Fig. 12.1
(a) Radiograph of an infant’s lower leg, showing metaphyseal fractures of the distal tibia (arrows). (b) Metaphyseal fracture of the left distal tibia in a 2-month-old (arrow)

Fig. 12.2
(a) Radiograph of an infant’s chest, showing posterior and lateral rib fractures (arrows). (b) Posterior 9th rib fracture in a 2-month-old (arrow)

Fig. 12.3
Transverse left femoral fracture in a 5-month-old
Other associations with nonaccidental trauma include children with head trauma, interhemispheric extra-axial hemorrhage, shear-type brain injury, and small bowel hematoma or laceration. These are often associated with shaking, direct head trauma, and trauma to the abdomen. The history cannot always corroborate the injury, so the provider must be aware. In children with unwitnessed head trauma, 22 % have been related to nonaccidental trauma with a skeletal survey [12]. A head CT is recommended in children less than 12 months old with a single, proximal, extremity fracture and a history of a nonaccidental trauma workup [13].
In older children, neurological and psychological conditions are often present in nonaccidental trauma victims [14]. This is a vulnerable population that often is non-ambulatory and any fracture should be concerning. Also, children with neurologic and psychiatric disorders can have metabolic bone diseases that predispose them to fractures. Poor nutrition, lack of sunlight exposure, medications, and lack of ambulation or physical activity decrease bone mineral density in this population [15]. Therefore, their fractures are multifactorial. Bone density decreases after immobilization and leads to an increased risk after surgical procedures to fractures in the immobilized extremity. Fractures in children with neuromuscular conditions are often spiral fractures from a twist or a fall out of their wheelchair. Concerning patterns are transverse fractures, metaphyseal fractures, and physeal separations as to the mechanism of the injury and the child’s inability to inflict the injury up on themselves.
Rickets secondary to vitamin D deficiency can predispose children to fractures. Radiographs may show widening of the physis, cupping of the metaphysis with diffuse osteopenia and bowing deformities of the lower extremities [16]. Children who are exclusively breastfed are pre-disposed to vitamin D deficiency [17]. While biochemical vitamin D deficiency may be found in children with multiple fractures, this deficiency is common in the pediatric population as well [18, 19]. Therefore, though biochemical vitamin D deficiency may be associated in a case of NAI, it should not presumed to be the cause of suspicious fractures in children, especially those that are non-ambulatory.
Osteogenesis Imperfecta
Osteogenesis imperfecta (OI) is a heterogeneous group of connective tissue disorders characterized be increased propensity to fractures throughout life. Other clinical features of OI include short stature, dentinogenesis imperfecta, fragile skin with increased tendency to bruising, a blue or gray scleral color, joint laxity, joint dislocations and pre-senile deafness. The clinical course of OI is extremely variable, ranging from stillbirth due to multiple intrauterine fractures to a lifelong absence of fractures. OI is the most common bone condition that has to be considered in the differential diagnosis of an infant with unexplained fractures.
In 1979, using clinical, radiographic and genetic criteria, Sillence and colleagues [20] classified OI into four major types (I–IV). However, the numbers of OI types have increased with the discovery of new genetic defects. Currently, 16 genes have been identified in the Online Medelian Inheritance in Man (OMIM) database. In 90 % of cases, OI is caused by heterozygous mutations in the COL1A1 and COL1A2 genes that encode for the pro-alpha-1(I) and pro-alpha-2(I) chains of type I collagen. These forms of OI are inherited as an autosomal- dominant trait; however, up to 25 % of children with OI have new mutations. Recurrence of OI without an affected parent may occur due to gonadal mosaicism mutations in one of the parents.
