CHAPTER 34 Surgical Management of Rheumatoid Arthritis
Rheumatoid arthritis is a chronic, progressive, systemic disease with widespread involvement of connective tissues and primarily synovial joints. Women are affected two to three times more often than men with most cases manifesting in the 4th or 5th decades, although patients may be affected at any age. More recent improvements in medical management have correlated with a decrease in hospitalizations for severe rheumatoid disease; however, one study found that although admission for treatment of several medical complications of rheumatoid arthritis decreased significantly from 1983-2001, the rate of admission for cervical spine surgery in this population remained unchanged.1 The cervical spine is involved in 25% to 90% of patients with rheumatoid arthritis, making it the most commonly affected site after the metatarsophalangeal and metacarpophalangeal joints.2 In contrast to rheumatoid arthritis of the thoracic and lumbar spine, cervical spine involvement carries significant risks of spinal cord and medullary and vertebral artery compromise and sudden death.3,4
Numerous patients with cervical instability may be asymptomatic or may have symptoms indistinguishable from symptoms in patients without instability. Neva and colleagues5 reviewed 154 patients with rheumatoid arthritis who were on a waiting list for various orthopaedic procedures at a Finnish hospital. Overall, 44% of patients had radiographic evidence of cervical subluxation or prior cervical fusion. When excluding patients with previous fusions, these investigators noted that 69% of patients with radiographic evidence of subluxations reported neck pain compared with 65% of patients without subluxation. Similarly, rates of occipital, temporal, retro-orbital, and upper extremity radicular pain were not significantly different in patients with or without cervical instability. Many patients with significant cervical involvement may be overlooked by the treating physician because of the lack of clear neurologic deficits on physical examination. The physician must be cognizant of the natural history, pathophysiology, clinical presentation, radiologic findings, and treatment options to avoid the grave consequences of rheumatoid disease of the cervical spine.
Historical Perspective
There is some evidence that rheumatoid arthritis was described in the 17th and 18th centuries.6,7 More concrete evidence points to a period around 1800, according to a description by Landré-Beauvais, a French medical student, in his doctoral thesis.8 The description of rheumatoid arthritis of the cervical spine occurred much later, with a report in 1890 by Garrod.9
Interest increased in the 1950s and 1960s with reports describing cervical instability.10–13 Articles on the surgical treatment of cervical instability in patients with rheumatoid arthritis followed in the 1960s and 1970s.14–18 For the most part, C1-2 fusion with Gallie-type19 wiring and graft techniques were used early on. Brooks and Jenkins,20 Wertheim and Bohlman,21 and Clark and colleagues22 subsequently described modified wiring techniques. Other types of C1-2 fixation emerged later with the use of the Halifax interlaminar clamp,23 the Magerl transarticular screw technique,24–28 and posterior C1 lateral mass–C2 pedicle screw fixation.29
Foerster30 originally described occipitocervical procedures in 1927. Kahn and Yglesias31 were the first to add iliac crest bone graft to occipitocervical fusion in 1935. Hamblen16 later described the combined use of wires and iliac crest graft for occipitocervical fusion in four patients with rheumatoid arthritis and cervical instability in 1967. In a 1976 report, Brattström and Granholm14 modified the wiring technique for occipitocervical fusion by adding methyl methacrylate. Modifications of occipitocervical fusion techniques continued in the 1980s with the emergence of the looped rod with iliac crest bone graft, as described by Ransford 32 and Flint 33 and their colleagues and later occipitocervical plating, as described by Grob34,35 and Smith36 and their colleagues. Current use of plate or rod fixation extends to the subaxial spine, especially with fixation of the lateral masses described by Magerl and Roy-Camille.25–27
Pathophysiology
The inflammatory process in the cervical spine mirrors the inflammatory process in other sites of the body and consists predominantly of T lymphocytes, macrophages, and plasma cells in a hypervascular synoviocyte pannus.37,38 The inflammatory reaction has a predilection for synovial joints, and the multiple synovial joints39 of the cervical spine (facets, uncovertebral, atlanto-occipital, atlantodens) are particularly affected. In contrast to the thoracic and lumbar spine, the cervical spine relies heavily on ligaments and joint congruency for stability and so is at greater risk for instability. With significant inflammation, pannus formation and erosion of the joint and capsular structures can occur. Progressive instability can result, manifesting as atlantoaxial (C1-2) instability, subaxial subluxation, cranial settling (also known as basilar invagination or atlantoaxial impaction), or a combination thereof. Rheumatoid discitis may also occur at the discovertebral junctions with noninfectious erosion of the endplates.3
