Fig. 34.1
Schematic representation of phases of fracture repair, inflammation/hematoma, repair (soft callus and hard callus formation), remodeling
The inflammation phase peaks at 24 h after initial fracture and continues for approximately 1 week after the fracture occurs. An inflammatory response is elicited and MSCs migrate to the fracture site; these cells activated to perform immunosuppressive functions termed licensing [25]. Fracture of the bone causes the interruption of vascular endothelium integrity of bone and the surrounding tissues; in this fracture gap, fibrinogen is converted into fibrin, leading to the formation of fracture hematoma. The environment is characterized by hypoxia, low pH, high lactate, and potassium concentrations [26]. Local tissue injury and immunologic reaction to the necrotic and hypoxic conditions evoke an immunologic reaction in both innate immune system and adaptive immune systems (PAPE). Acute inflammatory cells; polymorphonuclear neutrophils (PMNs) followed by macrophages migrate to the region. The primary role of the PMNs is the modulation of the inflammatory response by releasing numerous cytokines and chemokines although they contribute to phagocytosis of the damaged cells and debris, and protection against infection [27–29]. This modulation is very important, under excessive inflammatory conditions; hyper-activated PMNs may aggravate tissue damage and diminish healing. Improved bone healing has been found in animal models by inducing neutrophilia [30] although studies found improved bone generation by depleting neutrophils [31–34]. A balanced adjustment of PMNs is necessary for regular bone regeneration. By releasing chemokines, PMNs attract macrophages, which further eliminate pathogens and initiate tissue repair. The initial inflammatory response involves secretion of IL-1, IL-6, TNF-α, IFN-ϒ, IL-11, and IL-18 from the inflammatory cells [21].
IL-1 secreted from macrophages during inflammatory phase triggers the release of IL-6, GMCSF, and MCSF; it stimulates angiogenesis and formation of soft callus [35, 36]. The second peak of IL-1 corresponds to 3 weeks after injury; this release is mainly expressed by osteoblasts and promotes degradation of callus tissue and stimulation of the remodeling [37, 38]. The diverse function of IL-1 during fracture healing is attributed to the differential expression of IL-1 receptors, IL-1 receptor I and IL-1 receptor II [37]. TNF-α is produced by macrophages, NK cells, and T-lymphocytes and highly expressed during both inflammatory and repair phases. TNF-α mediates the invasion of MSC into the bone fracture site and stimulates the production of M-CSF by mesenchymal stem cells (MSCs) [37, 39]. Similar to IL-1, TNF-α release shows biphasic pattern and the effects are related to two receptors, TNFR1 and TNFR2 [40]. IL-6 is produced by osteoblasts in response to stimulation of IL-1 [38]. Parallel to IL-1 and TNF-α, the IL-6 expression is peaked in early inflammatory phase, but in contrast IL-6 levels immediately return to the baseline amounts by the end of the first week and remain constant during further phases. IL-6 stimulates angiogenesis, vascular endothelial growth factor (VEGF) production, and differentiation of osteoblasts and osteoclasts [38, 41]. Another pro-inflammatory cytokine Interferon-ϒ (IFN-ϒ) levels rise in response to bone injury and remain elevated through fracture healing phases and return to the baseline in the late remodeling phase [42]. IFN-ϒ plays an important role in licensing MSCs and may trigger the proliferation and immunomodulatory function of MSCs [43]. Also, recent studies show that inhibition of T-cells and functions of IFN-ϒ may be useful for bone-mediated allogenic MSCs [44].
MSCs are required to migrate from sources such as the periosteum, bone marrow, and circulation. Migration of MSCs to the fracture site is mediated by several growth factors, cytokines, and chemokines including stromal derived factor-1 (SDF-1 or CXCL12), chemokine motif ligand 7 (CXCL7), TNF-α, monocyte chemotactic protein-1 (MCP-1), and macrophage inflammatory protein-1 α (MIP-1 α) [45–50]. Recruitment of MSCs to the fracture site is believed to occur in early phases of fracture healing by day 1 [51]. Activation of MSCs is mediated through TNF-α, IFN-ϒ, IL-17, Toll-like receptors (TLRs), BMPs, Oncostatin M, and interleukin-22 (IL-22) [25, 37, 43, 52–58]. Suppression of inflammatory response is critical to the further initiation of repair phase of fracture healing. Besides proinflammatory cytokines (IL-1, IL-6, IL-8, IFN-ϒ, and TNF-α), anti-inflammatory cytokines such as; IL-1 receptor antagonist (IL1-ra), IL-4 and IL-10, found to be higher concentrations in fracture hematoma this heterogeneity represents a dual control on the inflammatory response [59]. TGF-β2 and TGF-β3 levels that peak at the end of the inflammatory phase may have a role to reduce immune response [60]. The activated MSCs suppress the inflammatory response by direct feedback mechanism [25]. With the suppression of the inflammatory response, repair phase starts although some immune cells and cytokines continue their roles during the following phases.
