Calcium
Vitamin Da
Age
RDAb (mg/d)
ULc (mg/d)
RDAb (IU/d)
ULc (IU/d)
0–6 months
(200)d
1000
(400)d
1000
6–12 months
(260)d
1500
(400)d
1500
1–3 years
700
2500
600
2500
4–8 years
1000
2500
600
3000
9–13 years
1300
3000
600
4000
14–18 years
1300
3000
600
4000
19–30 years
1000
2500
600
4000
Females—Pregnancy and Lactation
14–18 years
1300
3000
600
4000
19–30 years
1000
2500
600
4000
Despite persistent controversies about the optimal calcium intake, it is evident that calcium intake before and during puberty can contribute to the maximization of PBM within an individual’s genetically determined potential. Several observational studies of children and adolescents in different countries have demonstrated an association between habitual calcium intake and BMC and/or BMD [48, 50–53]. A large, retrospective study of older, white American women also found that a higher milk intake during childhood and adolescence was associated with higher BMC and reduced fracture risk in adulthood [54]. Contrary to previous concerns, a calcium-rich diet in childhood has been linked to a reduced rather than increased mortality in adulthood from stroke [55].
The positive effects of calcium on height and BMC/BMD have also been supported by findings from several prospective randomized placebo-controlled trials [9, 56–58]. The skeletal effects have varied with the amount and source of calcium supplement, the skeletal region, and the age and maturity of the child [48, 57, 58]. Gains are greater at sites rich in cortical (appendicular skeleton) rather than trabecular bone (spine) [48].
Two meta-analyses have similarly confirmed the benefits of calcium supplementation and dairy products on bone mass during growth [59, 60]. The first one summarized 21 randomized controlled trials including 3821 subjects (aged 4–17.3 years) and found that greater calcium intake, with or without vitamin D, significantly increased total body and lumbar spine BMC in children with low intake at baseline [59]. The second one analyzed 19 randomized controlled trials including 2859 subjects (aged 3–18 years) and reported a small, positive effect of calcium supplementation (300–1200 mg/day) for total body BMC and upper limb BMD [60].
Whether the benefits from calcium supplementation are sustained after discontinuation is also controversial. Some studies have detected benefits for one or more years [9, 61] while other data suggest that the effects are lost soon after discontinuation [48]. A meta-analysis of several studies found that benefits of calcium supplementation persisted only at the upper limb [60].
Phosphorus
Despite the fact that phosphate makes up at least half of bone mineral mass, there is less concern about this nutrient in pediatrics. Phosphorus deficiency is rare because the element is abundant in common foods. In fact, concerns have been raised about overconsumption of phosphorus especially from soft drink consumption. Wyshak et al. found that the incidence of fractures in adolescent girls was correlated with the amount of carbonated beverages consumed [62]. The association between soft drinks and poor bone health is perhaps more likely explained by displacement of milk from diet than by high phosphorus intake [63]. A meta-analysis of 88 studies found an inverse relationship between soda consumption and intake of milk [64].
Vitamin D
Vitamin D is essential for efficient absorption of calcium. Only 10–15 % of dietary calcium is absorbed without vitamin D [65]. With few exceptions (oily fish), natural foods are not a significant source of vitamin D2 (ergocalciferol) or D3 (cholecalciferol). In some countries milk and other foods are fortified with vitamin D while in others, only infants and small children are routinely provided with supplemented products.
The essential role of vitamin D for bone health has been demonstrated by several studies. A longitudinal study of 198 children observed that when mothers had low levels of 25-hydroxyvitamin D (25OHD) during the late months of pregnancy, the children had low total-body and spine BMC at 9 years of age [42]. A 3-year longitudinal study of 171 healthy Finnish girls aged 9–15 years found that girls with severe vitamin D deficiency during puberty may fail to achieve their genetic potential for PBM, particularly at lumbar spine [66].
Vitamin D deficiency is relatively common, particularly in northern countries, in dark-skinned individuals, and in those with inadequate exposure to sunlight. Levels of 25OHD below 30 nmol/L [12 ng/ml] have been observed during winter and spring in up to 50 % of children living in Denmark, Finland, Poland, Greece, Germany, and Switzerland [66–71]. Vitamin D deficiency is more common in black and Hispanic teenagers, and in winter [65–74]. Obese children and adolescents are also at increased risk, possibly due to vitamin D sequestration in body fat [74–76]. Milder forms of vitamin D deficiency are typically asymptomatic but may still compromise optimal bone growth and mineralization. Vitamin D deficiency, not sufficiently severe to cause rickets, may also lead to secondary hyperparathyroidism.
