Fig. 50.1
Articular cartilage structure. Articular cartilage is organized in four distinct regions: (1) the gliding zone (or superficial tangential), composed of thin collagen fibrils in tangential array and associated with a high concentration of decorin and a low concentration of aggrecan, (2) the transitional zone (or middle) with radial bundles of thicker collagen fibrils, (3) the radial zone (or deep) in which the collagen bundles are thickest and are arranged in a radial fashion, and (4) the calcified cartilage zone, located immediately below the tidemark and above the subchondral bone. The interterritorial cartilage matrix bestows tensile strength and consists primarily of type II collagen fibrils with type XI within the fibril and type IX integrated in the fibril surface with the non-collagen domain projecting outward, permitting association with other matrix components and retention of proteoglycans
In a healthy joint, normal alignment, stability provided by ligaments, and smooth motion provided by menisci and articular cartilage maintain a noninflammatory microenvironment that allows chondrocytes to reside in a quiescent state with very little turnover of the ECM. Interestingly, physiological loading may protect against cartilage loss by inhibiting TAK1 (TGF-β-activated kinase 1) phosphorylation [8] as well as inhibiting IKKβ (IƙB kinase-beta) activity in the canonical NF-ƙB cascade and attenuating NF-κB transcriptional activity [9]. When physiological factors are affected by trauma or overload, the homeostatic joint environment is disrupted, and the chondrocytes become activated with increased proliferation, production of matrix-degrading enzymes, cytokines, and cytokine receptors. In the case of chronic overload, the consequence could be an increased chondrocyte activity as mechano- and osmo-sensors, as osteocytes in bone alter cell metabolism in response to physical cues [10, 11]. In the case of trauma, the activated phenotype is likely an injury response driven by inflammation. Despite the classical view of osteoarthritis (OA) as a noninflammatory arthritis, recent literature supports the concept of OA as an inflammation-driven process where cartilage destruction is maintained and perpetuated by inflammatory mediators [10, 12, 13] (Table 50.1).
Table 50.1
Summary of biologic effects and molecules involved in inflamed joints
Stimulatory molecules | Inhibitory molecules | References | |
---|---|---|---|
ECM breakdown | ADAMTS, MMPs | TIMPs, IL-4, IL-10 | |
Recruitment of inflammatory cells | IL-8, VEGF, MCP-1 | NSAIDs, IL-4, IL-10 | |
Synovial cell activation | IL-1β, TNF- α | IL-4, IL-10 | |
Synthesis of ECM components | IGF-1, BMPs, TGF-β1 | IL-1β, TNF-α, IL-6 | |
Cell proliferation, cell survival | FGF-2, PDGF | IL-1β, TNF-α | [25] |
Cell differentiation | SOX9, TGF-β1 | IL-1β, TNF-α |
IL-1β and TNF-α are the main pro-inflammatory cytokines produced by activated chondrocytes [12]. They have a dual action: the upregulation of degradative proteases (starting from ADAMTS-5 and MMP3) and the synthesis by chondrocytes and synovial cells of other inflammatory mediators. The result is an autocatalytic process established and maintained in an autocrine/paracrine manner. Other pathways, as prostaglandin E2, are involved in the establishment of a catabolic environment, increasing the complexity of the problem [27]. In OA, degradative enzymes are produced primarily by chondrocytes, but the episodic intra-articular inflammation with synovitis indicates that the synovia may also be a source of cytokines and cartilage-degrading proteinases [28, 29]. In addition, the cartilage and bone are tightly interlinked with a cellular and molecular communication through the WNT and BMP signaling pathways [30, 31]. Consequently, when chondrocytes acquire the activated phenotype, enhanced matrix remodeling occurs in the articular cartilage and in the subchondral bone, ultimately leading to damage the articular surface, to subchondral bone sclerosis [31, 32] and osteophyte formation (Fig. 50.2).


