Fig. 15.1
A schematic diagram illustrating a knee joint being tested on a robotic/universal force-moment sensor (UFS) testing system. The knee can be placed at preselected angles of knee flexion, leaving the other 5-degree of freedom joint motion (anterior-posterior, proximal-distal, medial-lateral, varus-valgus, internal-external, flexion-extension)
There are a number of distinct advantages of the robotic/UFS testing system. This testing system can repeat exact positions as well as paths of motions of a joint [33, 32]. The data on 6-DOF kinematics and in situ forces in the ACL can be obtained from the same joint specimen; thus, it eliminates interspecimen variation and offering a higher statistical power using repeated measures ANOVA. It allows for noncontact measurement of in situ forces when the joint is subjected to externally applied loads. It has been particularly important for determining the function of the ACL and other knee ligaments including joint kinematics [35], in situ forces [32], the effect of knee motion and external loading on the length of the ACL [30], the effect of joint constraints on the in situ load distribution in the ACL [36], and the interdependence of the medial meniscus and ACL [37, 38]. This powerful instrument has been adopted by more than 30 laboratories worldwide.
15.4 New Therapies for ACL Healing
In addition to early work on biological augmentation to heal partial ACL injuries, Murray and coworkers have used collagen-platelet-rich plasma (C-PRP) as a bioscaffold for healing of a fully transected and sutured porcine ACL [39]. At 4 weeks, the linear stiffness under uniaxial tension and the load at yield and at failure were more than 2 times that of the suture repaired control group [39]. These improvements persisted up to 3 months postoperatively with a 320% increase in linear stiffness and a 76% greater yield load than suture repair alone [40].
In our research center, we have chosen a different approach for ACL healing. Our work was based on the successful use of ECM-SIS as this bioscaffold derived from porcine ECM has improved the healing of a middle third patellar tendon defect as well as healing of MCL with a 6 mm wide gap injury. Thus, we chose to employ ECM-SIS as well as its hydrogel to heal a fully transected ACL in a goat model.
15.4.1 Selection of Animal Models
A number of in vivo animal models have been used to investigate biotherapies for healing of ligaments and tendons. Rodent models were not frequently used because of their small size making it difficult to perform surgical repair accurately. For ACL reconstruction, large animals such as dogs, pigs, goats, and sheep are preferred. Based on a comparative study of the stifle joint of a number of experimental animals, the goat is a good choice partly because there are large volumes of data in the literature that could be used for comparison [41–43].
Ng and coworkers published an animal study on ACL reconstruction in a goat model where a patellar tendon graft was used. These authors showed that after surgical reconstruction, the structural properties of the graft had increased gradually over time from 6 weeks up to 3 years after surgery. At 3 years, the stiffness and ultimate load were 49% and 44%, respectively, of those for the sham-operated controls [42]. In our research center, we have also used a goat model to study the results of the reconstructed ACL after using a bone-patellar tendon-bone (BPTB) graft for surgical reconstruction. In terms of joint function, the anterior-posterior tibial translation under 67-N anterior-posterior tibial load increased up to 47% from 3 to 6 weeks, while the in situ force of the graft decreased as much as 22% [43]. Histological evaluation also showed a better incorporation between the bone block and femoral tunnel than that between the tendinous part and tibial tunnel.
The goat model was also used in another study to examine whether a “press-fit” fixation of BPTB grafts would function as well as interference screw fixation for ACL reconstruction. At time zero, no significant difference in the viscoelastic and tensile properties could be demonstrated between the two groups, although the ultimate load at failure was 34% lower for the “press-fit” group [44]. A third study to determine if the initial graft tension had any effects on graft healing after ACL reconstruction was also performed. It was found that although high initial graft tension could replicate normal knee kinematics better at time zero, these positive effects were diminished by 6 weeks postsurgery [41].