Severe vitamin D deficiency (serum 25OHD below 15 nmol/L [6 ng/ml]) causes nutritional rickets in children and osteomalacia in adults. Low intestinal calcium absorption and secondary hyperparathyroidism lead to defective mineralization of growth plates and bones, with bone deformities and high risk of fractures. Fortification of infant foods with vitamin D has greatly reduced the incidence of rickets during the first 2 years of life in developed countries [17] but this condition remains a major health problem where vitamin-D–fortified foods are not available. Severe vitamin D deficiency is also associated with reduced bone mass in adolescents [73, 77]. A prospective study of 6712 physically active girls (age 9–15 years) found that greater intake of vitamin D (not calcium or dairy foods) during childhood was associated with reduced risk of stress fractures [78].
Protein
Adequate protein intake is necessary to build the bone matrix. Proteins also influence the secretion and action of insulin-like growth factor 1 (IGF-1), an osteogenic hormone needed to achieve optimal PBM. Inadequate protein intake adversely affects bone mass acquisition [79, 80]. A study by Chevalley et al. showed a positive correlation between protein intake and both BMC and BMD in pre-pubertal boys. With high protein intake, greater physical activity was associated with greater BMC at both axial and appendicular sites [81]. On the contrary, children with inadequate protein and caloric intake exhibited growth retardation and decreased formation of cortical bone [82].
The optimal type and quantity of protein for bone health remain to be determined [83, 84]. Milk and dairy products are probably the best sources of the calcium and proteins for bone health. Alternative sources of calcium include some vegetables, tofu, and almonds [85]. Long-term milk avoidance is associated with shorter height and lower BMC and aBMD [86–88]. Pre-pubertal children with low milk intake may be at greater risk of fractures, mainly of the distal radius [89, 90]. A 7-year study found regular intake of dairy products to be positively associated with hip and spine aBMD and greater total and cortical area at the proximal radius [91].
Exercise
The skeleton and muscles are interrelated in a more complex way than simple locomotion. According to the “mechanostat” model of bone growth and bone loss, muscle activity and weight load (gravity) continuously apply forces to the skeleton [92, 93]. The resulting strains stimulate bone modeling and remodeling. Throughout life, the bone’s cellular and biochemical reaction to mechanical strains translates into a continuous adaptation in terms of both bone mass and bone architecture that maintains and optimizes bone strength . Osteocytes imbedded in bone act as mechanosensors, transmitting signals to osteoblasts to build bone [94, 95]. Not surprisingly, muscle mass and strength are important predictors of bone strength [96–99] and conversely, prolonged immobilization and skeletal unloading lead to bone loss [100].
Regular physical activity is a major determinant of the accrual and maintenance of PBM. The type, intensity, frequency, and duration of exercise are all important. Dynamic loading seems more effective than static loading and the magnitude of the strain on bone may be more important than the number of repetitions [101]. Two observational studies of adolescent gymnasts found they had sustained increases in both BMC and aBMD [102, 103]. Several randomized controlled studies in children and adolescents reported positive effects from jumping and other high-impact activities [102–111]. Gains in hip BMC were 3.6 % greater in prepubertal children who completed a 7-month high-impact jumping program than in controls who completed non-impact stretching activities. Significant differences between the groups persisted even after 8 years (1.4 %, p < 0.05) [106]. A review of 22 intervention trials in children and adolescents concluded that physical activity had significant positive effects on bone, and weight-bearing exercise may enhance bone mineral gain in children, particularly during early puberty [112].
The sustained benefits of activity are mediated at least in part through changes in bone geometry. A systematic review of 14 intervention and 23 observational studies evaluated the effect of physical activity on bone structure (cross-sectional area, cortical thickness) as well as mass. Results indicated that changes in bone structure rather than bone mass were most often related to significant increase in bone strength. Prepuberty and peripuberty may be the best periods for improving bone strength through physical activity in both sexes [113].
There appear to be additive or even synergistic effects from various lifestyle factors . For example, one study found that physical activity enhanced the response to calcium supplementation at weight-bearing sites [107]. Conversely, gains from physical activity may be blunted in individuals who have inadequate intake of calcium or calories [114].