Atlantoaxial instability is the most common instability pattern in the rheumatoid cervical spine and may be the result of the development of periodontoid pannus and progressive inflammatory destruction of the upper cervical spine.39–41 Pannus formation localizes around the synovial joint formed between the transverse ligament, the posterior arch of the atlas, and the base of the odontoid. The soft tissue may compress the spinal cord and often distends and erodes the surrounding periodontoid-ligamentous structures (alar, apical, and transverse ligaments), the odontoid process (dens), and the lateral articular masses between C1 and C2.42 Erosion of the dens may lead to the development of occult, atraumatic odontoid fractures.43 Subsequent static or dynamic instability or subluxation occurs in 50% to 70% of patients.18,44 Most often, the subluxation is anterior (70%), but lateral, posterior, and rotational subluxations can also occur.45,46 Anterior subluxation of 0 to 3 mm is normal in adults, subluxation of 3 to 6 mm suggests instability with disruption of the transverse ligament, and subluxation of 9 mm or more suggests disruption of the entire periodontoid-ligamentous and capsular structures with gross instability and is a clear indication for surgery.18,47,48
The combination of periodontoid pannus buildup and instability may cause spinal cord and root impingement leading to myeloradiculopathy and sudden death. Delamarter and Bohlman49 conducted postmortem studies suggesting that paralysis can be due to mechanical neural compression, vascular impairment of the neural structures, or both. Henderson and colleagues50 reported that diffuse axonal injury with or without frank necrosis may irreversibly affect the spinal cord owing to mechanical damage. These findings may partly explain the smaller diameter and cross-sectional area of the spinal cord found in patients with severe disease.51 Some evidence suggests that instability promotes worsening periodontoid pannus formation and that stabilization reverses the buildup.40
Posterior atlantoaxial subluxation is rare and should raise the possibility of an anterior arch defect of the atlas or erosion or fracture of the odontoid.48 Patients usually present with myelopathy and posterior kinking of the cord without radiographic compression.52 Lateral subluxation is defined as more than 2 mm of lateral displacement of the C1-2 lateral masses and occurs in 21% of atlantoaxial subluxations.48 Lateral subluxations occur more commonly in patients with spinal cord compression than patients without compression and are often accompanied by rotational subluxation.53
Subaxial subluxation is the second most common instability pattern and results from destruction of the facet joints, interspinous ligaments, and discovertebral joints.44,54 These pathologic changes can lead to longitudinal collapse, bony erosion, soft tissue hypertrophy, and sagittal plane instability along multiple spinal segments, causing a “stepladder”-type pattern of deformity. Concurrent spondylodiscitis may occur with pain, neural compression, and instability.
Natural History
It is difficult to study the natural history of rheumatoid arthritis because patients are usually treated at some stage of the disease and it would be unethical to do otherwise. From studies on rheumatoid arthritis, it seems consistent that the inflammatory processes in the cervical spine begin early after the onset of rheumatoid arthritis and progress along with peripheral involvement. Atlantoaxial instability may be detected within 2 to 10 years of disease onset in most patients,55 and there is a strong correlation between cervical spine subluxation and peripheral erosions of the hands and feet.56 Approximately 10% of patients with cervical spine involvement eventually require surgery.57
The natural history of rheumatoid arthritis of the spine without surgical intervention, especially in patients with myelopathy, seems to be progressive disability and risk of sudden death. In one study of 21 patients treated medically, 76% showed deterioration at an average of 6 years of follow-up. All patients in the study became bedridden within 3 years of developing myelopathy, and all died within 7 years with one third dying suddenly for unknown reasons.58 Risk factors for progression include mutilating articular disease, history of high-dose corticosteroid use, high seropositivity, rheumatoid subcutaneous nodules, vasculitis, and male gender. Other potential but unproven risk factors for cervical involvement include high C-reactive protein level and HLA-Dw2 or HLA-B27 positivity.59,60 As the disease progresses, pain, neurologic deficits, and sudden death are the primary risks.
