Fig. 44.1
Lipoaspiration of abdominal adipose tissue performed in the clinic setting
Subcutaneous adipose tissue is rich in mature adipocytes (67.6%), but also consists of blood vessels, leucocytes, fibroblasts, macrophages, and pre-adipocytes, identified as stromal vascular fraction (SVF) [18–20]. Each adipocyte is completely surrounded by a capillary system, thus explaining why the concentration of MSCs in adipose tissue is five times greater than that of the bone marrow [21, 22]. The possible therapeutic applications of stroma and the associated stromal vascular fraction that contains a high prevalence of stem/stromal cells have led to increasing interest in the clinical applications of adipose tissue, and the number of clinical trials involving this therapy is greatly expanding.
Despite advances in isolating human ASCs, a number of obstacles remain, including the difficulty of ex vivo expansion, the poor delivery efficiency (less than 5% of transplanted cells are retained after transplantation), and the uncertain fate of these cells in vivo [23]. These are issues that may minimize the potential benefits of cell expansion. Moreover, regulatory approval of using these stem cells in a clinical setting after they have been subjected to extensive manipulation, including ex vivo expansion, is remarkably delayed due to requirements for compliance with “cell Practice (cGMP) Guidelines”. These restrictions, however, are not applied in the case of minimal manipulation (Regulation (EC) No. 1394/2007 of the European Parliament and Council). Developing processing technologies to obtain suitable isolates of autologous human ASC products with minimal manipulation are, therefore, highly desirable for clinical applications.
44.4 Clinical Applications of Adipose-Derived Mesenchymal Stem Cells
There has been a great quantity of in vitro and preclinical data collected over recent years related to MSCs, and the clinical application of MSCs has been examined in numerous trials to treat a range of orthopaedic conditions that include chondral defects [24], osteoarthritis [25], tendinopathy [26], intervertebral disc disease [27], and bone non-union/delayed union [28].
In preclinical studies, research by Toghraie et al. reported that a single injection of ASCs, withdrawn either from the infrapatellar fat pad or subcutaneous tissue, led to repair of the cartilage in the case of osteoarthritic lesions [29, 30]. Specifically, in rabbits where osteoarthritis had been induced by unilateral anterior cruciate ligament transection, improvements in the quality of cartilage were identified in those treated with ASCs. Furthermore, there was a reduction in clinical signs associated with osteoarthritis, such as decreased osteophyte formation and cartilage ossification, in the ASC treatment group. Findings also demonstrated reduced immunological response, which may be attributed to the low immunogenicity and anti-inflammatory characteristics of ASCs, which secrete several soluble factors, including TGF-beta, prostaglandins, and interleukin (IL)-10. Findings consistent with this research were reported by Ude et al., where chondrogenic properties of ASCs and bone marrow-derived MSCs were demonstrated in the treatment of osteoarthritis in a sheep model [31]. In the clinical setting, ASCs have been available commercially for veterinary use since 2003. Beneficial effects of a single ASC injection have been reported in the treatment of elbow and coxofemoral joints in dogs affected by chronic osteoarthritis [32–34].
Following these initial animal studies, a number of in vivo studies have been conducted using human subjects who have undergone scaffold-based surgery or ASC intra-articular injections to treat chondral and osteochondral injury. Koh and Choi [35] reported encouraging results after 2 years of follow-up in cases of knee OA treated with intra-articular injection of infrapatellar fat pad-derived mesenchymal stem cells [35]. Furthermore, Koh et al. [36] reported clinical improvement 2 years after treatment, in addition to improved or stabilized cartilage status at second-look arthroscopy [36].
44.5 Clinical Applications of Lipoaspirates
Isolated ASCs in the form of lipoaspirate have been investigated using an in vitro protocol to examine the effectiveness of intra-articular autologous lipoaspirate injection, as an alternative to cell-based therapy in the treatment of cartilage injury and as a method to inhibit the progression of degenerative cartilage changes. Recently published research has evaluated the ability of lipoaspirate to promote the outgrowth of cells capable of repopulating chondrocytes, to repair damaged cartilage, and to contribute paracrine effects on resident chondrocytes.
