Vectors
Capability of transferring genes into nondividing cells
Maximum size of insert
Stability of transgene expression
Retro-/lentiviral vectors
No (yes for lentiviral vectors)
≤8 kb
Stable (random DNA insertion)
Adenoviral vectors
Yes
8 kb
Expression lost in 1–2 weeks in normal animals; expression can last weeks to months with immunosuppression
Herpes simplex viral vectors
Yes
>25 kb
Stable; maintained as episome
Adeno-associated viral vectors
Yes
<4.5 kb
Stable; unclear if DNA integrates in vivo
Nonviral vectors
No
4.3 kb
Short term; maintained as episome
14.4.1 Nonviral Gene Transfer Systems
Nonviral systems have been developed by complexing DNA to various factors such as cationic and anionic liposomes, non-liposomal lipids, peptides, DNA–ligand complexes, and others [9–14]. There are no side effects associated with their use, as nonviral systems avoid the risk of acquiring replication competence inherent to viral vectors and can be repeatedly administered. The issue of a lower efficacy is particularly critical for direct applications in vivo, so ex vivo strategies are normally necessary, by re-implantation of genetically modified cells to the location of interest.
14.4.2 Adenoviral Vectors
Adenoviral vectors were among the most employed gene vehicles especially for cartilage repair in preclinical models [15–21]. They possess high transduction efficiencies in a variety of cells and in vivo. However, adenoviral vectors are immunogenic and transgene expression is limited in time (1–2 weeks) as the transgenes are kept as episomal elements. Especially the problem of in vivo immunogenicity has been a major problem and consequently this vector class is not tested anymore in clinical gene therapy trials.
14.4.3 Retro-/Lentiviral Vectors
These vectors are capable to integrate in the genome of the target, allowing for the replication and maintenance of the transgene over extended periods of time. However, they have a restricted host range and cannot transduce nondividing cells. Ex vivo approaches are usually required with retro-/lentiviral vectors [22, 23] as they can be produced only at relatively medium titers and do not exhibit very high efficiencies of transduction.
14.4.4 Herpes Simplex Virus Vectors
Herpes simplex viral vectors (HSV) are large vectors that can deliver long transgenes in almost all known cell types, including nondividing cells. Although first-generation vectors induced high levels of cytotoxicity, recent work has demonstrated that second-generation HSV were less deleterious, in particular for cartilage repair [24]. One problem remains the transient nature of transgene expression mediated by this family of vectors.
14.4.5 Adeno-Associated Viral Vectors
The adeno-associated virus (AAV) is a nonpathogenic, replication-defective human parvovirus that has been manipulated to generate small recombinant (rAAV) vector particles by completely replacing the viral sequences by a transgene cassette, making them less immunogenic. rAAV are mostly maintained under stable episomal forms, allowing for long-term transgene expression (several months to years), avoiding the risk of insertional mutagenesis. rAAV can transduce both dividing and nondividing cells at relatively high efficiencies, favoring direct approaches in vivo. The problem of limited capacity of rAAV has been apprehended by taking advantage of the ability of the virus to form circular concatemers. As a result, rAAV became a currently preferred gene transfer tool [25–29].
14.5 Articular Cartilage Gene Therapy
Gene therapy approaches for articular cartilage chiefly concentrate on gene therapy for OA and for focal articular cartilage defects. Although there are principles of overexpression of transcription and growth factors are similar, the very differences between these two entities necessitate their separate assessment.
14.5.1 Gene Therapy Approaches for Focal Articular Cartilage Defects
Current reconstructive surgical treatments of focal articular cartilage defects comprise, among others, marrow stimulation techniques and autologous chondrocyte implantation (ACI). Particularly for marrow stimulation techniques, addition of a gene transfer approach appears very reasonable: Due to the nature of the cell types involved in the repair process, targeting the MSCs that originate from the subchondral bone space and that fill a lesion after marrow stimulation appears to be the most straightforward solution. Here, particularly rAAV vectors have shown benefits in organ cultures and in small and large animal models. Pilot experiments in small and large animal models using transduction with insulin-like growth factor (IGF-I), fibroblast growth factor (FGF-2), and TGF-β genes improved articular cartilage repair [30]. These gene vectors can also be delivered in conjunction with biomaterials. An interesting variation is the use of bone marrow containing transduced cells, which might further enhance bone marrow aspirate supported strategies.