Fig. 50.2
Articular cartilage destruction due to mechanical loading and biologic factors. ADAMTS a disintegrin and metalloproteinase with thrombospondin-1 domains, C/EBP CCAAT enhancer-binding protein, ESE1 epithelial-specific ETS, ETS E26 transformation specific, GADD45β growth arrest and DNA damage 45 beta, HIF-1α hypoxia-inducible factor-1-alpha, NF-κB nuclear factor-kappa-B, PA plasminogen activator, TIMPs tissue inhibitors of metalloproteinases. (Modified from Goldring MB, Marcu KB. Cartilage homeostasis in health and rheumatic diseases. Arthritis Res Ther 11(3), 224, 2009)
50.2 The Reparative Potential Rising from the Subchondral Bone: Microfractures
For these reasons, the well-known problem of the poor cartilage spontaneous healing potential is worsened by the generation of an inflammatory microenvironment by the interlinked intra-articular structures. However, the network between joint tissues is the starter point for reparation, too. In fact, the subchondral bone is in continuity/contiguity with the underlying bone, and its vascular system allows for the migration of mesenchymal progenitor cells from the bottom of the lesion and of chondrocytes from the margins of the adjacent cartilage [33]. These are the theoretical bases of the microfracture (MF) technique, which was introduced by Pride in the 1950s under the name of drilling and spread rapidly after the work of Steadman in 1998 [34]. However, in the case of an injury, the fine equilibrium between anabolic and catabolic cytokines [4] is disrupted, and the imbalance is not beneficial to cartilage repair, as it is in the bone, but typically results in a flawed regenerative process, which resembles endochondral ossification and leads to chondrocyte hypertrophy and death, cartilage calcification, and scar fibrous healing. In fact, while the role of IL-1β and TNF-α in the initiation of the regenerative process has long been known, especially for bone tissue [7], different experimental settings demonstrated the detrimental effect of the exposure of IL-1β to articular cartilage [14, 35, 36]. The limited response of articular cartilage together with the multipotent potential of the bone explains the prevalence of the bone over cartilage after bone marrow stimulation techniques such as MF [37–39] and the formation of a fibrous repair tissue that might not be able to preserve the articular cartilage function for the long term.
MF is a feasible, cost-effective, and simple first-line option but only when performed in young patients with small, single lesions and low postoperative demands. Deterioration of the repair tissue frequently begins between 2 and 5 years posttreatment, and degenerative changes are seen at long-term follow-up, regardless of lesion size [40]. Older athletes with large and multiple lesions typically display higher failure rates [41]. The poorer results in older patients could be explained by inflammation and gene expression. The former enhances endochondral ossification by human bone marrow-derived mesenchymal stem cell [42–44] and is likely present in older patients who have higher IL-1β, MMP-13, and oxidative stress levels [45]. The latter is similar in aged chondrocytes and in OA chondrocytes, suggesting an intrinsic lower healing potential of aged cartilage, independently from the inflammatory state [26].
Hyaluronic acid (HA) and platelet-rich plasma (PRP) have been tested as adjuvant therapies for MF with encouraging results [46–51]; nevertheless, a recent meta-analysis showed that when OA degenerative changes are established, viscosupplementation with HA is associated with a small and clinically irrelevant benefit [52], and HA alone may not be sufficient to restore joint environment in these patients. Besides, given the wide variability in PRP composition, it is difficult to define properly its dual anti-inflammatory and trophic effect and additional studies should be promoted.
50.3 Regenerative Strategies: Cellular Therapies
As far as researchers try to improve MF reparative tissue, it is still mechanically and biologically far from native articular cartilage. To bridge this gap, they focused on the cellular component, essential for the recovery and maintenance of the complex tissue structure, and they developed new treatment strategies. The main field of application of this paradigm is the autologous chondrocyte implantation, but, in the last few years, new perspectives (i.e., mesenchymal stem cells) are springing up.
50.3.1 Autologous Chondrocyte Implantation (ACI) Procedures
Since Peterson and Brittberg first introduced ACI technique in the clinical practice in 1994 [53], it has become popular and widespread with more than 20,000 patients treated. Yet their efficacy and cost-effectiveness are still debated [54, 55]. The technique is two-step, expensive, and complicated with short-term clinical results comparable to those of MF, but potentially has the capacity to develop a more durable hyaline-like and functional repair tissue thanks to the chondrogenic cellular component implanted. A series of clinical trials have been reported with improvement of symptoms at medium- to long-term follow-up [54], but recently published results of the longest follow-up investigation (i.e., 14–15 years) comparing MF and ACI confirmed the absence of significant difference in clinical outcomes and radiographic signs of OA between the two groups [56]. It is important to underline that more than half of the patients had radiographic evidence of OA as determined by the Kellgren and Lawrence scale. Patients who had undergone prior surgery and patients with a history of symptoms of more than 2–3 years’ duration frequently have worse results at 5-year follow-up when compared to those with traumatic/acute lesions [57–59]. These findings highlight how an “activated environment” could potentially lead to failure in healthy tissue regeneration, even when differentiated cells are used. Consistent with what was described for the microfracture procedure, it may be crucial to develop strategies to restore the proper balance between anabolism and catabolism before surgery and during the long process of graft maturation. A further intriguing application of this concept is the use of intra-articular PRP to establish a regenerative microenvironment to improve results of ACI, similar to that reported for the MF procedure. A crucial step forward would be represented by the identification of the most suitable PRP preparation to be used as adjuvant for cartilage repair procedure [60, 61]. Interestingly, P-PRP (a pure platelet concentrate without leukocytes with a lower amount of platelets) appears to have more anabolic and anti-inflammatory properties than L-PRP (with high concentration of both platelets and leukocytes) [60, 61] highlighting the need for studies focused on the identification of the optimal ratio of cellular and molecular components of PRP according to the different requirements. Moreover, a recent systematic review showed no evidence supporting the use of intra-articular injections of PRP for preventing OA progression. This demonstrates that further knowledge is needed for the identification of the proper use and the ideal formulation of PRP in the OA patients.
