© ISAKOS 2017
Alberto Gobbi, João Espregueira-Mendes, John G. Lane and Mustafa Karahan (eds.)Bio-orthopaedics10.1007/978-3-662-54181-4_1717. Stem Cells in Joint Repair
(1)
Musculoskeletal Translational Medicine, Novartis Institutes for Biomedical Research Basel, Novartis Pharma AG, CH-4056 Basel, Switzerland
(2)
Medicine for Sports and Performing Arts, Department of Health and Sport Sciences, Osaka University Graduate School of Medicine, Osaka, Japan
(3)
Department of Orthopaedic Surgery, Osaka University Graduate School of Medicine, Osaka, Japan
(4)
Institute for Medical Science in Sports, Osaka Health Science University, Osaka, Japan
(5)
Center for Advanced Medical Engineering and Informatics, Osaka University, Osaka, Japan
17.1 Introduction
Autologous cellular therapies have been introduced in the treatment of articular cartilage defects in 1994 by Brittberg et al. [1] Indeed, autologous chondrocyte implantation (ACI) has been proven to restore hyaline-like articular surface, which is mechanically and functionally stable even in athletes at long-term follow-up. However, despite the breakthrough merit of the original procedure, it showed some issues such as local morbidity for periosteal harvest, complications related to the use of periosteum as a cover, and uncertain distribution of the cell suspension. In particular, the possible periosteal patch hypertrophy and the potential degenerative changes of chondrocyte that have been extensively passaged in vitro [2] prompted the development of improved and alternative techniques to overcome these limitations [3].
In order to improve the biological performance of the autologous chondrocytes, by providing a suitable three-dimensional environment, and to make the engineered autologous graft easier to handle, scaffold-based ACI techniques have been developed. In these techniques, autologous chondrocytes are seeded onto the scaffold after cell expansion and further cultured prior to implantation [4, 5]. However, some limitations of ACI still remain in these more recent procedures, such as the donor site morbidity and the consequent potential degenerative changes and the relevant variability and age-dependent decrease in the chondrogenic potential of autologous chondrocytes [6, 7].
For the abovementioned reasons, chondrogenic progenitor cells, obtained from stem cells from various mesenchymal tissues, could represent the mainstay of future cell-based or tissue-based therapies for the regeneration of cartilage. Selection of appropriate cells is of paramount importance, and the characteristic of each cell type can influence significantly the outcome. In this chapter, the potential cell sources of relevance for cartilage repair will be discussed.
17.2 Mesenchymal Cells
17.2.1 Bone Marrow-Derived Mesenchymal Stem/Stromal Cells (BMSCs)
Friedenstein et al. described BMSC from mouse bone marrow for the first time in 1966. Their high proliferative capacity and multi-lineage differentiation potential into not only mesodermal cells, such as osteoblasts, chondrocytes, and adipocytes, but also into other lineages, such as hepatocytes [8], neurons [9], and glial cell [10], have been reported. BMSCs have been used in various form for the regeneration of cartilage and other tissues. One of the first applications of BMSC for cartilage repair has been published by Wakitani et al. Briefly, authors reported the repair of rabbit osteochondral defects by the use of allogeneic BMSC suspended in a type I collagen gel [11]. This strategy was then applied in patients for the first time into two patellar cartilage defects cases [12]. In addition, they compared the efficacy of this method with the unseeded collagen gel in patients who received high tibial osteotomy for knee osteoarthritis (OA). Interestingly, there were no significant differences in the clinical outcome between cell-based and acellular groups, but significantly better histological repair in the cell-based group. This mismatch between clinical and histological features has been reported in other studies and prompts for a better understanding of the healing process and its impact on knee function. In addition, other clinical applications of BMSC have been proposed and confirmed the clinical value of this approach [13, 14].