Clinical Presentation
Neck pain is the most common symptom in patients with cervical spine involvement. Peripheral erosive changes similarly occur along the apophyseal joints and surrounding soft tissues and may be a source of pain. Cervical instability may cause secondary impingement of the posterior rami of the lesser and greater occipital nerves, which may lead to occipital headaches. Pain in the suboccipital region generally suggests atlantoaxial pathology or cranial settling. Middle or lower cervical pain should suggest subaxial subluxation. These are not hard and fast rules because pain localization in rheumatoid cervical disease may be poor. Subaxial involvement may also lead to painful neck deformity with loss of sagittal plane supporting structures, which may later become fixed deformities owing to postinflammatory ankylosis. Patients may complain of a “clunking” sensation in the neck with neck motion. This sensation is most common in patients with C1-2 instability, owing to spontaneous reduction of the subluxation with neck extension (known as the Sharp-Purser test).61
Progressive instability leads to decreased effective canal diameter and brainstem compression. Myelopathy and vertebrobasilar dysfunction may result because of mechanical and ischemic damage to the white and gray matter evidenced on histologic specimens by long tract demyelinization, lateral column necrosis, and focal gliosis.49 According to Bell,62 C1-2 instability may lead to a phenomenon termed cruciate paralysis, characterized by upper motor neuron dysfunction of the upper extremities with paresis and paralysis and normal lower extremities similar to a mild central cord syndrome after trauma. Pathologically, there is selective injury to the decussating corticospinal tracts of the upper extremities at the level of the cervicomedullary junction with sparing of the uncrossed lower extremity pyramidal and extrapyramidal tracts.
Additional neurovascular changes may occur because of vertebral artery occlusion with decreased flow into the posterior inferior cerebellar artery and cephalad brainstem circulation. Without adequate collateral blood flow, these patients may develop Wallenberg syndrome or lateral medullary infarction. These clinical syndromes are characterized by ipsilateral cranial nerve palsies (cranial nerves V, IX, X, and XI), cerebellar ataxia, Horner syndrome (ptosis, miosis, anhidrosis, and enophthalmos), facial pain, and contralateral loss of pain and temperature sensation.63 Rarely, patients may develop quadriplegia, quadriplegia with facial muscle paralysis (locked-in syndrome),64 and sudden death.38,58
In contrast to pain symptoms, neurologic signs are less straightforward. Myelopathy is progressive but may not be evident by loss of fine motor control, gait imbalance, or global numbness of the hands, but rather by the slow onset of deteriorating independence and becoming wheelchair bound. In many instances, hand deformities mask motor deficits in the upper extremities, and the patients’ deteriorating ambulatory status may be attributed to large joint involvement rather than myelopathy. Patients must be assessed keeping their global disease in mind. Progression of myelopathy, especially early disease, may be misinterpreted as progression of peripheral disease. A high index of suspicion is necessary in these patients to detect early myelopathy because patients do poorly without early surgical intervention.38,58
The Ranawat grading system may provide some useful clinical information in assessing patients with neurologic deficits (Table 34–1).65 This system classifies pain as none (grade 0), mild (1), moderate (2), or severe (3). Neurologic function falls into three classes: Class I has no neurologic deficit; class II has subjective weakness, dysesthesia, and hyperreflexia; and class III has objective weakness and long tract signs. This last class is subdivided further into class IIIA, in which patients are ambulatory, and class IIIB, in which patients have quadriparesis and are unable to walk. Casey and colleagues66 reported that only 25% of 55 Ranawat class IIIB patients who underwent surgical decompression and stabilization had a favorable outcome. The mortality rate was 13% after 30 days and 60% by 4 years.
TABLE 34–1 Ranawat Criteria for Pain and Neural Assessment
Pain Assessment | |
Grade 0 | None |
Grade 1 | Mild, intermittent, requiring only aspirin analgesia |
Grade 2 | Moderate; cervical collar needed |
Grade 3 | Severe; pain not relieved by either aspirin or collar |
Neural Assessment | |
Class I | No neural deficit |
Class II | Subjective weakness with hyperreflexia and dysesthesias |
Class IIIA | Objective findings of paresis and long tract signs, but walking possible |
Class IIIB | Quadriparesis with resultant inability to walk or feed oneself |
Adapted from Ranawat CS, O’Leary P, Pellici P, et al: Cervical spine fusion in rheumatoid arthritis. J Bone Joint Surg Am 61:1003-1010, 1979.