ACI is useful for treating large chondral lesions. Here, a genetic modification of the implanted chondrocytes appears to be reasonable. Articular chondrocytes can be transduced or transfected with a variety of nonviral and viral methods. A long list of publications established both approaches, the most effective growth factor genes being FGF-2, IGF-I, BMP-2 and -7, together with the transcription factors SOX9 [30–32]. So far, no clinical studies have been undertaken to test this approach in patients.
14.5.2 Gene Therapy Approaches for OA
OA is the most prevalent chronic joint disease. The approach of restoring the damaged articular cartilage in the process of OA is much more complicated, largely due to the different nature of the disease which not only involves the cartilage, but also the subchondral bone and other tissues. However, gene-based approaches appear reasonable, especially for the very early stages of human OA, in which significant parts of the cartilage volume are still preserved [8]. Gene therapy aims at resulting in a controlled, site-specific, long-term presence to rebuild the OA cartilage. rAAV vectors are particularly suited to directly transfer gene sequences in human articular chondrocytes in situ, providing a potent tool to modulate the structure of OA cartilage. However, there are only few preclinical animal studies which have been performed at different models of OA. Of note, several gene therapy clinical trials have been carried out in patients with end-stage knee OA. Here, an intra-articular injection of human juvenile allogeneic chondrocytes overexpressing a cDNA encoding TGF-β1 via retroviral vectors was performed. A recent placebo-controlled randomized trial reported improved clinical scores. These important translational results provide sufficient reason to proceed with further clinical testing of gene transfer protocols for the treatment of OA [8].
14.6 Gene Therapy for Fracture Repair
Current surgical treatment strategies for disturbed bone regeneration, e.g., in nonunion fractures, mainly rely on the implantation of autologous bone grafts with or without the addition of bone anabolic agents such as rhBMP-2 or -7 [33]. Yet, these methods are limited by donor-site morbidity, restricted supply of autologous bone grafts, and specific side effects of the recombinant proteins as stated earlier [34–36], rendering this clinical field susceptible to novel approaches. While bone formation capacity is similar using in vivo or ex vivo strategies, the indirect use of genetically modified cells allows for a faster and more controlled bone formation [37]. Interestingly, in a “same-day approach,” stem cells may be transduced and implanted on the day of isolation without an expansion phase in vivo [38].
Fracture healing is a complex process including an inflammatory, chondrogenic, bone formation, and bone remodeling stage [39], with specific spatial and temporal gene activities appearing at different stages [40]. Therefore, the correct choice and timing is crucial for successful therapeutic gene delivery [33]. In order to stimulate bone formation by direct gene delivery in vivo, genes encoding for growth and differentiation factors such as BMPs, FGF-2, IGF-I, TGF-β, platelet-derived growth factor (PDGF), and vascular endothelial growth factor (VEGF) have been used with varying results [33]. The discovery of novel target genes involved in fracture healing such as the runt-related transcription factor 2 (Runx2) [41] could further promote this field. When ex vivo gene therapy is performed, genes like hypoxia-inducible factor 1 alpha, also known as HIF-1α [42], and osterix [43] are potential candidates for bone formation. The combination of several genes, such as VEGF and BMP-6 [44] or BMP-2 and BMP-7 [45], may also yield convincing results. The use of gene activated matrices consisting of synthetic scaffolds [46] or bone allografts [47], impregnated with plasmid DNA or coated with viral vectors encoding osteogenic factors, represents another promising approach [48]. Recently, attention has also been paid to microRNA and silencing RNA as therapeutic candidates [49].
In the future, gene therapy studies will have to be performed in translational large animal models [50] in order to assess the clinical applicability of this experimental approach and its superiority compared with established surgical measures such as autologous bone grafting.
14.7 Gene Therapy for Meniscal Repair
The menisci aid in transmitting forces for joint stability, nutrition, lubrication, and proprioception. Type-I collagen is the predominant collagen of the meniscal extracellular matrix [51]. The peripheral, vascularized part of a meniscus contains fibroblastic cells and type-I collagen with low proteoglycan contents [52]. The central, avascular part instead contains fibrochondrocytes and a higher proportion of type-II collagen and proteoglycans (water retention for viscoelasticity) and has a poor healing capacity [52–54]. Meniscal injuries (trauma, age-related degeneration) are very common issues in orthopedic surgery and sports medicine [55], accelerating the onset of OA [56]. Lesions in the peripheral zone may be sutured [57], while those in the central zone may be treated by arthroscopic partial meniscectomy. Yet, despite such clinical options, more effective treatments are needed to improve the outcomes of meniscal repair.