34.2.5.2 Repair Phase: Soft Callus and Hard Callus Formation
Granulation tissue forms after hematoma facilitating vascular ingrowth and angiogenesis. Within the granulation tissue, endochondral formation occurs in between the fracture ends. Following the differentiation of MSCs into chondrocytes and osteoblasts, the cartilaginous tissue forms a soft callus. Periosteum, bone marrow, and endosteum are three sources of osteoblasts and osteocytes whereas chondrocytes within the fracture callus are primarily derived from the periosteum. Types I and II collagen are produced in order to form a matrix that restores stability to the bone ends. This corresponds roughly to the time when the fragments are no longer moving freely, approximately 2–3 weeks after the initial fracture occurs. TGF-β, FGF-1 and 2, PDF and IGF-1 are all expressed in the soft callus and regulate chondrocyte differentiation. FGF-2 regulates expression of the transcription factor Sox-9, which activates the expression of type I, IX, and collagen genes and the gene for aggrecan protein [61].
The hard callus appears with the conversion of cartilage to a calcified cartilage matrix with terminal differentiation of the chondrocytes. Macrophages produce matrix metalloproteinases (MMPs) to degrade the cartilage matrix. These MMPs have a central role in soft-to-hard callus switch [11, 62–65]. Other immune cells also reappear during the mineralization of cartilaginous callus. This includes T- and B-lymphocytes that were found to be located in a close contact with osteoblasts and osteoclasts. These lymphocytes produce TNF-α which trigger the death of mature chondrocytes aiding the transition from cartilage into bone [28, 42, 66]. Monoclonal antibodies to BMP-2, 4, and 7 showed an increasing intensity of staining for the BMPs in periosteal mesenchymal cells in the region of hard callus formation, in the proliferating mesenchymal cells of the early soft callus and chondroblasts differentiated from these cells [61]. IL-17 is another cytokine that can affect the conversion of soft callus into the hard callus. Also, IL-17 enhances the MSC differentiation into osteoblasts [67]. Given the reduction in the number of chondrocytes, the dominant cell types during the hard callus phase are the osteoblast and osteoclast. Several growth factors including PDGF, TGF-β, IGF, fibroblast growth factor-1 (FGF-1), and BMPs involved in proliferation and the chondrogenic differentiation of MSCs as well as deposition of collagen [38, 68, 69]. Overall, the conversion of soft callus into hard callus is highly controlled by macrophages, T- and B-lymphocytes, and various cytokines and growth factors [25].
34.2.5.3 Remodeling
Fracture healing is completed during the remodeling phase in which the healing bone is restored nearer to its original shape, structure, and mechanical strength. Cutting cones are formed by osteoclasts that first remove the disorganized woven bone. Then, osteoblasts follow and lay down lamellar bone in an organized pattern around a central blood vessel. During remodeling, the canalicular architecture of bone is reestablished, and the Haversian system with its osteocytes is restored. This process starts in concert with bone consolidation and continues for months or years after a solid osseous union has been achieved. The remodeling of the fractured bone is mainly related to the balance between osteoblast and osteoclast functions under both systemic and local control pathways. The osteoblast/osteoclast function is controlled by MSCs, macrophages, and cytokines such as TNF-α and IL-17 [25]. The TNF family molecule RANKL and its receptor RANK are key regulators of bone remodeling [70]. Osteoprotegerin (OPG), a soluble decoy receptor for receptor RANKL, inhibits both differentiation and function of osteoclasts and decreasing bone resorption [71]. At the same time, IL-17 enhances the expression of RANKL on MSCs enhancing the osteoclastogenesis when co-cultured with peripheral blood mononuclear cells (PBMCs) [63, 72]. Another cytokine, TNF-α produced by MSCs and osteoblasts during the late phase of bone healing [37] can also influence both osteoblast and osteoclast functions demonstrating its vital role in the remodeling phase [73, 74]. Also, several systemic hormonal pathways are involved in bone remodeling. PTH, PTHrP, 1,25-20H vitamin D3, and leptin increase bone resorption by increasing osteoclast differentiation. Conversely, sex steroids inhibit osteoclast differentiation, while intermittent PTH administration and high doses of vitamin D3 stimulate osteoprogenitor cells to differentiate into osteoblasts.