OI type I, (the mild nondeforming form with blue sclera) is the mildest form of the disease. The majority of patients with type1 OI have mutations in COL1A1 allele leading to approximately 50 % reduction or haploinsufficiency in the synthesis of type 1 procollagen. Fractures usually start during pre-school years, when the child becomes increasingly ambulant and tend to decrease after puberty. Intrauterine long-bone fractures, fractures before walking age, and skeletal deformities, such as bowing, are rare. The majority of OI types II to IV arise from mutations in COL1A1 and COL1A2 genes that lead to the production of structurally abnormal collagen. OI type II, (the perinatal lethal form), is the most severe form of the disease. Affected individuals have multiple intrauterine fractures with crumpled femora and beaded ribs. Most patients die in utero or shortly after birth due to respiratory failure. Patients with OI type III, (the severe deforming type), have limb deformities from numerous fractures occurring in utero and characteristic triangular facies and yellowish opalescent teeth (i.e., dentinogenesis imperfecta). Patients with OI type IV, (the moderately deforming type), usually have white sclerae, and most (but not all) patients have dentinogenis imperfecta. The severity of bone disease in subjects with OI type IV can be variable; some have fractures in utero leading to deformities, whereas others suffer only a few fractures throughout their life. OI type V accounts for around 5 % of cases and is inherited in an autosomal dominant manner. It is caused by heterozygous mutation in the gene, which encodes interferon-induced transmembrane protein 5 (IFITM5). These patients have distinctive features: hyperplastic callus formation, a dense metaphyseal band adjacent to the growth plate during infancy and progressive calcification of the interosseous membranes in forearms and legs. They are also prone to radial head dislocation. Patients with OI type 5 have white sclera and dentinogenesis imperfecta is not present.
Autosomal recessive forms of OI account for around 10 % of cases, and are caused by deficiencies in proteins responsible for posttranslational modification, folding and chaperoning of type I collagen. Mutations in some of these genes CRTAP, PPIB & LEPRE1, SERPINF1, SERPINH1, SP7, WNT1, PLS3, BMP1, PLOD2, CREB3L1, TMEM38B and FKBP10 account for some of the causes of OI type II to IV [21].
Radiographic features in milder types of OI may be nonspecific but include slender (gracile) bones with thin cortices and osteopenia. Wormian bones measuring 6 × 4 mm or larger in size and more than 10 in number around the lambdoid suture on skull radiographs are suggestive of OI. Rib and metaphyseal fractures, which are seen in infants with NAI, may also occur in OI, but are less common in those with milder forms of the disease (types I & IV).
In a child with unexplained fracture(s), the clinical diagnosis of OI is based on obtaining the relevant the family history (fragility fractures, joint dislocations, dentinogenesis imperfect), undertaking a clinical examination looking for the signs and symptoms of the disease (blue-gray sclera, characteristic triangular facies, dentinogenesis imperfect, limb deformities and joint hypermobility) and looking for characteristic radiographic features (Wormian bones in the skull vault, osteopenia and gracile looking long-bones). Unfortunately, bluish-gray sclerae are not uncommon in healthy infants and therefore this finding is not particularly useful in the first 6-months of life. Dentinogenesis imperfecta , which is more likely to affect primary than permanent dentition, is usually not present in a child with OI type I, and some forms of OI type IV. Joint hypermobility is not uncommon in otherwise healthy infants and toddlers. Wormian bones are only present in around 30 % of cases of OI type I [22]. Osteopenia and gracile appearance of long-bones, which are subjective radiographic features of OI, may not be evident on radiographs of children with milder forms of OI (types I & IV). In summary, the diagnosis of OI is relatively straightforward in a child with a positive family history who has typical clinical and radiological features of the disease. Milder forms of OI, especially type IV with normal sclera and absence of dentinogenesis imperfecta, can be difficult to diagnose clinically, especially if classical radiological features of the disease are absent and no other family members are affected. Unexplained fractures in infants with such forms of OI may be confused with NAI. In such cases, genetic testing should be considered. Marlow et al studied skin fibroblasts of 262 samples that had been submitted to their laboratory in whom NAI was suspected, but OI was also considered or could not be excluded [23]. Skin fibroblast cells in 11 (4.2 %) infants had abnormalities consistent with OI and in 11 others the diagnosis could not be excluded. Therefore, clinical evaluation did not identify some children with mild forms of OI. Genetic testing is justified in these children as failure to diagnose OI may lead to prolonged separation of the child from his/her parents, and in some case they may be put up for adoption. In such cases, Pepin & Byers recommend sequence of COL1A1A (OI type I or IV), COL1A2 (OI type IV), and IFITM5 (OI type V) genes simultaneously. If a causative mutation is not identified, and the infant with unexplained fractures does not have clinical features of OI, no additional gene testing is indicated. However, such analysis may uncover variants of unknown significance, which may or may not be pathogenic. If the variant is identified in one of the asymptomatic parents then it is likely to be nonpathogenic. If the variant segregates with a bone phenotype then it may be pathogenic and further studies, such as protein studies, may be required [24].