Gene therapy offers powerful tools to treat meniscal lesions based on the use of gene transfer vectors (nonviral, adenoviral, retro-/lentiviral, or rAAV vectors) capable of modifying directly cells within the lesions (in vivo approach) or following ex vivo re-administration to the site of injury [58]. Such approaches may be combined with the use of a biocompatible material adapted to support cell growth and/or differentiation (differentiated meniscal fibrochondrocytes versus progenitor cells). Candidate gene code for therapeutic factors that:
- 1.
stimulate cell proliferation/survival,
- 2.
target anabolic pathways, and/or
- 3.
inhibit catabolic and possible inflammatory pathways.
In vitro stimulation of proliferative activities in meniscal and progenitor cells (bone marrow) has been achieved by gene delivery of the IGF-I [59], FGF-2 [60, 61], and TGF-ββ without [62] or in a type-I collagen/glycosaminoglycan (GAG) matrix [63] using nonviral [59, 61], adenoviral [63], and rAAV vectors [60, 62]. Successful activation of anabolic pathways has been reported in meniscal and progenitor cells (bone marrow) by application of IGF-I or TGF-β genes without [62, 64] or with type-I collagen/GAG matrix [63] via adenoviral [63], retroviral [64], and rAAV vectors [62]. In vivo therapeutic gene transfer has been tested by implanting progenitor cells (bone marrow) modified with an IGF-I nonviral vector using alginate in goat meniscal lesions, leading to enhanced tissue repair [59].
14.8 Ligament Gene Therapy
Ligament tears due to various etiologies are one of the most devastating and frequent soft tissue injuries in the field of sports medicine. To date, restoration of these weak self-healing and limited regenerative tissues remains a persistent clinical challenge [65–67]. Genetic modification of ligament grafts, MSCs, fibroblasts, and myoblasts prior to implantation by means of an efficient vector system carrying mitogenic and proliferative growth factors or therapeutic genes (such as FGF-2, IGF-I, PDGF-B, BMP-12, and decorin) offers an appealing and successful approach to make considerable improvements upon the outcome of ligament injuries [65, 66, 68–74].
Studies on transgene expression and effects of the adeno- and retroviral systems delivering lacZ reporter genes to the anterior cruciate ligament (ACL) and the medial collateral ligament (MCL) indicated that both vectors can effectively and continuously transfer genes into the ACL and the MCL, without inflammatory responses [69, 75], showing promise in the treatment of ligament damages using gene transfer. To investigate the potential biomechanisms of FGF-2 on the healing profiles of ligaments, Madry et al. [68] applied rAAV vector carrying FGF-2 to transduce primary human ACL fibroblasts in vitro and experimentally injured human ACL explants in situ and found efficient, stable FGF-2 expression via rAAV vector facilitating the healing process of such human ACL lesions by activating key cellular and metabolic processes, which is consistent with the effects of IGF-I via rAAV gene transfer upon human ACL fibroblasts in vitro [73]. Nakamura et al. [71] injected the PDGF-B gene directly into the injured patellar ligament of rat and observed an enhanced and accelerated matrix synthesis after the PDGF-B gene transfer. In addition, when antisense oligodeoxynucleotides (ODNs) to decorin were combined with a hemagglutinating virus of Japan-conjugated liposome system and directly injected into MCL scars, the delivery of antisense decorin ODNs significantly decreased the expression of decorin and promoted the healing of early rabbit ligament scars with increased collagen fibrillogenesis in vivo, illustrating the potent and therapeutic efficiency of decorin for ligament healing via gene transfer [70, 74].
Application of genetically modified fibroblasts and myoblasts is another potential strategy to enhance ligament healing [69]. Fibroblasts and myoblasts transduced with adenoviral vectors containing lacZ gene and injected into rabbit ACLs could be detected in the ligament tissues and the synovial tissues surrounding the ACL, indicating that a direct application and survival of genetically engineered cells for ligament healing is possible [69].