Radiologic Evaluation
Historically, the AADI was used to screen patients who required surgical stabilization for atlantoaxial instability based on an AADI greater than 9 mm, with AADI 0 to 6 mm suggesting instability and AADI 0 to 3 mm being normal.48 AADI is limited by the occasional difficulty of obtaining accurate measurements in some patients because of erosive changes that distort the normal anatomic landmarks and because it has not been correlated clinically with the mass effect of retrodental pannus that may compress the spinal cord. Boden and colleagues67 showed that PADI may be more reliable in predicting neurologic outcome because it is a more accurate indicator of the space available for the cord. Patients with a PADI of at least 14 mm were more likely to have neurologic recovery after surgical stabilization, whereas patients with a PADI less than 10 mm had no neurologic recovery. These observations can also be explained by the anatomy of the spinal cord at the C1 level because the dura requires an average space of 1 mm on the anterior and posterior sides, the cerebrospinal fluid requires 2 mm, and the cord requires 10 mm for a total space of 14 mm.68
The open-mouth view is useful to identify lateral subluxation, defined as more than 2 mm of lateral displacement of C1-2 lateral masses, which occurs more commonly in patients with spinal cord compression than in patients without compression.48,53 Erosive changes of the dens and C1-2 articulations may also be visualized. Taniguchi and colleagues69 defined the atlantodental lateral shift (ADLS) as a means of assessing lateral atlantoaxial instability. Using dynamic open-mouth odontoid views (taken in maximal left and right lateral bending), ADLS is calculated by dividing the distance from the center of the dens to the medial edge of the C1 lateral mass (in the direction of lateral bending) by the distance between the medial borders of the bilateral C1 lateral masses and is expressed as a percentage. With this method, these investigators noted that in patients with rheumatoid arthritis, the ADLS averaged 14.8% compared with 6.1% in control patients. Among the subgroup of patients with rheumatoid arthritis and increased AADI, the ADLS averaged 20.6% versus 12.7% in patients without anterior atlantoaxial instability.
Posterior subluxation, although rare, may be seen on lateral radiographs of the cervical spine and should be suspected in patients with an absent or fractured odontoid process.52 The AADI decreases with worsening cranial settling as the arch of the atlas approaches the wider base of the odontoid process and is a potential source of mistaken radiographic improvement.
Cranial settling carries the greatest risk of neurologic deficits and sudden death, especially when superimposed on atlantoaxial instability. As a consequence, several measurement techniques have been used with varying sensitivity and specificity, based on lateral plain radiographs of the upper cervical spine (Fig. 34–1).70 Most of these techniques assess the relationship between the tip of the odontoid, the hard palate, and the base of the skull. Traditionally, McGregor line (drawn from the posterosuperior tip of the hard palate to the caudad base of the occiput) has been widely used for its simplicity. Cranial settling occurs when the tip of the dens is more than 4.5 mm above this line.
Erosive changes in the odontoid anatomy may make it difficult to analyze many of the measurement techniques, so Redlund-Johnell and Pettersson71 described a technique that measured the vertical line from the midpoint of the caudad margin of C2 to McGregor line. Cranial settling occurs when the distance is less than 34 mm in men or less than 29 mm in women. Ranawat and colleagues65 described a similar technique in which the distance along the odontoid was measured from the C2 pedicle to the transverse axis of the ring of C1. This technique avoided problems with changes in odontoid anatomy and identifying the bony landmarks at the base of the skull and hard palate. Cranial settling was positive if the distance was less than 15 mm in men or less than 13 mm in women. Clark and colleagues22 defined the “station of the atlas” (Fig. 34–2), which indicates the position of the anterior ring of C1 to parts of the body of the axis divided into thirds. Normally, the atlas is adjacent to the upper third (station I) of the axis. Riew and colleagues70 noted that none of the current radiographic measurements alone had a sensitivity, specificity, or negative or positive predictive value greater than 90% and recommended the combination of the Clark station, Redlund-Johnell criteria, and Ranawat criteria with sensitivity and negative predictive values of 94% and 91%.