15.4.2 Biological Augmentation
Using the same animal model, the potential advantages of using ECM-SIS to heal the transected ACL were examined [10, 45, 46]. The ACL was first surgically transected and then underwent suture repair. Then, an ECM-SIS sheet was used to wrap around the injury site before injecting ECM hydrogel into the transected site (Fig. 15.2). Suture repair alone served as the control group (Fig. 15.2a). After 12 weeks, the CSA of the ACL following ECM-SIS treatment was 4.5 times over that of the suture repair group (127 ± 90% vs. 34 ± 25%, respectively). The function of each stifle joint was assessed using the robotic/UFS testing system. The anterior-posterior tibial translation for the ECM-treated group, in response to a 67-N anterior-posterior tibial load, had statistically significant improvement as compared to those for the suture repair control. At 30° of joint flexion, the anterior-posterior tibial translation was 8.6 ± 2.0 mm which was 27% less than that of the control group (Table 15.1). The in situ force in the healing ACL was also found to be nearly two times higher than that of the control group (Table 15.1). Similar results were found at 60° and 90° of joint flexion.


Fig. 15.2
Cartoon drawings illustrating the two groups of goats for ACL healing (1) suture repair (control) group, (a) suture repaired alone, and (2) ECM-treated group, (a) suture repaired, plus (b) ECM bioscaffold applied, and (c) ECM hydrogel injected
Table 15.1
Anterior-posterior tibial translations (APTT, in mm) of the goat stifle joint and corresponding in situ force in the ACL (N) at 30° of joint flexion in response to an externally applied a 67-N anterior-posterior tibial load (mean ± SD)
APTT (mm) | ACL force (N) | |
---|---|---|
I. ECM-treated group | ||
Sham-operated control | 3.4 ± 0.4 | 62 ± 5 |
Experimental | 8.6 ± 2.0* | 52 ± 11 |
II. Suture repair group | ||
Sham-operated control | 3.8 ± 0.2 | 50 ± 12 |
Experimental | 11.8 ± 3.4* | 26 ± 24 |
Afterward, the healing ACL was exposed, and an abundance of continuous neo-tissue formation could be seen, but there was hypertrophy. Histologically, the healing ACL had an organized collagen matrix with spindle-shaped cells. Tensile testing of the FATC was done, and the stiffness for the treated group was 2.4 times that of the control group. These results suggest biological augmentation could indeed enhance ACL healing [10].
We had also conducted a preliminary experiment involving a longer time point (26 weeks). In this case, the healing of the ACL continued as its collagen fibers had become completely aligned and packed. During tensile testing, the mode of failure was at the femoral insertion site rather than the original transection site. While the healed ACL had become stronger, the insertion sites became weakened due to the lack of adequate loading of the entire FATC during the slow healing process. These results also suggested that some form of mechanical loading of the ACL during its healing would be needed to limit the deleterious effects of disuse atrophy of its femoral and/or tibial insertion sites.
15.4.3 Mechanical Augmentation
Literature has suggested that mechanical augmentation by means of sutures could help to stabilize the knee with an injured ACL. Fleming and coworkers used suture augmentation, in which sutures were passed from bone to bone in a porcine model to reduce anterior joint laxity [47]. In our research center, we have also used sutures for mechanical augmentation in a series of goat experiments. Our first study was to determine the best location of the tunnels for suture (#2 Fiberwire sutures) augmentation on stifle joint stability following a complete ACL transection [48]. We have found that placing the sutures from the anterior footprint of the femoral origin (FA) to the medial aspect of the tibial footprint (TM), the anterior tibial translation (ATT) as measured by the robotic/UFS testing system was restored to within 3 mm of the intact joint following suture augmentation, and the in situ force was similar to that of the intact ACL (Fig. 15.3). Furthermore, these data represented a 54–76% improvement over suture repair. It was concluded that suture augmentation, when placed close to the ACL insertion, could provide good initial joint stability which could aid ACL healing. Another study also showed the relative contribution of the soft tissues in resisting the anterior tibial load. Under the 67-N anterior tibial load, the ACL provided the dominant support in the intact joint. In the case of ACL-deficiency, the MCL and medial meniscus carried significant loads (Table 15.2). At 30° of joint flexion, these loads reached up to 36% and 53% of the intact ACL, respectively. Following suture repair of the ACL, the in situ force was 81% of the intact ACL, while that for the augmentation sutures was 103% (Fig. 15.4). Similar results were seen for 60° and 90° of joint flexion [49]. It could be concluded that suture augmentation had provided sufficient initial joint stability and concomitantly lowered the loads on the MCL as well as the medial meniscus and, potentially, reduced their chance of injury from overloading.