The Role of Bone Densitometry in the Assessment of an Infant with Multiple Unexplained Fractures
As mentioned previously, milder phenotypes of OI, especially type IV, may be difficult to differentiate from NAI. In cases where parents deny harming their child, it is not uncommon for the parents and their legal representatives to inquire as to whether measurement of bone mineral density will help in differentiating normal children who have been victims of NAI from those with milder forms of OI, as 30–40 % of bone mineral density must be lost in order to have radiographic changes [25].
DXA measurements of bone mineral density (BMD ) in older children and adults with OI have provided conflicting results. Paterson and Mole [26] found areal BMD (aBMD) to be within the reference range in most adults with type I or type IV OI. In contrast, others [27–30] reported that aBMD in children with OI was significantly lower than that of age-matched controls. In a cross-sectional study, Rauch et al found that after adjustment for age, sex, and height Z-scores, the mean LS aBMD Z-scores of children with OI type 1 with mean age was 7.5 years (confirmed by genetic testing) was −4.0 [31].
Lund et al. [32] measured whole body and lumbar spine bone area (BA), bone mineral content (BMC), and aBMD in 63 subjects with both mild and severe types of OI. Their study cohort included 24 children (17 males), aged 5–18 years, of whom 15 were classified as having OI type I or OI type IV. The authors used the approach of Mølgaard et al. [33] to determine whether (a) the subject’s height was appropriate for age (looking for “short bones”), (b) the bone area was appropriate for height (looking for “narrow bones”), and (c) the BMC was appropriate for bone area (looking for “light bones”). They compared these findings with quantitative and qualitative defects in type 1 collagen produced by subjects’ cultured skin fibroblasts. Mean aBMD for age in both children and adults was low in patients with OI type III or IV and/or a qualitative collagen defect. Reduced BMC for age in OI children was due to reduced height (short bones) and reduced BMC for BA (light bones). In contrast, their BA for height was normal (normal bone width). Forty percent of all subjects studied and 75 % of those with either OI type I, a quantitative collagen defect, or both had aBMD for age values within the reference range. The fact that OI subjects suffered recurrent fractures despite normal or only slightly low aBMD for age suggests that impaired skeletal mineralization alone was not the only cause of bone fragility. The authors concluded that DXA has limited value in the assessment of recurrent fractures because aBMD in OI can be normal and because there are few pediatric reference data for children under the age of 2 years, the period when fractures due to NAI are most prevalent.
Very few studies have measured BMD in young infants with the milder forms of OI (i.e., type I and type IV) that would be considered in the differential diagnosis of fractures due to NAI. One small study of 14 children with OI by Miller and Hangartner recommended that the investigation of the infant with unexplained fractures should include assessment of BMD by quantitative computed tomography (QCT) [34]. However, few centers have QCT scanners capable of measuring volumetric BMD in infants, and there is a paucity of age and gender reference data to allow calculation of Z-scores. Larger studies are needed to confirm the authors’ preliminary findings.