Importance of Peak Bone Mass
PBM is recognized as a key determinant of bone health and fracture risk in adulthood and old age. After early adulthood, BMC and BMD remain stable and then inevitably decline with menopause and aging. With enough bone loss, a “bone fragility” threshold is reached where fractures are more likely. Factors that enhance early bone accrual or slow the subsequent bone loss may help reduce the risk of osteoporosis (Fig. 1.1).


Fig. 1.1
Diagrammatic representation of the bone mass life-line in individuals who achieve their full genetic potential for skeletal mass and in those who do not. (The magnitude of the difference between the curves is not intended to be to scale.) Along the bottom of the graph are arrayed several of the factors known to be of particular importance. (© Robert P. Heaney 1999, used with permission.) From: Heaney RP, Abrams S, Dawson-Hughes B, Looker A, Marcus R, Matkovic V, Weaver C. Peak bone mass. Osteoporosis Int 2000;11:985–1009
In older adults, the risk of fracture doubles for each standard deviation (SD) that BMD falls below the healthy young adult mean value. An intervention that results in a 10 % increase in PBM in youth (+1 SD in BMD) could thus reduce an individual’s future fracture risk by 50 % [18, 23]. The magnitude of benefit from increasing PBM bone or reducing subsequent bone loss has been modeled [115]: a 10 % increase in PBM would delay by 13 years the time a woman meets criteria for osteoporosis (a BMD value of 2.5 SD or more below the young adult mean). By contrast, postponing the age at menopause or reducing the rate of age-related bone loss would delay osteoporosis by less than 2 years. In summary, optimization of nutrition and activity during childhood and adolescence can be viewed as an important and effective strategy to prevent or delay osteoporosis.
Threats to Pediatric Bone Health
The expected gains in bone mass and geometry described above can be compromised by a number of heritable or acquired disorders as discussed in more detail in Chap. 4 [17, 116, 117]. Osteogenesis imperfecta (OI) , the most common of the genetic disorders of bone, is characterized by increased bone fragility due to reduction in the quantity or quality of type 1 collagen caused by mutations in the COL1A1 and COL1A2 genes [118]. Loss of function mutations in the low-density lipoprotein receptor-related protein 5 (LRP5) gene result in reduced bone formation and low bone mass (as seen in osteoporosis-pseudoglioma syndrome ) [119]. High bone mineral density syndromes including osteopetrosis and pychnodysostosis are also associated with greater bone fragility [120].
The diverse causes of secondary osteoporosis share one or more skeletal risk factors [116, 117]. For example, Crohn disease [121, 122] and the rheumatologic disorders [123] are marked by chronic inflammation, undernutrition, reduced mobility, and exposure to osteotoxic drugs. In cerebral palsy and Duchenne muscular dystrophy (DMD), reduced mobility results in narrower long bones with thinner cortices that are more vulnerable to fracture [124, 125]. In DMD, glucocorticoid therapy and delayed puberty increase the risk of vertebral fracture. Children with malignancy [126, 127] or undergoing transplantation [127, 128] are vulnerable to fracture during treatment with chemotherapy, radiation, or immunosuppression; hormone deficiencies may follow. Finally, the improved survival in patients with cystic fibrosis [129] and thalassemia [130] has been complicated by diabetes, hypogonadism, undernutrition, and reduced exercise capacity. Depending upon age at onset and disease severity, these chronic conditions can impair bone growth and mineral acquisition with or without secondary bone loss. The potential for recovery from these skeletal complications depends upon the course of the acquired disease and the age of the patient.
Even apparently healthy children and teens with a history of low-trauma forearm fractures appear to be at increased risk for future bone fragility. When compared with children without a history of forearm fracture, children who fracture have lower bone mass, increased body fat, and less physical activity [131]. They have been shown to have a greater incidence of future fracture not only during childhood [131] but as adults as well [132].
Bone densitometry is an important tool to monitor the skeletal effects of these genetic and acquired disorders. Specific indications for ordering a bone density study for patients with these conditions are discussed in detail in Chap. 4. The International Society of Clinical Densitometry (ISCD) Position Development Conference (PDC) , a working group of pediatric bone experts, generated comprehensive guidelines for DXA use in 2007 [133]. These guidelines were revised in 2013 to recommend that densitometry be considered when the patient might benefit from intervention and when the densitometry results would influence management [134].