50.3.2 A New Promising Cellular Therapy: Mesenchymal Stem Cells
In most recent years, a new cellular source has been introduced as orthobiologic approach to cartilage treatment: the mesenchymal stem cells (MSC), also known as multipotent mesenchymal stromal cells. They are a non-hematopoietic adult stem cell population, which is present in various tissues (e.g., bone marrow, adipose tissue, synovial membrane), which has the ability to differentiate into mesenchymal lineages including chondrogenic, and, therefore, is a promising source in regenerative medicine. It was initially believed that engraftment and differentiation of MSC would lead to neotissue formation and tissue repair [62]. However, more recently, it has been shown that MSC can stimulate tissue repair by the secretion of potent paracrine factors, and only a limited amount, if any, of MSC actually engraft and differentiate in vivo [63]. According to recent literature, the beneficial effects of MSC are due to the release of a cocktail of trophic and immunomodulatory factors, rather than to an active participation in tissue regeneration, thus working as “medicinal signaling cells” [64]. This twofold activity has the potential to address the complexity of joint disorders, which require both immunomodulation and tissue regeneration.
Chondrocyte implantation presents some disadvantages such as a two-stage surgical procedure that may cause further cartilage damage and degeneration [53, 65, 66] and the chondrocyte dedifferentiation during culture that might result in fibrocartilage rather than hyaline cartilage [65, 67]. MSC, on the other hand, are promising, as they would eliminate the need for ex vivo chondrocyte expansion, which is necessary for the ACI procedure. Additionally, from the patient, from the treating physician, and from an economic perspective, a single-stage non-cultured cell-based therapy would represent a great advantage [68].
As a source of MSC, bone marrow aspirate concentrate (BMAC) has been tested. Gobbi et al. reported a satisfactory clinical outcome in patients with grade 4 cartilage lesions of the knee who received one-step BMAC implantation with a hyaluronan-based scaffold [69]. Interestingly, this proved to be mainly affected by lesion size and number and not by age. In addition, it allows to address the older than 45-year-old population, with results comparable to younger patients. Alternatively, intra-articular bone marrow-derived MSC injection following isolation and in vitro expansion showed positive clinical and MRI outcomes [70] as an adjuvant treatment following high tibial osteotomy (HTO) for early unicompartmental OA and genu varum, further supporting the role of MSC in the restoration of the articular surface.
As the concentration of active cells present in the bone marrow was found to be particularly low, other cell sources were tested for the same purpose and a higher concentration of MSC was founded in adipose tissue, the so-called adipose tissue-derived MSC (AD-MSC). However, to date, few clinical studies have examined this option. The injection of stromal vascular fraction containing adipose-derived MSC as an adjuvant of MF for osteochondral lesions of talus showed encouraging clinical and MRI results even in patients with poor prognostic factors [71]. Another study highlighted the benefit of injecting a high dose of in vitro expanded AD-MSC (1 × 108 cells) in OA knees with no adverse events, improvement of function, and, foremost, histological regeneration of hyaline-like articular cartilage [68].
Acknowledging their limited power, these pioneering studies support the potential of intra-articular MSC injection and highlight the need for a high number of MSC to obtain a clinical improvement, encouraging further studies involving cohorts of patients [72].
50.4 Shifting the Paradigm: Tissue Therapy
Despite the general clinical improvement, current strategies are far from generating a long-lasting repair tissue that matches native cartilage in terms of tissue quality and mechanical performance [73].