Subaxial subluxation is the second most common instability pattern and occurs secondary to inflammatory destruction of the apophyseal and discovertebral joints and supporting soft tissues. White and Panjabi72 defined radiographic cervical instability as 3.5 mm or more of vertebral translation and greater than 11 degrees of angular changes between adjacent motion segments, although their study is more representative of acute conditions. The space available for the cord should also be considered in these patients because critical stenosis may be present with or without instability. Boden and colleagues67 correlated anatomic measurements with neurologic findings and reported that a sagittal diameter of less than 14 mm was considered critically stenotic in the rheumatoid subaxial spine. This is in contrast to 13 mm in cervical spondylotic patients who do not have pannus in the canal.
Computed tomography (CT), especially with intrathecal contrast medium, is a valuable addition to plain radiographs in delineating the bony anatomy and identifying cord compression and cranial settling. The degree of medullary compression on CT scans has been shown to correlate with myelopathy.40 Static or dynamic magnetic resonance imaging (MRI) is the best option, however, to assess the soft tissues and to look for the presence of periodontoid pannus (Fig. 34–3) and spinal cord or brainstem compression.73–75 MRI is also useful for postoperative evaluation of the periodontoid pannus, which has been shown to decrease after stabilization.40 Myelopathic signs correlate with MRI findings75 of a cervicomedullary angle of less than 135 degrees (normal 135 to 175 degrees). This angle measures the intersection of vertical lines drawn along the anterior surface of the brainstem and the cord on sagittal MRI.
Nonsurgical Treatment
Although life expectancy is lower in patients with rheumatoid arthritis, many are living longer with their disease because of more aggressive medical therapy that has slowed disease progression with fewer side effects.59 Only a few of these patients eventually need surgical stabilization. Patients with early cervical disease and intermittent pain without radiographic instability or myelopathy can be managed with a soft cervical orthosis or trials of physical therapy. Medication options include nonsteroidal anti-inflammatory drugs (NSAIDs); oral steroids (prednisone); and disease-modifying antirheumatic drugs (DMARDs) such as methotrexate, gold, sulfasalazine, hydroxychloroquine, penicillamine, and azathioprine.
In recent years, biologic agents such as tumor necrosis factor (TNF)-α and interleukin-1 antagonists have become increasingly available. The addition of antiresorptive osteopo-rosis therapy may improve vertebral bone strength in patients with rheumatoid arthritis.76 Patients should be followed closely for neurologic or radiographic changes. Cervical collars provide support, warmth, some pain relief, and a feeling of stability; however, rigid collars are often poorly tolerated, especially in patients with temporomandibular disease, dental problems, and skin sensitivity, and may have further detrimental effects by blocking spontaneous reduction of anterior atlantoaxial subluxation in extension.
Surgical Management
Indications
Patients with any one or more of the instability patterns described earlier (atlantoaxial instability, cranial settling, subaxial subluxation) associated with or without pain, myelopathy, or neurologic deficits should be considered for early surgery. As noted previously, radiographic indicators for surgery include AADI of 9 mm or more; PADI of less than 14 mm; mobile subaxial subluxation greater than 3.5 mm; cord compression or space available for the cord of less than 14 mm; and cranial settling measured most accurately by the combination of Clark station, Redlund-Johnell criteria, and Ranawat criteria.70 CT myelography and MRI showing cord compression or a cervicomedullary angle of less than 135 degrees are suggestive of impending neurologic deficits and should be weighed heavily as an indication for surgery within the context of the remaining clinical picture. Patients with headaches in the distribution of the greater or lesser occipital nerve are likely to have atlantoaxial instability and may need C1-2 arthrodesis for pain relief.
Timing of surgical intervention is crucial. In a retrospective review of 110 patients who underwent cervical spine fusion for rheumatoid arthritis, only 3 of 55 patients (5.5%) with early C1-2 fusion for isolated atlantoaxial instability developed subaxial subluxation requiring surgery an average of 9 years later. This review contrasted with a 36% incidence of recurrent cervical instability at a mean of 2.6 years in patients who initially underwent occiput-C3 fusion for atlantoaxial instability combined with cranial settling. The investigators recommended early surgery before the development of cranial settling to decrease the risks of recurrent instability.39 In a series of 28 patients, Schmitt-Sody and colleagues77 reported that 7 of 10 patients who were Ranawat class II improved to class I after surgery, whereas only 1 of 11 class III patients improved (class IIIA to class II), and 2 patients deteriorated (class IIIA to class IIIB) postoperatively. Similarly, these authors recommended early surgical stabilization before the development of neurologic symptoms.