Densitometry as a Diagnostic Tool
Bone densitometry was developed as a noninvasive means to assess skeletal status and aid in identifying patients at greatest risk for fracture, ideally before fractures occur. In older adults, densitometry has proven useful in predicting fracture risk, thus guiding clinical management. In fact, low BMD is sufficiently linked to the likelihood of fracture in post-menopausal women that it can be used as part of the diagnostic criteria for osteoporosis . Older patients with a BMD that is equal to or more than 2.5 SD below the young adult mean (T score of −2.5) are diagnosed with “osteoporosis.” Densitometry results have been combined with clinical variables (including age, height, weight, prior fractures, glucocorticoid use, smoking, alcohol intake, and others) to create a Fracture Risk Assessment Tool (FRAX) . FRAX may provide a more precise estimate of the risk that an adult patient will have a hip or other fracture in the next 10 years than DXA alone [135].
Accurately identifying pediatric patients at greatest risk for fracture is especially important because treatment options are limited for younger individuals [116]. However, the interpretation of DXA data is more difficult and its role in fracture prediction less certain than in adults. In part this reflects the challenges of measuring bones that are changing in size, shape, and mass throughout the first two decades of life. The tempo of skeletal development varies among individuals, depending on pubertal development and skeletal maturation, and can be altered by illness. BMD measurements by DXA are 2-dimensional (BMC/bone projection area) and the results are influenced by bone size : this means that, in the presence of equivalent “volumetric” BMD (BMC/bone volume), children with smaller bones will apparently have lower BMD by DXA than children with larger bones [134]. Therefore it is important to account not only for sex, age, and ethnicity, but also for pubertal development, skeletal maturation, and bone size when interpreting BMD, particularly if growth and puberty have been altered by chronic disease. This may include adjustment for height Z-score or for skeletal maturation (bone age) as discussed in more detail in the Chaps. 6 and 7.
The association between bone densitometry and fracture risk in pediatrics is less well established than in adults. Furthermore, there is no established FRAX tool for younger patients to consider the contribution of clinical risk factors. The 2013 ISCD PDC guidelines included a comprehensive review of the current literature linking bone densitometry to pediatric fractures [136]. Most studies to date have explored which DXA parameter(s) best correlated with fractures after low- or moderate-trauma in healthy youth, because fractures are common; 50 % of boys and 30 % of girls will sustain at least one broken bone during childhood and adolescence, most commonly in the upper limb [137]. These studies found that low whole-body BMC or BMD corrected for bone size as well as low bone area for body size, were most strongly correlated with fracture risk [138]. However, even when the best estimates of skeletal mass and size from DXA and pQCT were combined, the predictive value for fractures was limited. The area under the receiver operator curve linking fracture to various densitometry measures ranged from 0.56 to 0.59, distinguishing those with forearm fractures from controls without fractures little better than chance alone [139]. A study using high-resolution pQCT detected alterations in bone microarchitecture associated with an increased risk of low-trauma forearm fractures, suggesting that this newer 3-dimensional methodology may offer more insights into fracture risk [140].
Extrapolating from observations made in healthy youth may not be appropriate for those with chronic illness. Unlike healthy youth who are most likely to sustain upper extremity fractures, children with immobilization disorders such as cerebral palsy or DMD more commonly fracture the lower extremity [141]. For these patients, BMD measured at distal lateral femur is more predictive of fracture than spine BMD. By contrast, younger patients with acute lymphoblastic leukemia (ALL) face a greater risk of vertebral compression fractures. One study found that 16 % of patients with ALL had at least one vertebral fracture at diagnosis [142] and an additional 16 % sustained an incident spine fracture during the first year of chemotherapy [143]. Spine BMD was highly correlated with fracture risk with an 80 % increased odds of vertebral fracture for every SD that spine BMD fell below expected mean for age [143].
After considering the limitations of densitometry to predict pediatric fractures, the 2007 PDC guidelines concluded that “the diagnosis of osteoporosis in children and adolescents should not be made on the basis of densitometry criteria alone” [133]. In 2013, criteria for the diagnosis of osteoporosis were expanded to include a child or teen who sustains one or more vertebral compression fractures without local bone disease or high-energy trauma [134]. Measuring BMD in these patients can add to the assessment but is not required. Alternatively, osteoporosis can be diagnosed in patients with a combination of low bone mass (BMC or BMD more than 2 SD below the mean for age) and a significant fracture history (two or more long bone fractures by age 10 years, or three or more long bone fractures before age 19).