A tissue therapy [74] based on a more mature tissue with high cell density and a more abundant cartilaginous ECM could potentially have a better chance of coping with the stresses of an inflammatory environment and therefore regenerating hyaline tissue. This is suggested by different basic studies, which demonstrated that cytokine synthesis and response to IL-1β by human articular chondrocytes are modulated by their differentiation stage [14, 15, 36, 75], and, in particular, more developed engineered tissues are more able to preserve the deposited cartilaginous matrix and to release lower amounts of MMP and higher amounts of anabolic factors [15]. These studies prompt the idea that more mature cartilage constructs might represent a better graft for cartilage regeneration as it might be more resistant to pro-inflammatory chemokines and more effective in recruiting/committing cells involved in tissue repair processes. In MF and in ACI techniques, cells are exposed to the joint environment without the protection typically provided by the cartilaginous ECM from inflammatory and mechanical insults.
A promising proof of principle of tissue therapy was recently reported [74] showing successful alar lobule cartilage regeneration with restoration of function and aesthetic satisfaction. Despite the notable difference in terms of environment and function with the articular cartilage, it is important to highlight the mechanical resiliency of the engineered graft, which is a valuable feature also for articular cartilage repair.
A further interesting advancement, which stems from this clinical experience and from several experimental basic science studies [14, 76–78], is represented by the use of autologous nasal chondrocytes as cell source for engineering a cartilaginous graft. Nasal chondrocytes demonstrated capacity of self-renewal and environmental plasticity [77]. They showed positive response to dynamic compression mimicking joint loading [76], higher chondrogenic capacity than articular chondrocytes (from matched human donors) [14], more efficient recover than articular chondrocytes from exposure to IL-1β (from matched human donor) [14], and a greater survival and integration in the joint environment together with the acquisition of a mesodermal phenotype [77]. Moreover, the use of nasal chondrocytes for tissue therapies has insignificant morbidity for their harvest from the nasal septum, which represents a crucial improvement compared to the use of articular chondrocytes. Taken together, these features render nasal chondrocytes a superior cell source for cartilage tissue engineering. Consistently, a phase I clinical trial for the treatment of traumatic cartilage defects is currently ongoing with an approved extension to 25 patients (http://www.clinicaltrials.gov/ Identifier:NCT01605210), and it represents the first clinical application of “tissue therapy” using autologous nasal chondrocytes, as opposed to the standard “cell therapy” procedure of delivering articular chondrocytes by a scaffold material. Promising results about the first ten patients have recently been published. For each patient, the nasal-derived engineered tissues were stable through handling with forceps and could be secured in the injured joints. No adverse reactions were recorded and self-assessed clinical scores for pain, knee function, and quality of life were improved significantly from before surgery to 24 months after surgery. Radiological assessments indicated variable degrees of defect filling and development of repair tissue approaching the composition of native cartilage [79]. This encouraging data confirmed the value of tissue therapy strategy and the need for future clinical experimentation before offering this approach to chronic/early OA patients. Based on the experimental and preclinical studies performed [74, 77], this strategy has the potential to overcome the classical limitations of cartilage regeneration such as kissing lesions, larger defects, and older patients [72]. A randomized phase 2 clinical trial has been planned to address these issues.
50.5 Could Allogeneic Therapies Be an Option?
The standard for cell therapies for musculoskeletal condition has typically been autologous cells, while allogeneic cells were mainly used for onco-hematological disorders [80]. However, following the first studies that demonstrated the safety of this approach [81], clinical trials involving allogeneic cells increased in number [82–84]. A growing body of literature proving the low immunogenicity of allogeneic MSC [85] supports their use, which has the potential to tackle the classic limitations of autologous cell therapies such as interindividual variability and the need for additional surgery for cell harvest. To ensure sufficient cells, expanded allogeneic MSC could be used as on off-the-shelf cell product [86]. This shift could improve the cost-effectiveness and the sustainability of the cell-based therapies.
De Windt et al. [86] reported positive results for allogeneic MSC implantation for single-stage cartilage repair. They demonstrated that the proof of concept, in which rapidly isolated chondrons were recycled from debrided cartilage instead of harvested from a non-load-bearing site of the knee, combined with allogeneic human bone marrow MSC, is feasible, stimulates reproducible tissue regeneration, and provides clinical improvement. This study was the first showing allogeneic MSC as safe and effective in stimulating cartilage regeneration in the knee when combined with autologous chondrons. The fact that 1 year after surgery no stem cell DNA could be traced in the regenerative tissue may confirm the recent view on MSC as cellular moderators, which stimulate autologous repair through paracrine mechanisms.
