosteal and endosteal circumference. These parameters can be used to calculate estimates of biomechanical bone strength including moment of inertia. QCT also has the potential to be applied to peripheral skeletal sites such as radius, tibia and mid femur with lower associated radiation exposure than spinal QCT [30, 42, 43]. In addition QCT studies can be used to perform finite element analysis allowing the calculation of bone strength [44].
The limitations of QCT include the substantially greater dose of ionising radiation than DXA for spine scans (Table 2.2). For single slice (2D) QCT low-dose protocols using 80 kVp (or 120 kVp) and 120 mAs (or 150–200 mAs) result in effective doses of less than 200 μSv [32]. Felsenberg et al. described a low-energy, low-dose protocol with 80 kVp and 146 mAs resulting in effective doses down to 50–60 μSv, including the preliminary scan projection (scoutview) radiograph [45]. In comparison DXA has radiation doses in the range of 5–13 μSv for the lumbar spine and 9 μSv for the hip while an AP lumbar spine radiograph has a dose of 700 μSv and a standard abdominal CT has an exposure dose of approximately 8000 μSv [46]. For volumetric (3D) MDCT QCT radiation doses are higher and have been estimated to be as high as 1.5 mSv for the spine and 2.5–3 mSv for the hip [47]. Access to QCT may be problematic because many radiology departments lack the appropriate phantoms and software to perform bone densitometry studies. Furthermore, CT equipment is in great demand for other clinical diagnostic purposes. Currently there is a dearth of commercial analysis packages for QCT that can be purchased which require little set-up (for example Mindways, Austin, Texas, USA), Therefore, some centres have resorted to developing their own analysis software [48]. As with other bone densitometry techniques, QCT requires skilled and dedicated technical staff to perform the scans and optimise precision. Finally, there are far fewer published pediatric reference data for QCT than for DXA (Table 2.3) [30, 39].
More recently Habashy et al. investigated the feasibility and potential limitations of estimating BMD from standard diagnostic CT studies. They compared BMD measurements obtained with and without an external calibration phantom and found that phantomless QCT adds clinically useful BMD information to standard diagnostic CT studies [49]. The preliminary scout view should always be scrutinised for the presence of vertebral fractures (Fig. 2.3e).
In summary, and based on the general consensus of the ISCD QCT Task Force and working group [30]: the most common sites scanned on whole body CT scanners are the lumbar spine (L1 to L3), mid femoral shaft and the tibia. In the long bones, a metaphyseal and diaphyseal site should be scanned, and ideally in longitudinal studies the whole bone using 3D MDCT QCT should be scanned. This will enable results from similar anatomical sites to be compared which may be problematic in growing bones in 2D pQCT. Also, lying on the table of a general purpose scanner may afford easier positioning of children with disability (e.g. cerebral palsy, Duchenne muscular dystrophy) and rapid scanning (20 s) reduces movement artefact. The proximal femur (hip) should not be scanned as it is in adults as the ionising radiation dose is high (3 mSv). Currently, adult scan protocols are being used in children, and there is an urgent need for development of pediatric protocols to minimise radiation dose. More robust reference data and analysis tools are required for the wider applicability of the technique.
Peripheral Quantitative Computed Tomography (PQCT)
Peripheral quantitative computed tomography (pQCT) first became commercially available in the early 1990’s [50–52]. The most commonly used pQCT scanner (XCT 2000, Stratec, Pforzheim, Germany) utilises the original rotate-translate CT technology, which generates only single 2D slices (1–2 mm thick) and requires a long acquisition time of about 1 min to obtain a single slice.
The sites of measurement are the radius, tibia and femur. For clinical assessment of a child’s bone , the most commonly used site is the distal 4 % of the forearm or tibia length proximal to the distal growth plate. Peripheral QCT is most commonly applied to the non-dominant forearm . The forearm length is measured as the distance between the tip of the ulnar styloid and the olecranon . The forearm is placed pronated in the pQCT gantry with the elbow resting on a block and the hand gripping the hand fixture and the arm is secured with Velcro straps to prevent movement (Fig. 2.4a and b). In children it is important to avoid the section including the growth plate, which produces falsely high measures due to the zone of provisional calcification. To locate the appropriate scan slice, a scanogram is performed (Fig. 2.4c and d). A reference line is placed to bisect the medial border of the end of the distal radius in adults. In children if the growth plate is visible, the reference line is positioned to bisect the medial border of the distal dense metaphysis (Fig. 2.4c). If the distal radial growth plate has fused, the reference line is placed to bisect the medial border of the distal articular surface of the radius (Fig. 2.4d). For the tibia the reference line location varies but is usually placed on the metaphysis and again the scanner moves to the measurement site from this point (Fig. 2.5) . Upon closure of the growth plate at skeletal maturity the distal surface of the epiphysis joint margin is used for placement of the reference line. Radial abnormalities such as Madelung’s deformity (disturbance of growth of the medial component of the distal end of the radius which causes reduction in the carpal angle) may cause difficulties in positioning the reference line. In children treated with bisphosphonates, the reference line should be positioned to try to ensure that the growth arrest lines (residue of the provisional zone of calcification) are avoided in the measurement which will be artefactually elevated [53, 54].