Although a single BMD measurement cannot be used to diagnose osteoporosis in children, densitometry is considered a valuable part of a comprehensive skeletal health assessment. Such an evaluation includes a review of prior chronic illness, medications, nutrition, activity, fracture history, and family history which can help to identify potential risk factors for bone fragility [116]. Recommended laboratory tests include a complete blood count, sedimentation rate, serum calcium, phosphorus, alkaline phosphatase, intact parathyroid hormone, 25OHD, blood urea nitrogen (BUN), creatinine, celiac screen, and urinary calcium to creatinine ratio. In addition, measurement of sex, thyroid, and growth hormones and genetic testing for OI may be indicated depending upon the clinical situation.
Future Directions for Densitometry
The future role of DXA in the management of pediatric patients appears promising, bolstered by advances in two areas of clinical investigation. Valuable insights have come from studies comparing DXA findings with those using the newer 3-dimensional densitometric techniques (discussed in detail in Chap. 11). Quantitative computed tomography (QCT) , peripheral QCT (pQCT) , and high-resolution pQCT (HRpQCT) capture elements of bone microstructure, geometry, and volumetric BMD not possible with DXA. These devices can also evaluate the trabecular and cortical compartments of bone separately. QCT, pQCT, and HRpQCT remain largely research tools because of a lack of standardized protocols for acquiring and analyzing scans, cross-calibration problems between devices, and the paucity of normative data. They have been used to investigate the process of skeletal development in both healthy youth and those with chronic illness. Comparisons of the longitudinal changes seen using 2-dimensional DXA with those detected using 3-dimensional tools enhance our interpretation of DXA findings. One study contrasted whole-body BMC and spine BMD using DXA with changes in tibial pQCT parameters 12 months following renal transplantation [144]. Changes in spine BMD correlated with changes in trabecular BMD at the tibia while whole-body BMC Z-scores correlated with tibial cortical area Z-scores . These observations suggest that DXA measures of spine BMD reflect changes in trabecular bone while whole-body BMC reflects cortical bone size but not volumetric density.
The potential for DXA to guide clinical management has also been confirmed from observational studies of pediatric patients requiring chronic glucocorticoid therapy (as described in detail in Chap. 10). Incident vertebral fractures were observed during the first year of steroid treatment in 6 % of those with rheumatologic disorders [145] and 16 % of those with ALL [143]. Children with lower spine BMD at baseline [143] and those who experienced greater decreases in BMD Z-scores during the first 6 months of steroid treatment [145] were more likely to sustain a fracture. As more data are gathered from similar observational studies, it may be possible to predict those patients at high risk for fracture with sufficient certainty to justify trials with bisphosphonates to prevent fracture before a first one occurs.
Summary
Early skeletal development is a key determinant of lifetime bone strength or fragility. The rationale for optimizing bone acquisition is to reduce the risk of fragility fractures from osteoporosis. DXA will likely remain the recommended tool to monitor bone health in clinical practice for the foreseeable future. DXA’s value in managing pediatric patients has been enhanced by improved normative data, guidelines for densitometry use, comparison of findings using DXA and 3-dimensional densitometry techniques, and studies exploring the relationship between DXA and fragility fractures in younger patients.
Summary Points
Peak bone mass attained by early adulthood serves as the “bone bank” for life
Optimizing peak bone mass in childhood and adolescence is an important strategy to reduce the lifetime risk of osteoporosis
Heritable factors determine 60–80 % of the variability in peak bone mass with hormones and modifiable factors such as diet and activity accounting for the remainder
The expected gains in bone mass and geometry can be compromised by a number of chronic genetic or acquired disorders in childhood or adolescence
Bone densitometry is an important tool to assess the skeletal effects of these disorders and DXA is the preferred method for clinical use
The interpretation of bone densitometry in pediatrics requires consideration of changes in bone size, shape, and mass through the first two decades of life
Densitometry results from pediatric patients should be compared with age, sex, and ethnicity-matched reference norms to calculate a Z-score. T-scores (using young adult reference norms) should not be used
Criteria for the diagnosis of osteoporosis in childhood and adolescence include low BMC or BMD for age plus a history of several nonvertebral fractures or a low-trauma vertebral fracture alone.
References
1.