14.9 Tendon Gene Therapy
Tendons are elastic cords serving to anchor muscles to the bone and enable muscles to move limbs. Restoration of lacerated tendons caused by injuries and degenerative diseases is challenged by restrictive adhesions of inflammatory stiffness and fibrotic scarring which leads to compromises of tendon functional gliding and limitations in range of motion (ROM) [76–79]. Due to their intrinsic limited potential for self-healing, damaged tendons have little capability to restore their original structures and functions [77]. Currently available therapeutic options in the clinics include tendon autografts and allografts transplantation. However, such procedures do not allow reproducing the original gliding tendon structure [77]. Genetically modified grafts or bone marrow-derived MSCs carrying tenogenic factors [such as growth and differentiation factor 5 (GDF-5) or BMP-14, TGF-β1, basic FGF-2 and BMP-2] prior to implantation to sites of injury might be a promising and efficient technique to overcome such critical issues [79–88].
Lou et al. [85] established a chicken tendon and tendon sheath model and transduced this model with a recombinant adenoviral vector containing the lacZ gene, establishing that a functional exogenous gene can be effectively transferred into a tendon. In a mouse model of allograft tendon reconstruction, Basile et al. found that rAAV-GDF-5-loaded freeze-dried tendon allografts facilitated the scratch closure rate of monolayer fibroblasts and wound healing in vitro and significantly improved nearly 65% of the normal ROM in vivo [80]. Subsequently, Hasslund et al. [82] analyzed the flexor tendon healing and adhesions on the same mouse model after freeze-dried allografts pretreated with rAAV delivering GDF-5 protein and demonstrated a dose-dependent retention in vitro and equivalent effects on the joint flexion function in vivo. However, the lower dose of GDF-5 protein indicated more potent effects on suppressing adhesions without adversely affecting the strength of the tendon repair, which is consistent with the observations of adenoviral GDF-5 transfer in a rat Achilles tendon healing model [81, 86]. In another in vivo experiment, Tang et al. [87] injected AAV-2 carrying a FGF-2 gene into the disrupted flexor tendon model and observed that biomechanical strength and tendon healing were increased and adhesions and the energy required for flexion were reduced after FGF-2 gene transfer.
More recently, MSCs have been successfully induced into tenocytes through genetic-modified differentiation techniques [83, 84]. Hou et al. [84] implanted adenoviral TGF-β1-transduced MSCs into injured Achilles tendons in rabbits and found that such MSCs significantly promoted the remodeling of collagen matrix and fiber bundles. Similarly, the healing process of the Achilles tendon was significantly accelerated and enhanced after MSCs grafts transduced with adenovirus harboring TGF-β1 were applied [83].
Conclusion
Following the initial enthusiasm in the past decade of the last century, continuous basic research has now laid a strong scientific base to translate gene therapy approaches in the clinical situation. Especially preclinical evaluations do support the concept of using gene therapy approaches to enhance the repair of musculoskeletal lesions, provided that combined efforts between scientists, clinicians, industry, and regulatory organizations will be ongoing to bring these strategies to the clinics. Of particular note is the completed phase I and II and the ongoing phase III double-blinded randomized controlled clinical trials for knee OA gene therapy. Application of gene therapy to OA and other nonlife threatening musculoskeletal diseases requires a better understanding of the mechanisms of musculoskeletal tissue repair to avoid undesired local and distant effects. The main challenge for such clinical musculoskeletal applications thus remains ensuring safety while guaranteeing effectiveness. From the clinical data available to date, there is no evidence that undesired events might occur and the recent OA trials have an excellent safety record. More large animal and safety studies together with more randomized controlled clinical trials will identify the best strategies in which gene therapy may complement the current reconstructive therapies to treat injuries and chronic diseases of the musculoskeletal system.
References
1.
Lo KW, Ulery BD, Ashe KM, Laurencin CT. Studies of bone morphogenetic protein-based surgical repair. Adv Drug Deliv Rev. 2012;64(12):1277–91. doi:10.1016/j.addr.2012.03.014. pii:S0169-409X(12)00110-X.
2.