Fig. 2.4
Peripheral QCT Radius: before scanning the forearm length is measured from ulna styloid to olecranon with arm flexed. (a) Child positioned in Stratec XCT 2000 pQCT scanner for scanning of non-dominant radius with forearm pronated in gantry and held securely with Velcro strap and (b) hand on moulded support. (c) Scout view: when the growth plate is unfused the reference line is placed to bisect the corner of the medial metaphysis of the distal radius (single line); the scan plane is then 4 % proximal to this reference line (double line). (d) Scout view: when the growth plates have fused in older children the reference line has to be placed to bisect the corner of the medial border of the distal articular surface of the radius. Cross-section at (e) 4 % distal radial site showing central trabecular bone and outer cortical bone (white) at which vBMD can be measured and (f) 50 % mid diaphyseal site at which bone geometry, density, strength and muscle area and ‘density’ can be measured

Fig. 2.5
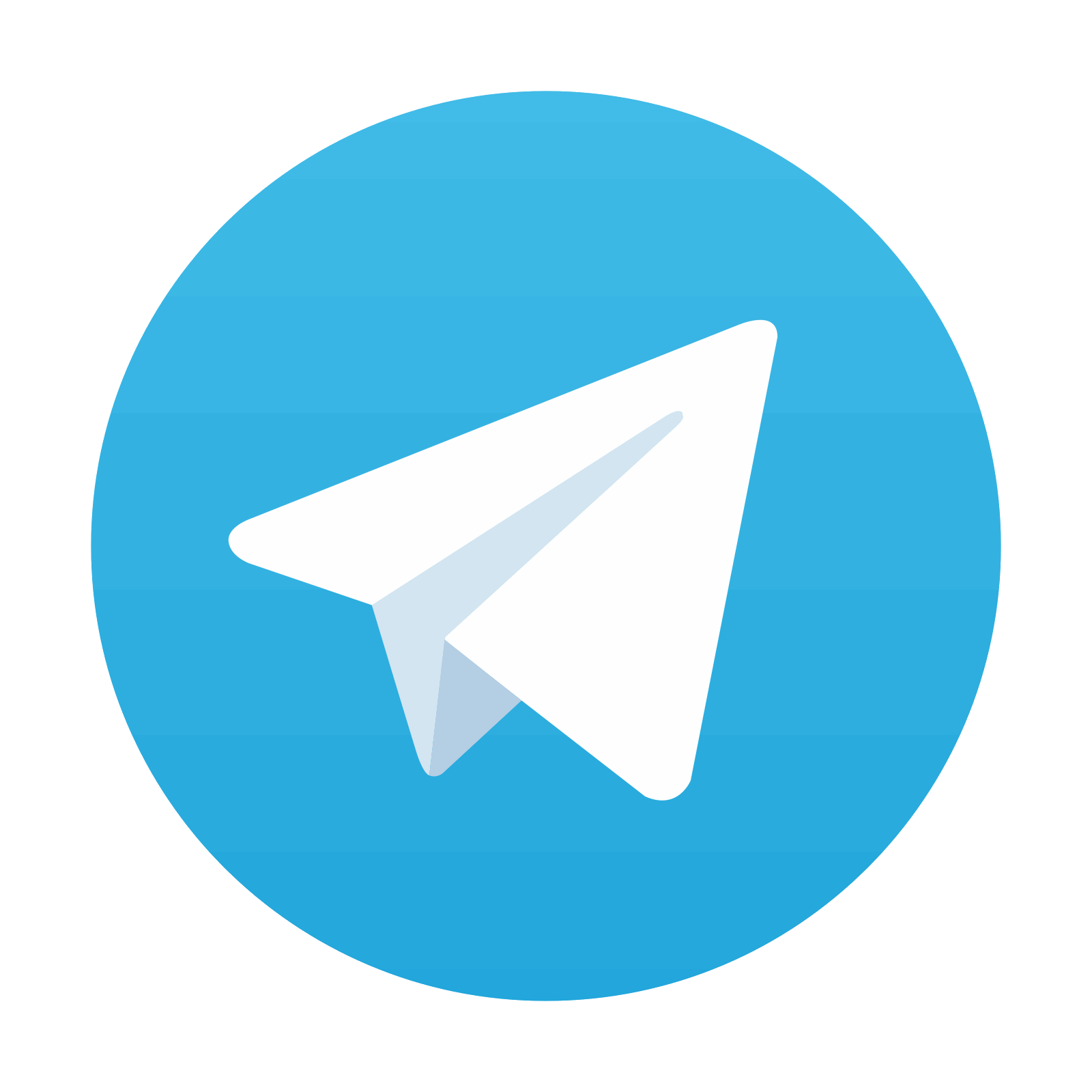
Peripheral QCT Tibia: (a) Scout view of distal tibia with unfused growth plate so the reference line is placed to bisect the lateral corner of the metaphysis of the distal tibia (single line); the scan plane is then 4 % proximal to this reference line (double line). (b) Section through the distal tibial shaft and (c) through the tibial diaphysis. At the distal site total and trabecular vBMD is measured. In the diaphysis bone geometry, density, strength and muscle area and ‘density’ can be measured
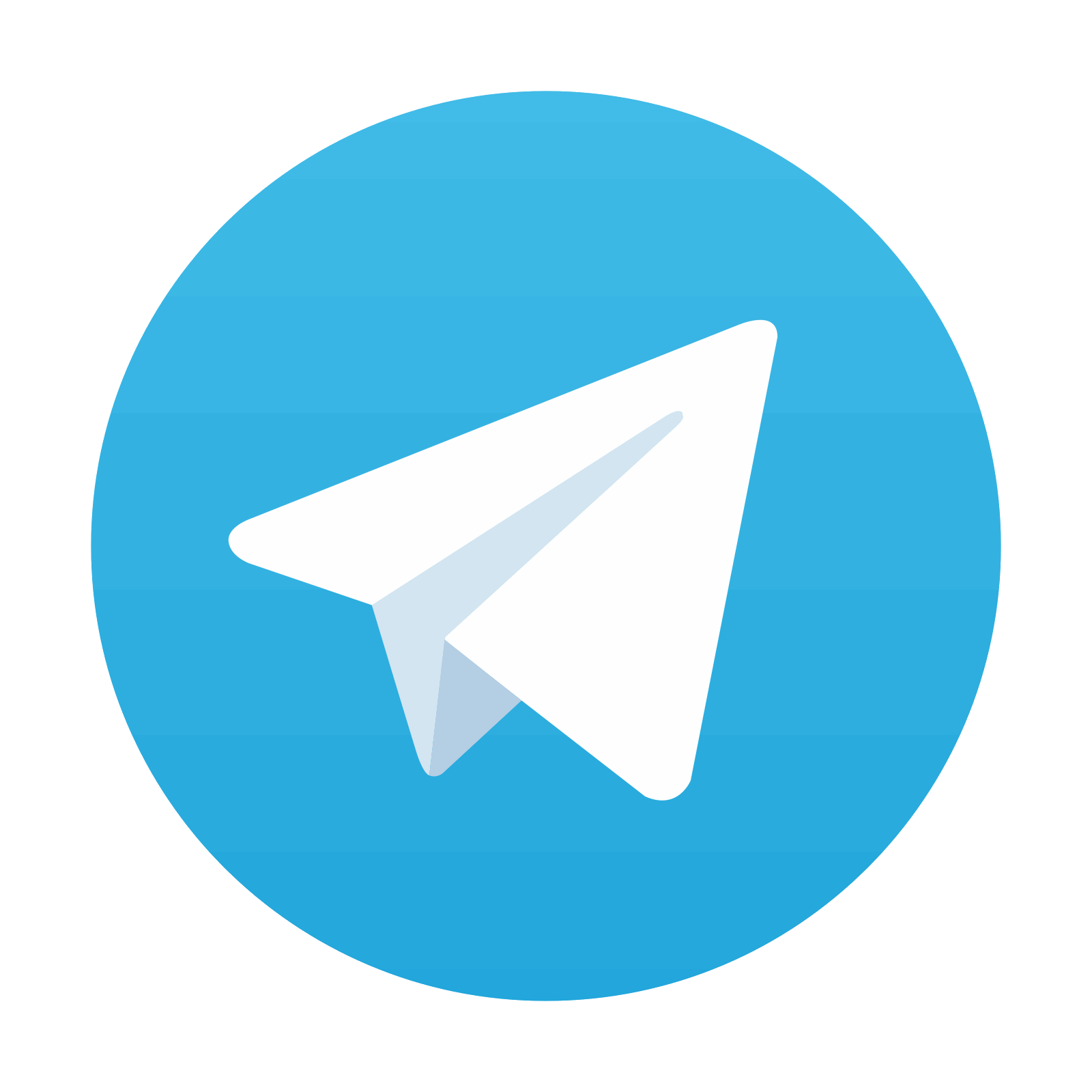
Stay updated, free articles. Join our Telegram channel

Full access? Get Clinical Tree
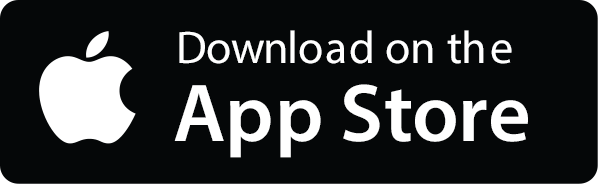
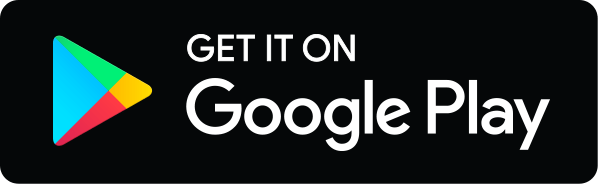