Preoperative Assessment
The goals of surgery should be relief of pain, spinal alignment, decompression to relieve neurologic deficits where necessary, and stabilization of the involved motion segments. Thorough preoperative assessment is a prerequisite to good outcome in these often fragile patients. The quality of the bone stock, the presence of irreducible subluxations, and the medical condition of the patients should guide the preoperative decision-making process, including the potential need for awake fiberoptic nasal or endotracheal intubation.78
Patients with severe basilar invagination should be considered for preoperative skeletal traction using a halo ring. The ring can be incorporated into a halo vest after surgery. Constant preoperative traction can improve alignment, neurologic symptoms, and pain. Traction may be required for 3 to 7 days or longer,52,79,80 and some authors have used a halo wheelchair to allow the patient to be more mobile and avoid secondary problems such as decubitus ulcers and infection from prolonged bed rest.52,79,80 Gentle traction along the midline longitudinal axis with approximately 7 to 12 lb while avoiding hyperflexion or hyperextension is recommended. Patients are monitored with frequent neurologic examinations, and plain radiographs should be obtained to avoid overdistraction during the process. The authors do not routinely use preoperative traction in atlantoaxial instability or subaxial subluxation. Many of these instabilities that moved little on preoperative flexion-extension views are better reduced when the patient is under general anesthesia positioned with the head holder.
When in the operating room, obtaining baseline neurophysiologic data including somatosensory evoked potentials and motor evoked potentials before positioning a myelopathic patient may help prevent cord injury during or after positioning of the head and neck. Finally, the surgical approach should be determined by the underlying pathology and its location, in part analogous to the approach for compressive lesions in patients with cervical spondylosis.81,82
Perioperative Management of Rheumatoid Medication
Because of the risk of increased bleeding time and potentially increased intraoperative blood loss, NSAIDs should be discontinued 3 to 5 half-lives before surgery.83 In addition, NSAIDs have been previously shown to inhibit bone healing and so should be held as long as possible postoperatively so as not to impair formation of a spinal fusion.
Corticosteroids may produce immunosuppression, which can adversely affect wound and bone healing. Sudden stoppage of long-term corticosteroid therapy may lead to adrenal insufficiency, necessitating the continued use of corticosteroids perioperatively and potentially requiring the use of stress dosing. Generally, perioperative management of corticosteroids depends on the long-term dosage and the potential degree of surgical stress. Most spinal procedures for these patients would be considered highly stressful, and patients on long-term moderate-dose to high-dose regimens (>20 mg/day of prednisone) should receive stress dose steroids on the day of surgery with rapid tapering over 1 to 2 days to their usual long-term dosage.83,84
Continued use of methotrexate in the perioperative period has not been shown to increase infection rates.85 It may affect bone healing, however86; this has not been studied in a spinal fusion model. Although withholding methotrexate may increase the likelihood of a flare of rheumatoid symptoms, the authors routinely discontinue its use postoperatively and recommend that patients remain off of methotrexate for 6 to 8 weeks if possible.
Few studies exist to direct perioperative management of the newer biologic agents such as TNF-α and interleukin-1 antagonists. Because of their strong immunoregulatory effects, these agents may predispose patients for opportunistic infections.83,84 In a retrospective review, Giles and colleagues87 reported that 10 of 91 (11%) patients with rheumatoid arthritis who underwent an orthopaedic surgical procedure developed early, major postoperative infection (septic arthritis, osteomyelitis, deep wound infection). Of the 10 patients with infection, 7 (70%) were receiving TNF-α inhibitor therapy during the perioperative period. There are no data on the effects of these agents on bone fusion. Current recommendations are to discontinue biologic agents preoperatively (at the end of the dosing cycle), and restart 10 to 14 days postoperatively.