Bishop and colleagues [35] compared aBMD of the lumbar spine (LS) by DXA in infants with fractures due to OI and those whose fractures were thought to have occurred nonaccidentally. They found that LS aBMD of infants less than 6 months of age in both groups were within the reference range and were not significantly different. There was considerable overlap in BMD between subjects with and without OI who were up to 2 years of age. In follow-up examinations, however, the increment in LS aBMD in OI infants was significantly lower (27/cm2/year) than that of non-OI infants (115/cm2/year). The authors concluded that a single BMD measurement was not helpful in differentiating between infants whose fractures resulted from OI versus NAI. Longitudinal aBMD measurements, however, may be helpful in discriminating between normal infants and those with milder OI phenotypes.
In summary, for the majority of infants with unexplained fractures, the diagnosis of NAI or OI can be reached with a detailed clinical history, a thorough clinical examination by a clinician experienced in bone disorders, and a skeletal survey interpreted by an experienced pediatric radiologist. DXA measurements do not help to distinguish healthy infants who have been victims of abuse from those with milder types of OI. Thus, in an infant with unexplained fractures, measurement of BMD cannot be used to diagnosed or exclude OI. Since up to 5 % of children evaluated for NAI may have OI, genetic testing may be justified to exclude OI in infants with unexplained fractures [24].
Fractures in Children with Disabilities
Postnatal skeletal development is modulated by the mechanical forces to which it is subjected to, which primarily arise from muscle contraction. The skeleton of a healthy growing child continuously adapts to increasing mechanical loading from bigger and stronger muscles by increasing bone mass and altering bone geometry. By contrast, non-ambulant children such as those with cerebral palsy, muscle weakness, spasticity, and lack of normal load bearing activities result in reduced periosteal bone expansion. This leads to development of slender long bones of abnormal shape, which have increased propensity to fracture due to altered mechanics. In muscle disorders such as Duchenne muscular dystrophy, progressive reduction in the mechanical loading and exposure to glucocorticoid therapy result in accelerated bone loss, as well as failure to accrue bone mass. Concern for skeletal fragility resulting from muscle disuse in children and adolescents often leads to investigations of skeletal status. Cognitive impairment, spastic movements, inability to lie still and seizures pose unique challenges for obtaining accurate densitometry measurements.
Cerebral Palsy
Cerebral palsy (CP) is a term used to describe a group of non-progressive disorders of movement and posture, resulting from an insult to the developing brain. It is one of the commonest chronic disabling childhood conditions, which is often associated with other comorbidities : cognitive impairment, behavioral problems, hearing and visual problems, feeding difficulties, poor growth, recurrent respiratory infections and epilepsy. Secondary musculoskeletal problems include joint contractures, kyphoscoliosis, hip subluxation, long bone deformity, and propensity to low trauma fractures of limb bones. Fractures in children and adolescents with CP tend to be more common in lower limb bones, especially around the knee [36–38]. They are more common in non-ambulant children and adolescents, who have severe forms of CP defined as level IV or V according to the Gross Motor Function Classification System (GMFCS) [39]. Such fractures often occur during normal activities such as dressing, handling and transferring. However, the cause of fracture may not be clear; the lack of a clear history of the injury causing the fracture due to difficulties in communication and delay in seeking medical attention for a fracture sometimes leads to suspicion of child abuse [40]. The prevalence of fragility fractures in non-ambulant children and adolescents with CP has been reported to be between 6 and 12 % [37, 38], and an annual incidence of 4 % [41].