Once MSCs are exposed to a chondrogenic microenvironment, either in vitro or in vivo, they can differentiate into chondrogenic cells and synthesize cartilaginous extracellular matrix. In this regard, the use of bone marrow aspirate concentrate (BMAC) cells, which contain multipotent MSCs and growth factors, coupled with chondroinductive biocompatible scaffolds, represents a promising strategy for regenerating cartilage tissue. Gobbi et al. prospectively followed up the patients with grade IV cartilage lesions of the knee who underwent one-step BMAC implantation with a hyaluronan-based scaffold and showed a satisfactory clinical outcome. Importantly, this proved to be a viable and effective option that is mainly affected by lesion size and number and not by age. In particular, it allows to address the >45 years population with functional outcomes that are comparable to younger patients at final follow-up [15, 16].
The one-step nature of BMAC-based strategies, being based on uncultured cells, makes it superior in terms of avoiding degeneration/dedifferentiation of the cells, multiple surgeries, and high cost for the in vitro cell expansion procedure, which needs cell processing facility under GMP conditions. However, it is not clear whether the high heterogenous cell population involving not only MSC (about 0.001~0.001% of the total nucleated cells in BMAC) but also other nucleated cells, such as endothelial cells and platelet which secretes many cytokines, contributes to cartilage repair. Nonetheless, the ease and cost-effectiveness of one-step cell therapies are highly valuable and make its clinical adoption more streamlined.
Long-term prospective randomized studies will be needed to confirm the value of BMAC-based strategies.
17.2.2 Adipose-Derived MSCs (ADSCs)
ADSCs are relatively more abundant in the body, easier to access (about 5% of the total nucleated cells in fat tissue) by outpatient-based plastic surgery procedures (lipodystrophy and breast augmentation), proliferate more rapidly, carry a lower harvesting risk than BMSC, and obtaining approximately 100 g of adipose tissue is considered a minimally invasive procedure, readily accepted by patients and characterized by a low morbidity and side effect rates [17]. In fact, it has been demonstrated that human processed lipoaspirate cells, isolated from liposuction procedures, could be induced to differentiate into osteogenic, adipogenic, chondrogenic, and myogenic lineages through incubation in specific media and showed increased expression of chondrogenic genes. In addition, ADSCs have been shown to regenerate cartilage in relevant preclinical animal models. Another valuable feature of ADSC is the slow progression of senescence, compared to BMSC [18]. Clinical trials using intra-articularly injected ADSC indicated the efficacy for the treatment of the knee osteoarthritis, prompting for further exploration of this approach [19].
However, the need for cell isolation and expansion still represents a hurdle to clinical translation. In this regard, ADSC in the form of stromal vascular fraction (SVF) may offer a more streamlined alternative, since SVF can be easily obtained with a 2-h long process from a lipoaspirate/liposuction. However, some authors claim adipose SVF alone may not be sufficient to regenerate cartilage in animals, and extrastimuli, such as platelet-rich plasma (PRP), are needed. As a matter of fact, only ADSCs in the form of SVF are currently allowed for clinical applications in humans. Culture-expanded ADSCs, although potentially more attractive, being a more pure and potent population for cartilage repair, require further clinical trials for regulatory approval, before entering routinely clinical practice.
17.2.3 Synovium-Derived MSCs (SMSCs)
When compared with other mesenchymal chondrogenic cells, SMSCs have been reported to demonstrate the greatest chondrogenic potential. SMSCs indeed hold great promise as a cell source for cell-based therapies for cartilage repair because of the relative ease of harvest and their strong capability for chondrogenic differentiation [20, 21]. When cultured in vitro to expand their number, SMCS displayed a long-term self-renewal capacity and expanded over at least ten passages in basal medium, with consistent growth kinetics and a cell surface marker phenotype consistent with that of MSC. Overall, a growing body of literature supports the use of SMSC as a chondrogenic cell source. In particular, they have been evaluated successfully with a scaffold-free strategy. A series of in vitro and in vivo experiments suggest that SMSCs, cultured in 3D in a scaffold-free system, are plastic, are adhesive, and are capable of chondrogenic differentiation, representing unique and clinically compliant implant for cartilage repair.
Based on this strong rationale, clinical trials in patients with articular cartilage lesions are ongoing, and preliminary results show the safety profile of this approach and a promising restoration of the articular surface at both MRI and histology level, which is paralleled also by an improvement in the clinical outcome (unique trial number: UMIN000008266).