34.3 Summary
The biological sequence of fracture healing is an overlapping process with many cytokines and growth hormones involved in different phases. The inflammatory phase is characterized by pro-inflammatory cytokines and inflammatory cells dominancy. MSC migration to the fracture site and licensing of these cells controlled by a chemokine cocktail. After the suppression of the initial inflammatory response repair phase starts and MSCs differentiate into osteoblasts and chondroblasts which form callus. Fracture healing is completed by remodeling which is regulated between osteoblast and osteoclast functions both systemic and local control pathways involved. Remodeling of the bone occurs slowly over months to several years. For successful bone remodeling, adequate blood supply and mechanical stability is essential. Moreover, with the current knowledge the final destination of the fracture is seemed to be controlled at the beginning with the early secretion of growth factors and cytokines further function in the later stages. With the recent advances, understanding of the biologic events occurs during healing phases, it will be possible to control healing process of a fracture at the beginning before any adverse outcomes occur (Table 34.1).
Table 34.1
Growth factors and cytokines released during fracture healing
Primary source cells and tissues during fracture repair | Effect on fracture healing | Reference | |
---|---|---|---|
Platelet-derived growth factors (PDGFs) | Platelets, monocytes, macrophages, and endothelial cells of fracture callus | Stimulates mesenchymal cell proliferation | [75] |
Initiate fracture repair and callus formation | |||
Insulin-like growth factors (IGFs) | Fracture callus, bone matrix | Stimulates osteoblast cell proliferation | [25] |
Fibroblast growth factors (FGF-1) and (FGF-2) | Inflammatory cells, osteoblasts, and chondrocytes within the fracture callus | Stimulates progenitor cell proliferation | [75] |
Promote blood vessel formation | |||
Transforming growth factor β (TGF-β) | Platelets, inflammatory cells, osteoblasts, osteoclasts, chondrocytes | Promotes angiogenesis | |
Stimulates progenitor cell proliferation | |||
Regulates cartilage matrix calcification | |||
Bone morphogenetic proteins (BMPs) | Osteoprogenitor cells, fibroblasts proliferating chondrocytes | Promotes fracture healing and bone regeneration | |
Granulocyte-macrophage colony-stimulating factor (GMCSF) | T-lymphocytes | Fibroblast migration and collagen synthesis | [76] |
Proliferation and differentiation of monocyte/macrophage | |||
Interleukin-1 (IL-1) | Macrophages (inflammatory phase) | Stimulates angiogenesis | |
Osteoblasts (repair phase) | Induces the secretion of IL-6, MCSF, and GMCSF | ||
Fibroblastic collagen synthesis and collagen crosslinking | |||
Promotes formation of soft callus | |||
Selective degradation of callus tissue | |||
Interleukin-6 (IL-6) | Osteoblasts | Stimulates angiogenesis, VEGF production | |
Differentiation of osteoblasts and osteoclasts | |||
Stimulation of bone resorption? | |||
TNF-α | Macrophages, (inflammatory phase) | Mediates the invasion of MSC into the bone fracture site and | |
Osteoblasts, NK cells, T-lymphocytes | Stimulates the production of M-CSF by MSCs | ||
Chondrocytes (repair phase, remodeling phase) | Licensing MSCs | ||
Interferon-ϒ (IFN-ϒ) | NK cells, T-lymphocytes | Licensing MSCs | |
Stimulates alkaline phosphatase activity in human osteoblasts | |||
Suppresses in vitro differentiation of osteoclasts | |||
Interleukin-17 (IL-17) | T-lymphocytes | Promotes bone formation | |
Facilitates bone fracture healing | |||
Enhances the MSC differentiation into osteoblasts | |||
Conversion of soft callus into the hard callus |
References
1.
Eriksen EF, Axelord D, Melsen F. Bone histomorphometry. New York: Raven Press; 1994.
2.
3.