Allogeneic human umbilical cord blood-derived MSC (hUCB-MSC) has been tested as a source of MSC, too. Park et al. [87] showed the safety and efficacy of articular cartilage regeneration by a stem cell-based medicinal product (a composite of culture-expanded allogeneic hUCB-MSC and hyaluronic acid hydrogel [Cartistem®]) in patients with Kellgren-Lawrence grade 3 OA and International Cartilage Repair Society (ICRS) grade 4 cartilage defects. Seven participants were enrolled. Maturing repair tissue was observed at the 12-week arthroscopic evaluation. The VAS and IKDC scores were improved at 24 weeks. The improved clinical outcomes were stable over 7 years of follow-up. The histological findings at 1 year showed hyaline-like cartilage. MRI at 3 years showed persistence of the regenerated cartilage. Only five mild to moderate treatment-emergent adverse events were observed. There were no cases of osteogenesis or tumor genesis over 7 years.
On the other hand, the increased immunogenicity and upregulation of cell surface antigenic antigens of MSC as they differentiate [85] create the need of assessing the feasibility of allogeneic cell therapies in the latest intriguing orthobiologic field of research: tissue therapy. The function of Fas ligand and induction of immune privilege in tissue-engineered cartilage have been recently studied, and the subsequent suppression of uncontrolled inflammation and macrophage activity resulted in increased formation of cartilage [88]. Although performed in a mouse model, with limited translational value, this work sheds light on a fundamental aspect of cartilage regeneration and paves the way to a broader application of regenerative therapies based on allogeneic cells. This could be achieved by treating the tissue-engineered cartilage with granulocyte colony-stimulating factor (G-CSF) [88] or by preventing IL-6 downregulation [89]. However, the pro-inflammatory activity of IL-6, which inhibits ECM synthesis, might limit its use to improve tolerance of the implanted graft.
50.6 Future Perspectives: Targeted Therapies
Another valuable option could be the development of more targeted therapies, capable of interacting with specific signaling pathways to alter the joint balance toward the trophic side, both modulating inflammation and enhancing cartilage anabolism.
IL-4 and IL-10 are modulatory cytokines and capable of reestablishing the joint homeostasis through inhibition of pro-inflammatory cytokines like IL-1β [16, 21, 22]. Additionally, IL-4 and IL-10 are known for their anti-inflammatory properties, and their combination is suggested to be beneficial [90], also preventing the potential harmful effects of IL-4 alone [91]. Strategies based on human recombinant IL-10 and IL-4 with a longer half-life, on autologous/allogeneic cells modified to overexpress IL-10/IL-4, or on smart scaffolds capable of controlled release of IL-10/IL-4 may be used alone or as a support to regenerative therapies. It has also been demonstrated that IL-4 increases IGF-1 expression, suggesting a cross talk between the two pathways. In addition, it possesses a synergistic effect with IGF-1 with greater type II collagen upregulation, inflammatory cytokine downregulation, and nitric oxide level decrease [92]. A recent study demonstrated that IL-4 is downregulated in human OA cartilage, compared to healthy cartilage, and directly involved in the downregulation of IL-1β-induced MMP13 [93]. These in vitro studies prompt further in vivo orthotopic studies to demonstrate the potential of these cytokines for cartilage repair in the most challenging scenarios.
A further interesting approach involved the modulation of the PGE2 signal through a specific E2 receptor agonist [5, 94]. In a first study, authors demonstrated successful cartilage tissue regeneration in a rabbit orthotopic model through microsphere-based release at 12-week follow-up. In a second study, the same authors reported a transient prevention of OA changes upon anterior cruciate ligament transection and subsequent intra-articular injection of the E2 receptor agonist [5, 94]. These studies highlighted the need for delivery systems capable of a long-term controlled release to harness the potential of a more targeted modulation of the inflammatory environment.
In a recently published paper, Johnson et al. [95] provided new insights for the control of chondrogenesis introducing kartogenin, a small molecule that promotes chondrocyte differentiation, shows chondroprotective effect in vitro, is efficacious in two OA animal models, and may ultimately lead to a stem cell-targeted therapy for OA. Most importantly, kartogenin selectively interacts with RUNX1, thus promoting hyaline cartilage formation, and does not activate RUNX2 signaling, which would lead to an undesired hypertrophic differentiation.