Fig. 15.3
Diagram depicting tunnel locations for augmentation sutures. (a) FA is located anterior to the ACL footprint at the femoral origin, and (b) TM is tunnels located medial to the footprint at the tibial insertion
Table 15.2
The anterior tibial translation (ATT, in mm) and in situ forces (N) in the ACL of the stifle joint at 30° of joint flexion in (1) the intact, (2) ACL-deficient, and (3) suture-augmented states in response to 67-N anterior tibial load (C) (mean ± SD)
ATT (mm) | In situ force (N) | |
---|---|---|
1. Intact state | 2.3 ± 0.5 | 69 ± 6 |
2. ACL-deficient state | 15.3 ± 2.2* | NA |
3. Suture-augmented state (FA/TM) | 5.2 ± 1.9 | 60 ± 4* |

Fig. 15.4
Relative contribution of (a) ACL, (b) medial collateral ligament (MCL), and (c) medial meniscus at various states of the stifle joint in response to a 67-N anterior tibial load at 30° of joint flexion. Data normalized by the intact ACL
Finally, an in vivo study was done to see whether suture augmentation could actually heal a surgically transected ACL [50]. After 12 weeks, the anterior-posterior tibial translation for the suture augmentation group was about 20% lower than that of the suture repair group, while the in situ force in the healing, ACL was more than 50% higher than the suture repaired ACL. Upon dissection, the ACL was found to heal with significant neo-tissue formation. In terms of the structural properties of the FATC, the linear stiffness was 75% greater than that of the suture repair group. It was concluded that suture augmentation could provide the needed stability to the stifle joint for the intrinsic healing of the ACL to take place. In addition, such augmentation would reduce the forces carried by secondary structures in an ACL-deficient stifle joint in order to help avoid injury to the MCL and medial meniscus.
15.4.4 Combined Biological and Mechanical Augmentation
With the knowledge that biological and mechanical augmentation could each individually enhance ACL healing, we then asked the research question whether their combination may work synergistically to further accelerate the healing process. We hypothesize that since the mechanical augmentation would stabilize the knee immediately after surgery, it could help the biological augmentation to stimulate and accelerate the ACL healing.
For this purpose, we designed a novel Mg-based ring to bridge the gap between the two torn ends of the ACL (Fig. 15.5). It serves as an internal splint for mechanical augmentation while simultaneously load the healing ACL as well as its insertion sites to prevent disuse atrophy. The function of the Mg ring repaired ACL was first evaluated in an in vitro study using a goat model. Following the application of an Mg-based ring, the anterior tibial translation of the repaired ACL was reduced by 60–70% from the ACL-deficient state as measured by the robotic/UFS testing system. This was within approximately 3 mm of that of the intact joint [51]. Mg ring repair could also restore the in situ forces of the repaired ACL to within ±5 N of the levels of the intact ACL [51]. These data suggest that the Mg-based ring is indeed a suitable device for mechanical augmentation.