17.2.4 Human Nasal Chondrocytes (HNC)
The use of HNC has been advocated to overcome the typical limitations of articular chondrocyte-based ACI procedures. HNC can generate cartilaginous tissues in a quality that is less dependent on the age of the donor [22], thus widening the population of patients that could possibly benefit from it. They are able to withstand the biochemical and biophysical factors typical of an injured joint [23]. Moreover, several studies have indicated that as compared to human articular chondrocytes, HNC proliferate faster and have a higher and more reproducible chondrogenic capacity, both in vitro and in an ectopic model in vivo [24]. Numerous studies have indicated that dedifferentiated HNC are also able to retain the capacity to generate hyaline-like tissues in vitro, with mechanical properties approaching those of native cartilage. In addition, it has been shown that HNC can respond in vitro to physical forces resembling joint loading with an improved composition and organization of the extracellular matrix [22].
Based on strong preclinical evidence in relevant large animal models, clinical use of HNC for articular cartilage repair has started, and the results of a phase 1 study (NCT01605201) have been recently published, showing safety, tolerability, and variable degrees of defect filling and development of repair tissue approaching the composition of native cartilage [25].
17.3 Pluripotent Stem Cells
17.3.1 Embryonic Stem Cells (ESCs)
From a multi-lineage differentiation and regenerative potential, ESCs represent the ideal cell source for tissue regeneration. Of course, several biological and ethical issues limited their clinical adoption, despite the significant potential.
ESC cultures were established for the first time in 1981 from mice [26] and in 1998 from human blastocysts [27]. ESCs have unlimited self-renewal capacity and pluripotency into any developmental lineage (ectoderm, mesoderm, and endoderm). Several papers have reported chondrogenesis of human ESC (hESC) by using variable combinations of biochemical (TGFβ1, BMP2, FGF2, PDGF-bb [28]) or biophysical stimuli (hypoxia [29] and mechanical compression [30]).
As mentioned before, the use of ESC is limited by serious ethical concerns, because, in order to obtain an ESC population, the embryo is destroyed in the process. In order to overcome this issue, Chung Y et. al. reported in 2006 a new method using single blastomeres, which does not require destruction of embryos [31]. However, concerns about immune rejection upon implantation, on the use itself of embryonic material [32], and on the risk of teratoma formation still exist [33]. The latter complication has been the object of several investigations, in order to minimize its risk. In particular, techniques such as prolonged differentiation [34], cell sorting and selection [35], and gene induction inducing selective apoptosis for undifferentiated cell have been evaluated [36].
Despite all these efforts, clinical trials using ESC have not been reported for cartilage repair yet and their successful application for nonlethal disease seems unlikely at the moment.
17.3.2 Induced Pluripotent Stem Cells (iPSCs)
iPSCs represent the forefront of cell-based strategies for regenerative medicine and are considered a promising solution for many incurable diseases. In 2006, Takahashi and Yamanaka reported a groundbreaking paper, demonstrating that differentiated cells can be reprogrammed to an embryonic-like state upon nuclear transfection of four key master regulator genes (Oct3/4, Sox2, c-Myc, Klf4) under SEC culture conditions. This innovative strategy allows, on the one hand, to overcome the ethical issues with the use of ESC and, on the other hand, to develop very potent cells to be used for regenerative medicine purposes and for drug screening [37]. The original, so-called, Yamanaka factors include c-Myc, which is known to have an oncogenetic activity, and are induced by a retroviral vector which can also cause host genome mutation. Therefore, more safe production methods, based on omitting c-Myc transfection (despite the lower iPSC generation efficiency [38]), on using of L-Myc in place of c-Myc [39], or on a virus-free induction, [40] have been evaluated.