Augustin G, Antabak A, Davila S. The periosteum. Part 1: anatomy, histology and molecular biology. Injury. 2007;38:1115–30.CrossRefPubMed
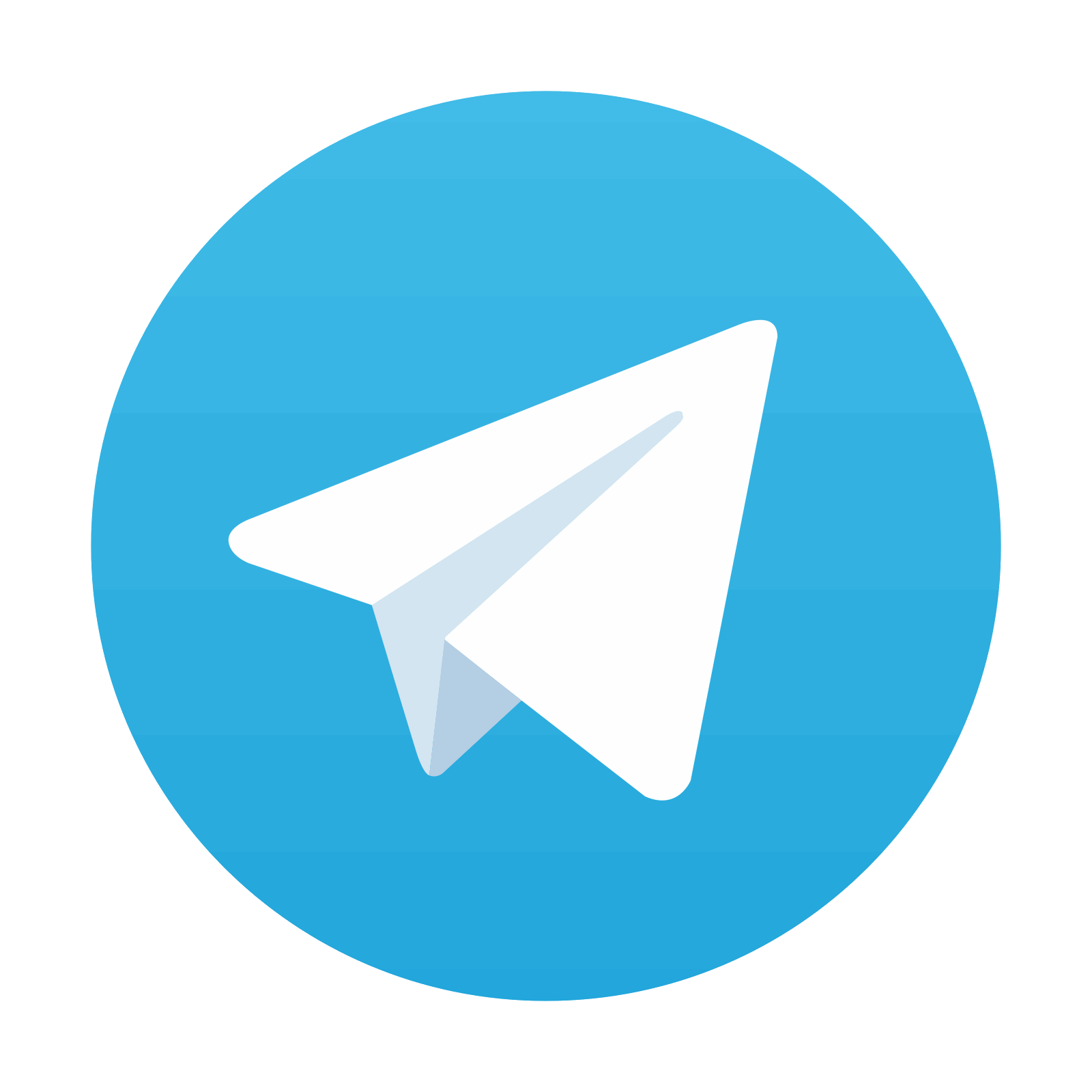
Stay updated, free articles. Join our Telegram channel

Full access? Get Clinical Tree
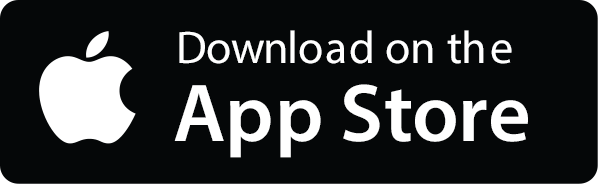
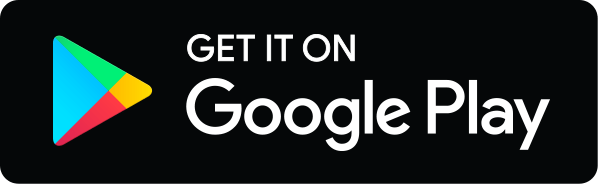