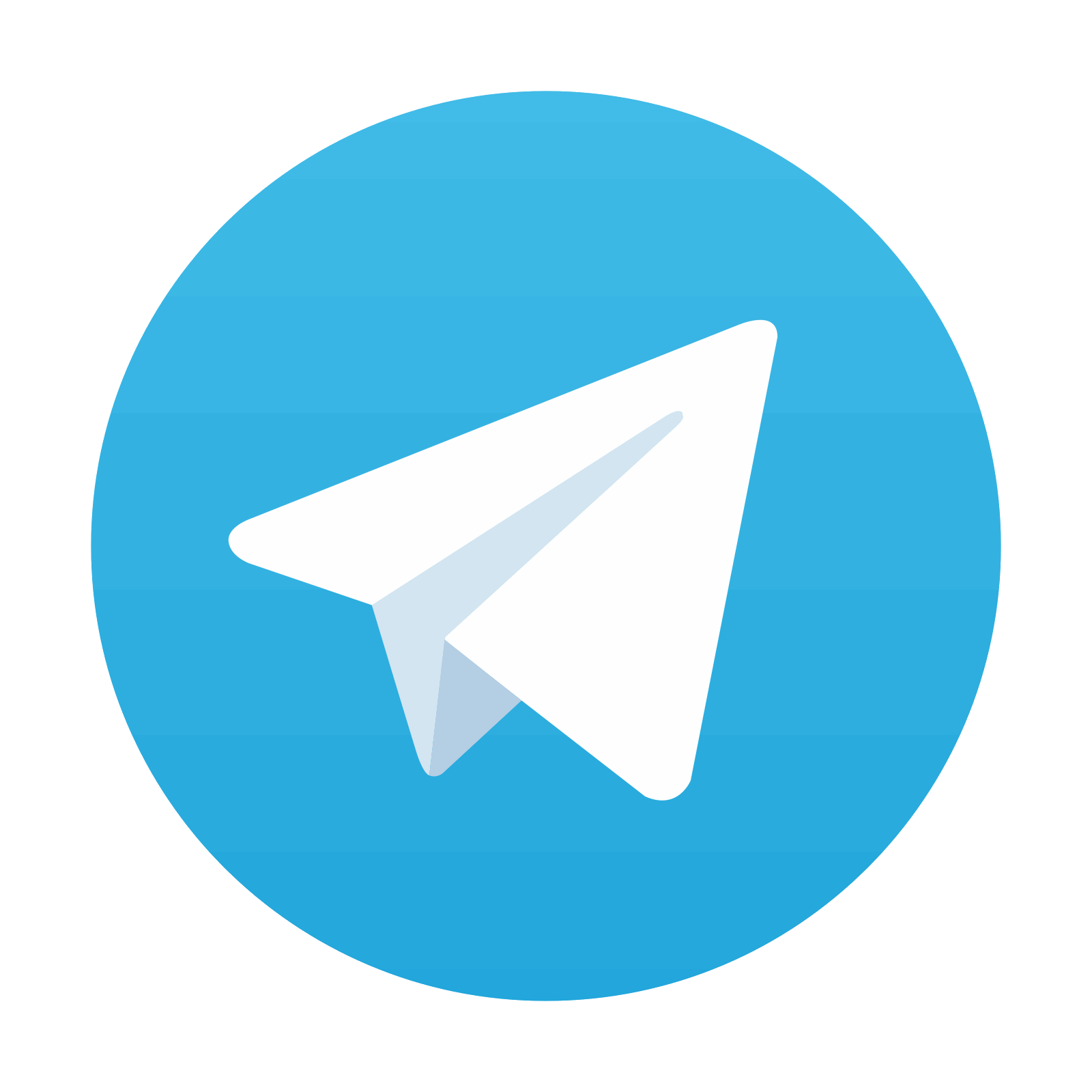
Stay updated, free articles. Join our Telegram channel

Full access? Get Clinical Tree
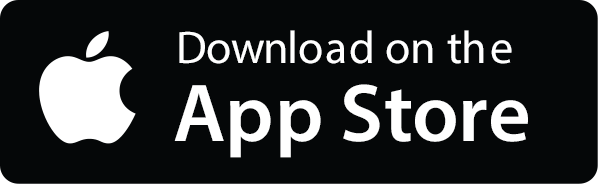
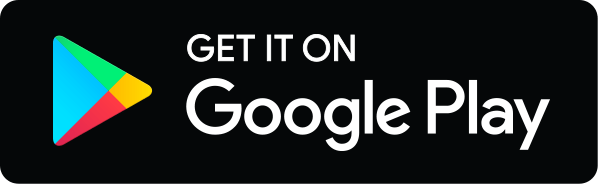