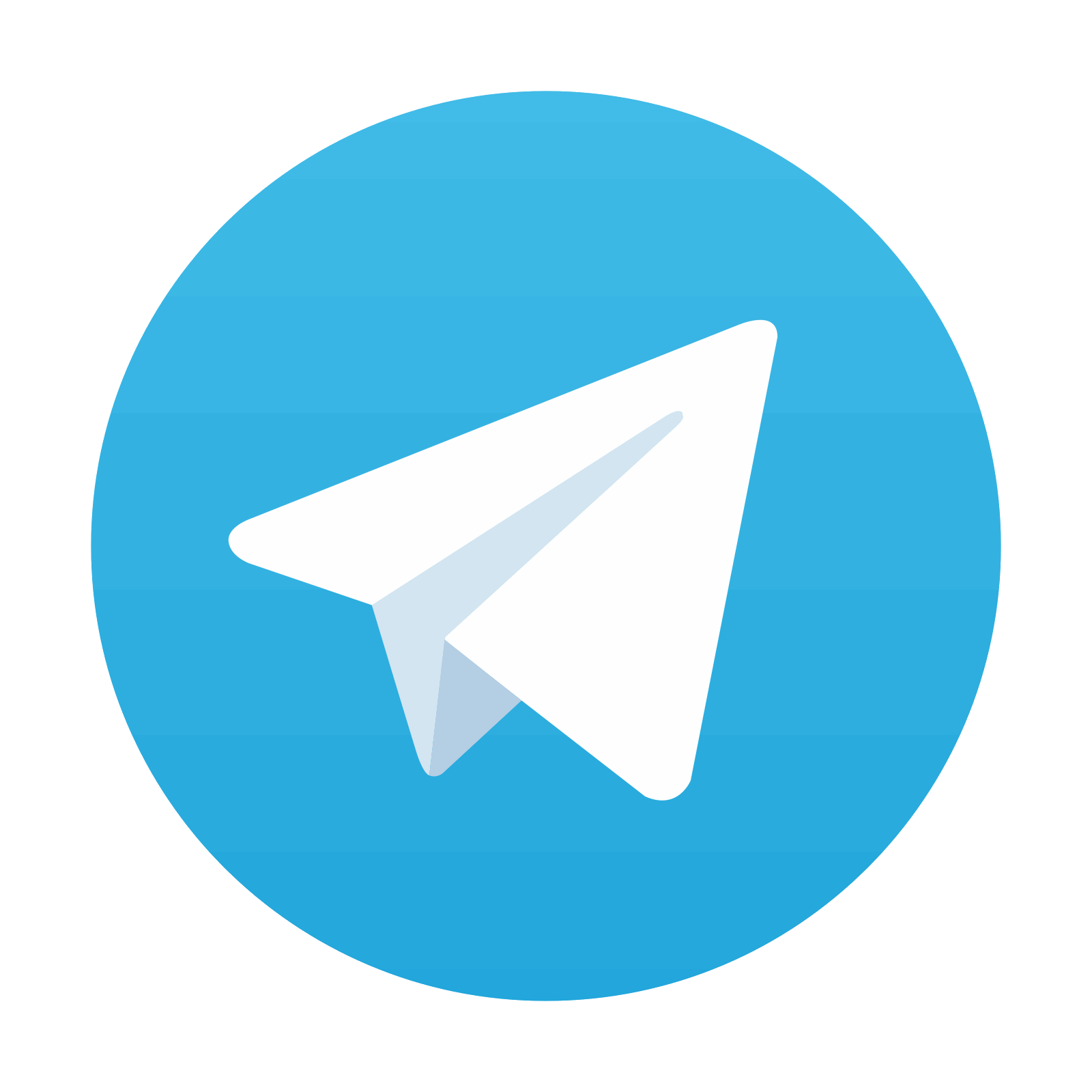
Stay updated, free articles. Join our Telegram channel

Full access? Get Clinical Tree
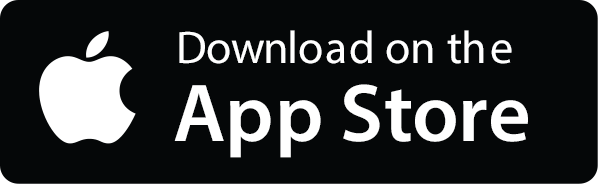
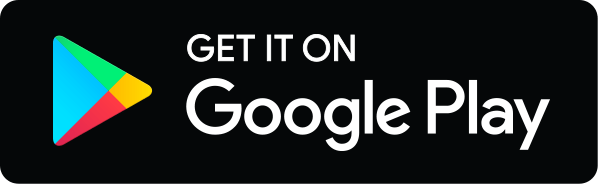