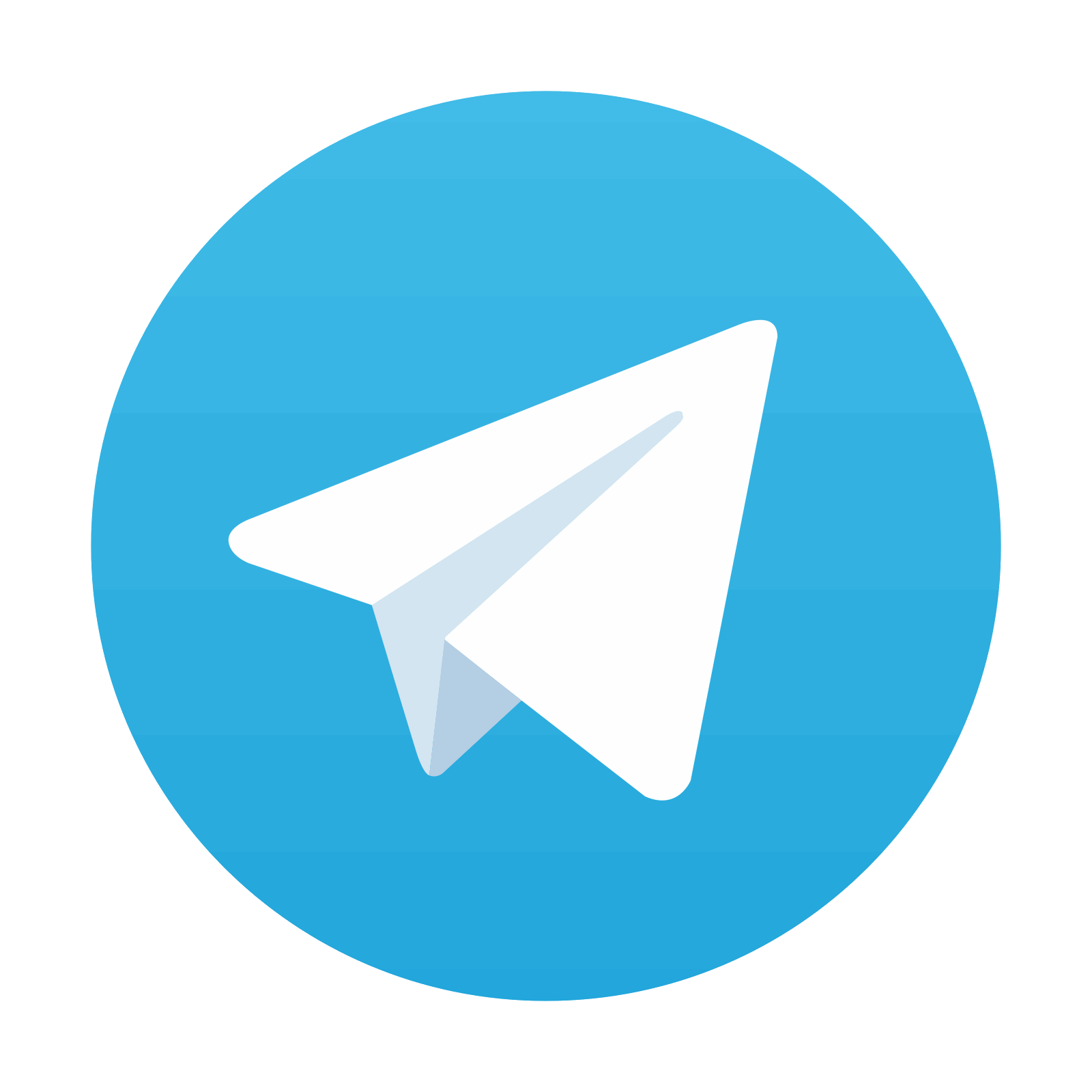
Stay updated, free articles. Join our Telegram channel

Full access? Get Clinical Tree
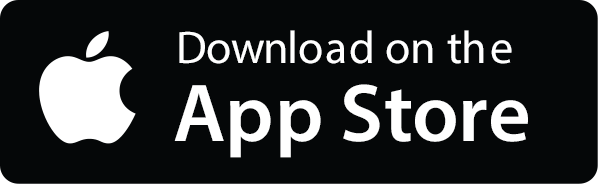
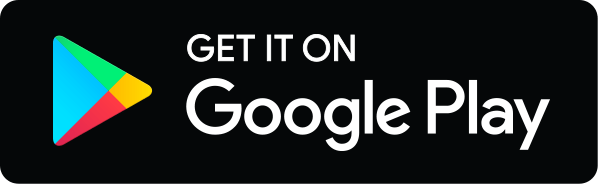