Fig. 15.5
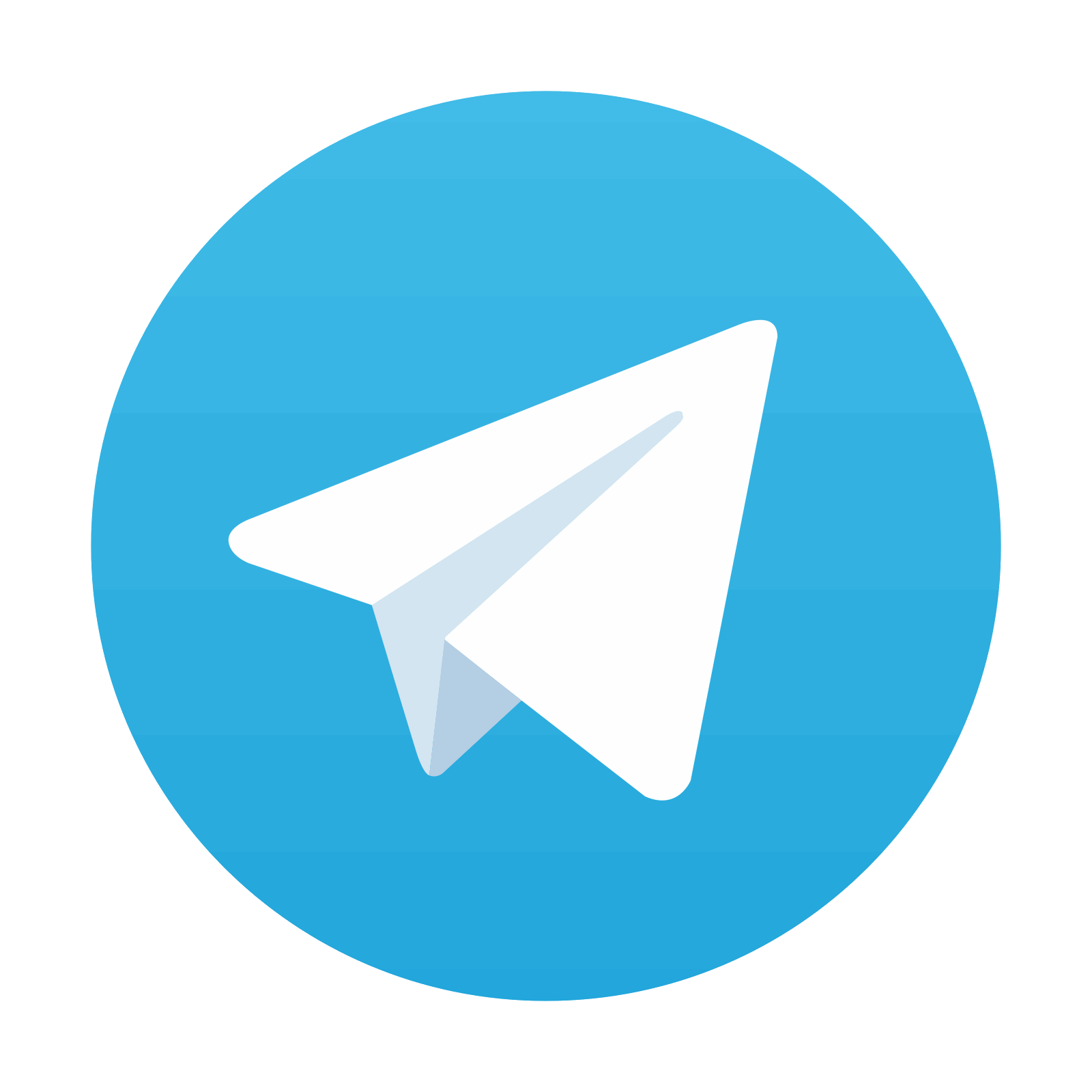
A schematic diagram showing the application of the Mg-based ring device to bridge the transected ACL. Fixation sutures through the femoral bone tunnels are fixed using a surgical button, while those through the tibial bone