Hustedt JW, Blizzard DJ. The controversy surrounding bone morphogenetic proteins in the spine: a review of current research. Yale J Biol Med. 2014;87(4):549–61.PubMedPubMedCentral
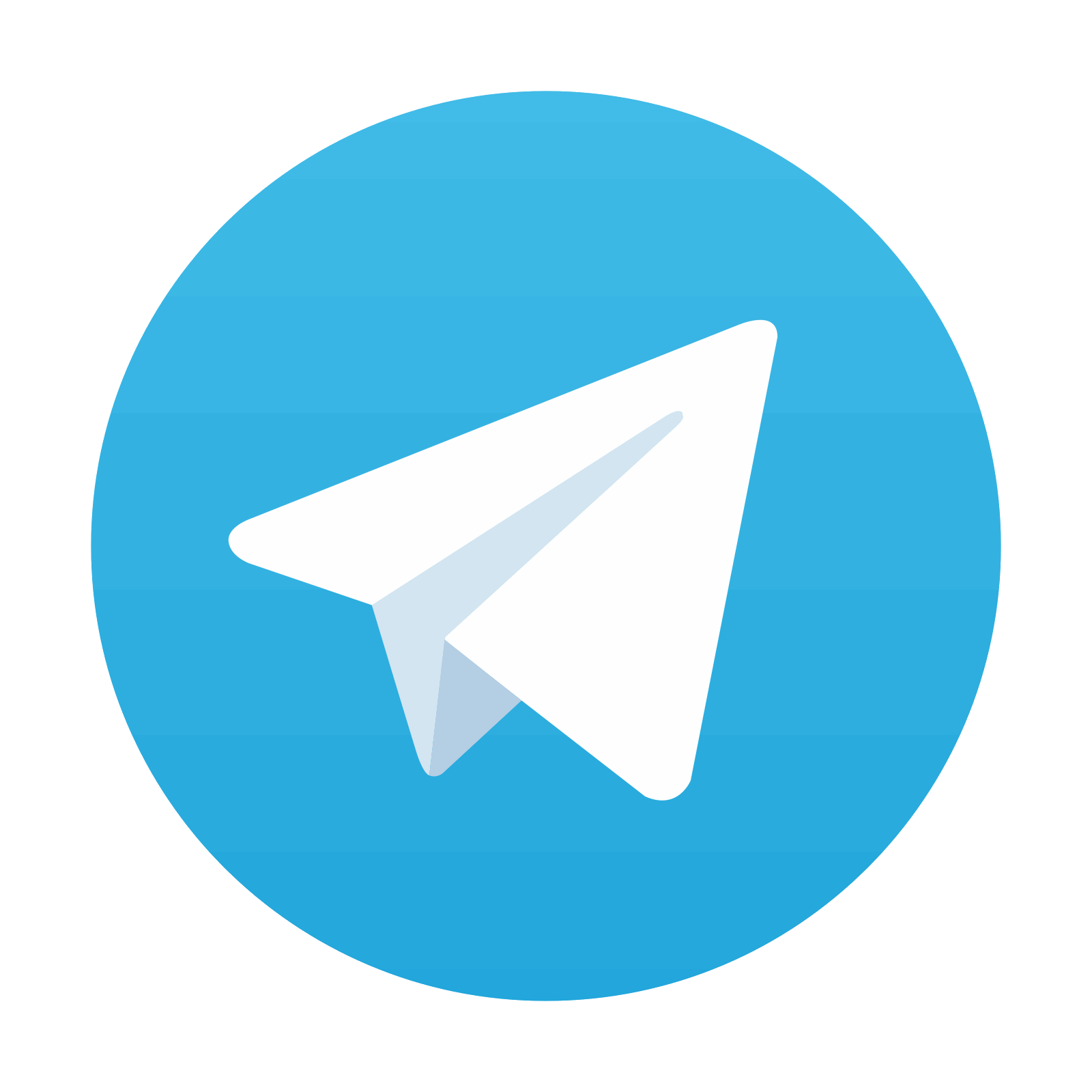
Stay updated, free articles. Join our Telegram channel

Full access? Get Clinical Tree
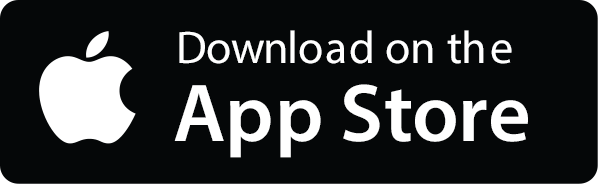
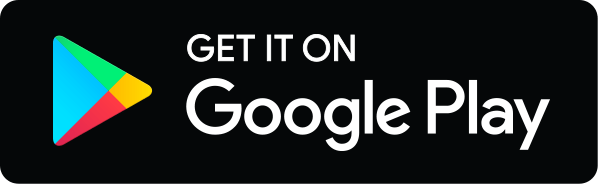