Lipogems® (PCT/IB2011/052204) is an innovative close-loop processing system that allows for the minimal handling of adipose tissue (solely via mechanical action), the maintenance of the SVF niches, the reduction of fat clusters to allow injection with fine needles, and the removal of impurities such as oil residues and blood (Fig. 44.2). The inspiration for the development of this product came from the Coleman lipofilling technique [37]. Similar to the Coleman method, the goal of the Lipogems system is to isolate a homogeneous and micronized fraction of adipose tissue, therefore allowing a patient’s subcutaneous fat to be processed for autologous reinjection within the operative procedure time [38]. The device is able to simplify the original macrostructure of the adipose tissue in order to progressively reduce the adipose matrix and to obtain transplantable adipose clusters while at the same time minimizing cellular trauma and optimizing the concentration of viable cells. Due to these design features, Lipogems® has been approved for use in the USA as a class II device intended for transferring autologous adipose tissue for aesthetic body contouring (lipofilling) in applications that include plastic and reconstructive surgery, neurosurgery, gastrointestinal and affiliated organ surgery, urological surgery, general surgery, orthopaedic surgery, gynaecological surgery, thoracic surgery, and laparoscopic surgery.


Fig. 44.2
Processing cylinders of the Lipogems® system containing stainless steel balls to emulsify the lipoaspirate into microfragments
A final product of microfragmented adipose tissue containing ASCs can be obtained by processing at least 60–80 mL of lipoaspirate. This process is uncomplicated and performed relatively rapidly, as the adipose tissue undergoing processing is continually maintained in a liquid suspension. This ensures minimal cellular damage and debris formation. Prior to processing, a sterile saline solution is used to fill the core of the device, which is a plastic cylinder containing five stainless steel beads. The fragmentation of adipose tissue is performed in sequential steps. Firstly, the lipoaspirated tissue contained within a syringe is transferred into the Lipogems device, passing through a large filter on the upper portion of the device which provides for the first reduction of the adipose clusters. This passage is achieved through a closed system to ensure maintained sterility of the tissue. As the lipoaspirate is moved into the Lipogems® device, the same quantity of saline solution is transferred to a disposal bag. By shaking the device vigorously, the stainless steel beads exert mechanical forces that microfragment the adipose tissue and emulsify the various components and phases contained within the device (oil residues, saline solution, and blood). This step is fundamental in order purify the ASCs, which occurs after several washes with the saline solution that systematically removes the aforementioned components. During this procedure, when the device is positioned vertically, the various components contained within are separated by gravity. The adipose tissue is contained within the first stratification at the top of the device, whereas the saline solution, containing blood, remains on the bottom [39]. Once the saline solution injected into the device becomes clear and separated from the yellow lipoaspirate layer, the saline solution injection is stopped and the device turned upside down. The final step of the process is the purification of adipose tissue, now composed of a floating layer of adipose clusters, which must be pushed through a filter into a 10 mL syringe. The passage through the filter provides additional size reduction of the clusters. The final isolate of ASCs is then ready for the desired clinical purpose (Fig. 44.3).


Fig. 44.3
(a) Lipogems® system; (b) 60 cc syringe containing unprocessed lipoaspirate and six 10 cc syringes containing microfragmented adipose tissue; (c) intra-articular knee injection of microfragmented adipose tissue cell suspension, 10 cc syringe
Bosetti et al. studied the lipoaspirates obtained from patients undergoing liposuction from abdominal subcutaneous adipose tissue in order to examine the ability of lipoaspirate-derived cells to repopulate adipose tissue [41], to examine the phenotype and differentiation potential of these repopulating cells, and to determine effects of lipoaspirate on human chondrocyte proliferation and production of cartilage matrix. Lipoaspirate was separated into different samples and processed by several methods that consisted of the PureGraft® system, the Lipogems® system, Coleman’s protocol, and a control sample. Histologic comparison of the lipoaspirates obtained from each of these four techniques demonstrated that the Lipogems® process resulted in the most significant changes in cellular organization, with only a few areas of adipose tissue identified and the remainder being almost completely replaced by collagen and glycosaminoglycans. The lipoaspirates obtained using both Coleman’s method and Lipogems® induced chondrocyte proliferation and extracellular matrix deposition and resulted in good cell outgrowth from cell clusters. Comparing these treatment groups, lipoaspirates obtained using Lipogems® demonstrated faster outgrowth and increased number of cells using a 3D collagen matrix. The authors concluded that the isolation and expansion of stem cells are unnecessary prior to autologous implantation.
44.6 Summary
Mesenchymal stem cells are easily sourced from adipose tissue in the form of adipose-derived stem cells. These cells can be harvested and processed in the outpatient setting for immediate clinical use, without the need for cellular cultivation. Recent technological developments allow for the microfragmentation of autologous adipose tissue that contains intact tissue niches and cell clusters. These isolates have demonstrated great potential for use in a wide array of chondropathic conditions, from localized traumatic cartilage injury to more advanced and diffuse changes consistent with osteoarthritis. Available outcome data for treatments using adipose-derived stem cells is preliminary. Further outcome analysis, including randomized trials, will be necessary to better clarify ideal indications for this therapy.