tunnels are fixed using a double-spiked plate and fixation post
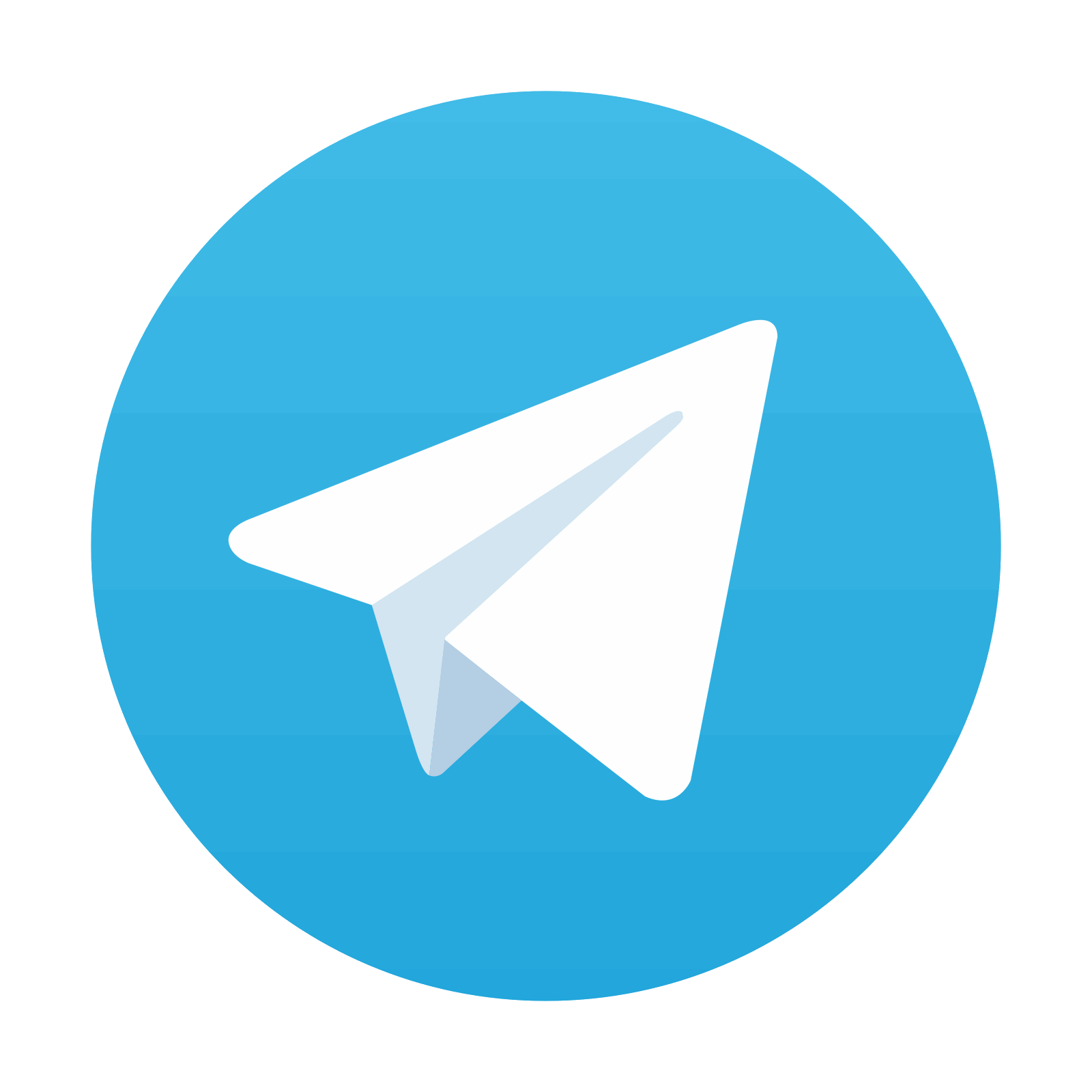
Stay updated, free articles. Join our Telegram channel

Full access? Get Clinical Tree
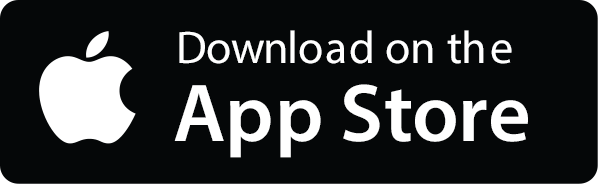
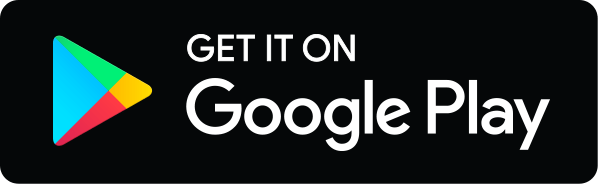