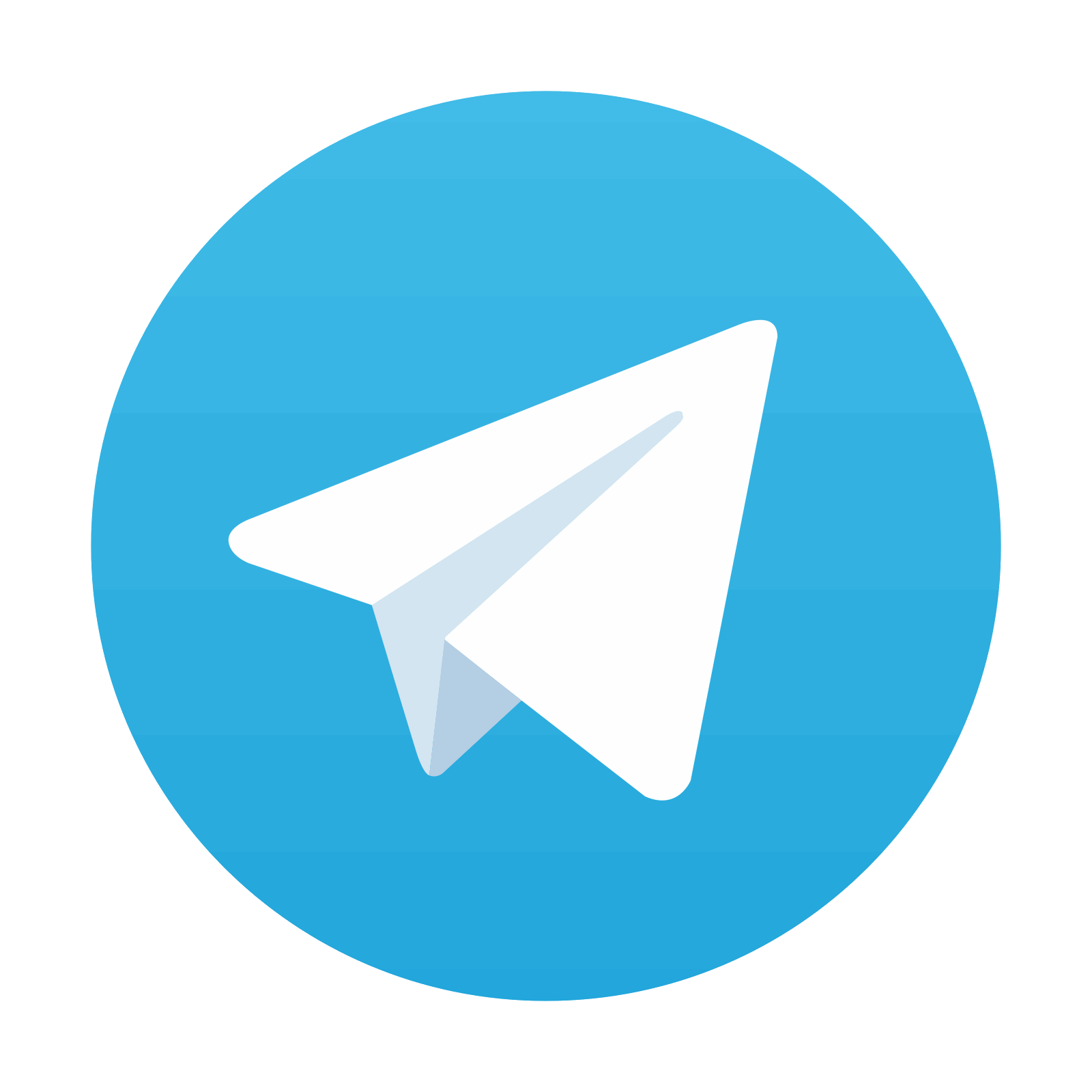
Stay updated, free articles. Join our Telegram channel

Full access? Get Clinical Tree
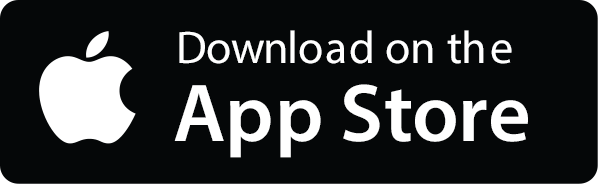
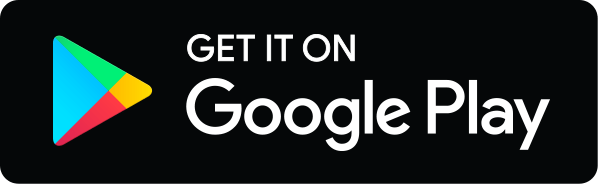