Bonjour JP, Theintz G, Buchs B, Slosman D, Rizzoli R. Critical years and stages of puberty for spinal and femoral bone mass accumulation during adolescence. J Clin Endocrinol Metab. 1991;73:555–63.PubMed
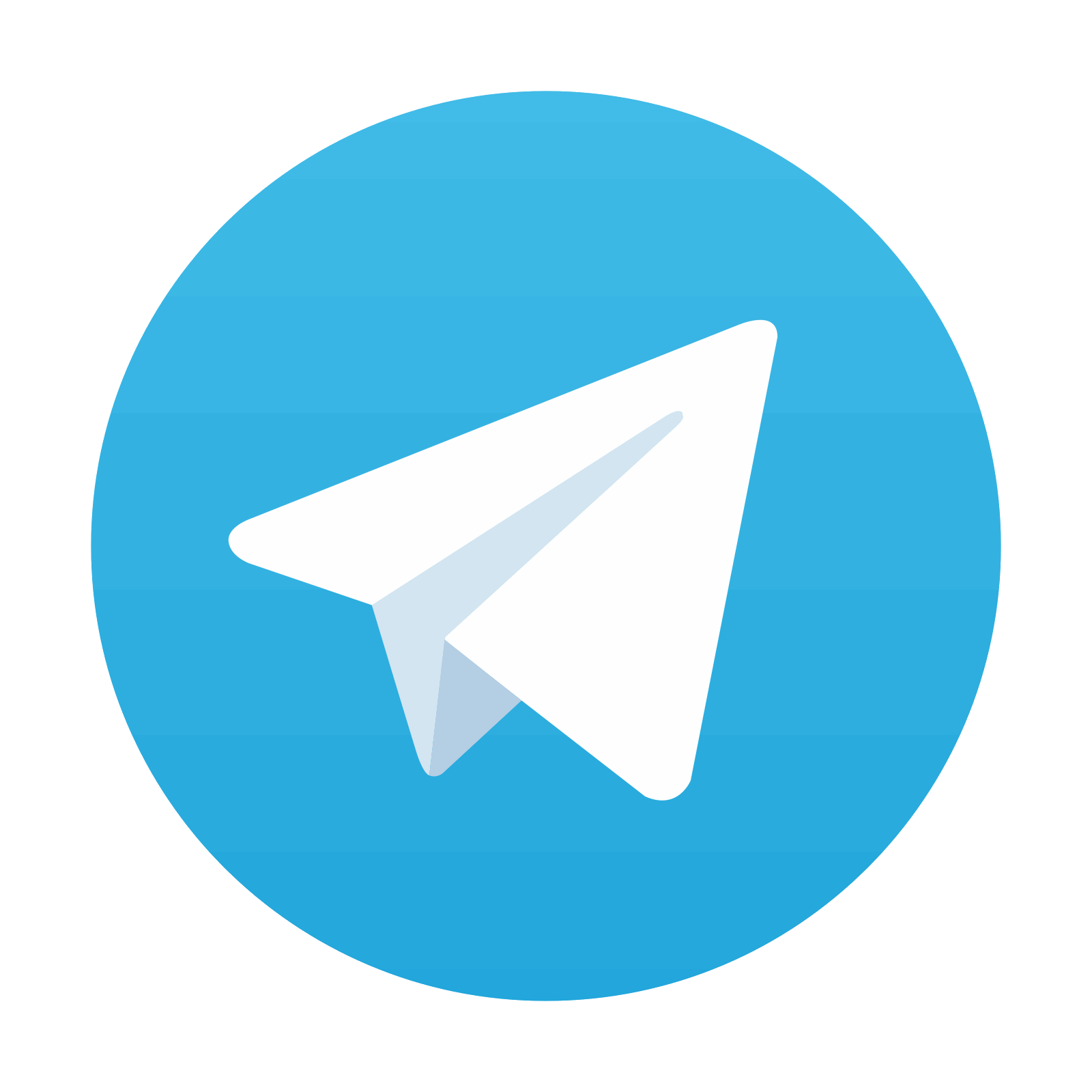
Stay updated, free articles. Join our Telegram channel

Full access? Get Clinical Tree
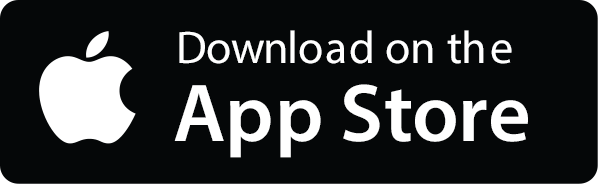
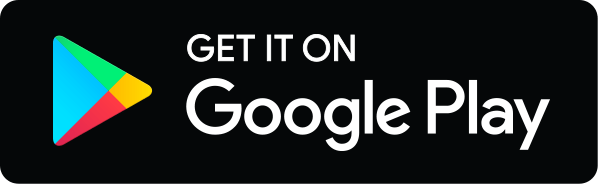