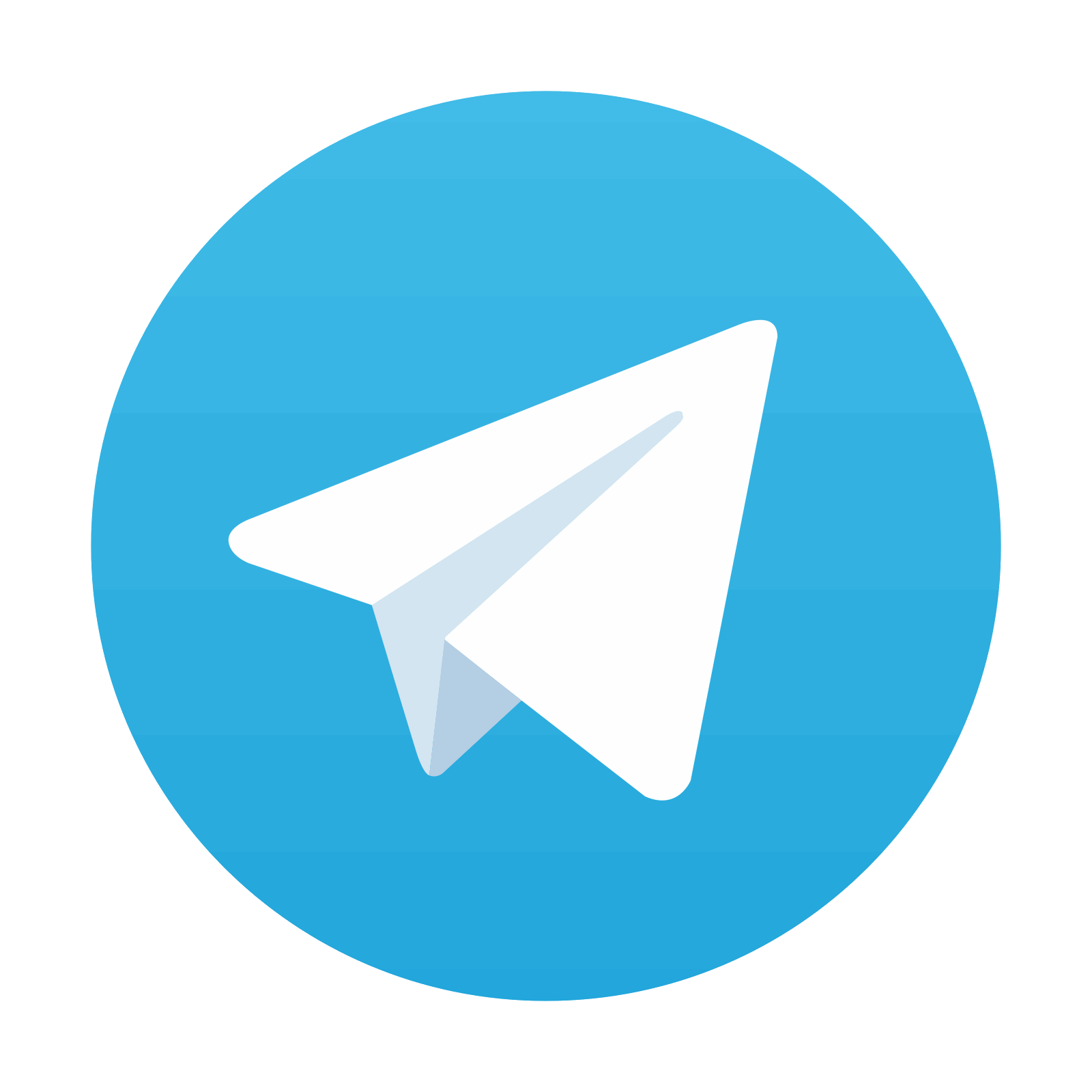
Stay updated, free articles. Join our Telegram channel

Full access? Get Clinical Tree
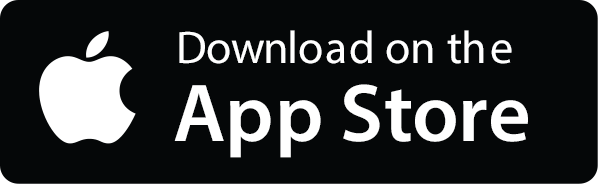
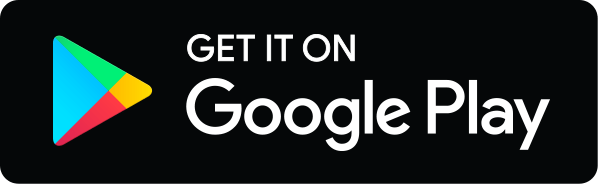