CHAPTER 49 Posterior Lumbar Interbody Fusion
The technique of posterior lumbar interbody fusion (PLIF) has become an important part of the modern spine surgeon’s armamentarium. This was not always so; for nearly half a century after the introduction of this technique, it was performed routinely by only a handful of surgeons. Most surgeons condemned the technique as unnecessary, technically difficult, or even dangerous.1,2 Controversy persists regarding the safety of PLIF compared with other approaches to the intervertebral space and regarding the benefit that PLIF adds to the outcome of surgical treatment for various spinal pathologies.
Historical Perspective
Attempts to identify the first surgeon to perform an interbody fusion from a posterior approach are muddled by several reports appearing in the published literature beginning in 1944,3–5 but no one disputes that credit for initially developing the techniques and key principles of the operation as it is practiced today belongs to Cloward,6 who emphasized the importance of a wide exposure of the spinal canal to minimize nerve root injuries, the use of structural graft to prevent intervertebral collapse, and the complete removal of nuclear material from the disc space and replacement with bone to promote fusion. Cloward was widely criticized for his operation, probably as much because he advocated that it be included as part of the routine surgical treatment for all lumbar disc herniations as for the frequency of poor outcomes and complications when it was attempted by other surgeons. A few surgeons embraced Cloward’s operation, and several large surgical series were published in the literature reporting good outcomes and high fusion rates.7–10 Widespread interest in and acceptance of PLIF as a valuable technique did not occur, however, until the introduction of pedicle screw instrumentation.
With the early generation of pedicle instrumentation, screw fracture was a common occurrence. Fracture especially occurred if a dramatic change in spinal alignment was achieved, such as in the reduction of a high-grade spondylolisthesis. Even without large changes in alignment, screw fracture occurred commonly in overweight patients or when the disc space was distracted.11 In those cases, the mechanical loads that the instrumentation was subjected to exceeded the fatigue properties of the screws. Surgeons, in particular Steffee and Sitkowski,12 recognized that a structural graft placed in the disc space would divert some of the load, decreasing the forces on the posterior instrumentation, and reduce the frequency of instrumentation failure. Steffee and Sitkowski12 reported their experiences with reduction of high-grade spondylolisthesis. In each of the first three patients treated with pedicle screws, the instrumentation failed, and alignment was lost. In the next 11 patients, an interbody graft was placed. All of these patients developed solid fusions with no loss of alignment. With PLIF, the load-sharing anterior column support could be added to protect the pedicle screws without the need for a separate anterior incision (Fig. 49–1).
Another development that favored the adoption of PLIF was the invention of the interbody fusion cage by Brantigan and the titanium mesh cage by Harms. Their designs eliminated the need to harvest a structural graft from the iliac crest, which was a major source of morbidity previously associated with the PLIF procedure. The cage provided the structure,13 and the osteogenic potential for fusion came from cancellous bone packed into the cage, which could be obtained from the iliac crest more easily than a structural graft, with less injury to the patient. Before the invention of the cages, it was possible to avoid the morbidity associated with graft harvest by using allograft bone, as advocated by Cloward.14,15 Concerns about availability, disease transmission, healing potential, delayed healing, and wide variation in the structural integrity of available grafts all contributed to the limited enthusiasm for the use of allograft bone for interbody fusion.16,17 The availability of cages overcame another common objection to the PLIF operation.
The availability of pedicle screw instrumentation and intervertebral cages contributed to progressively greater acceptance of the operation first pioneered by Cloward. Better alignment and higher fusion rates are routinely achieved using these devices. The training of surgeons and marketing of these devices by their manufacturers have also contributed to wider adoption of PLIF in surgical practice. Not all of these devices have proven to be successful, however. Cylindric threaded fusion cages enjoyed brief popularity as PLIF devices that did not require supplementary fixation, but mediocre results and high complication rates associated with their use resulted in their virtual disappearance as a posterior spinal implant.18 The clinical experience with threaded implants and the study of the relevant biomechanics have helped surgeons understand the PLIF operation better, however, and develop the operation that is currently performed. Although more recent advances, such as the development of the transforaminal and direct lateral approaches to the disc space, have decreased the frequency with which PLIF is performed, PLIF remains an important and powerful tool for spinal surgeons.
Indications for Interbody Fusion
Some surgeons believe that an interbody fusion is indicated whenever a lumbar fusion is done. They argue that the intervertebral space is biologically and mechanically superior for fusion compared with the intertransverse plane because of the larger surface area of the highly vascular bony endplate and because the interbody bone graft is subject to compressive forces. In addition, they criticize the large amount of muscle damage that occurs from exposure of the spine for posterolateral fusion. Despite these theoretical advantages, it is difficult to show clinical superiority of interbody fusions over posterolateral fusions for most lumbar degenerative conditions, and most published studies comparing the techniques reveal similar outcomes regardless of what fusion technique is used.19–21 There are, however, certain circumstances when interbody fusion offers definite advantages. Adding an interbody fusion to a posterolateral fusion increases the rate of achieving successful arthrodesis. This is especially true when a long fusion extends to the sacrum, which is historically associated with a high risk of lumbosacral pseudarthrosis.22
Some authors have argued that interbody fusion should be combined with posterolateral fusion in other patients at high risk for failed fusion, such as smokers.23 In patients with pseudarthrosis after failed posterolateral fusion, an interbody fusion is a good salvage operation, allowing the fusion to occur in a well-vascularized bony bed rather than attempting fusion again in the scarred devascularized posterolateral space.24 Interbody fusion offers a particular advantage in the treatment of scoliosis and kyphosis or flatback deformities. The destabilizing effect of disc space preparation facilitates rotational correction, and distraction of the intervertebral space allows correction of segmental kyphosis and asymmetrical tilt of the vertebrae. In patients with isthmic spondylolisthesis who undergo deformity reduction, it has been well shown that an interbody graft offers a biomechanical advantage, which protects instrumentation by load sharing, helping to maintain the alignment achieved at surgery. Also, the L5 transverse process is often hypoplastic in these patients, so the increased bone surface area of the vertebral endplate offers a vastly larger area to which bone can fuse.
Most series evaluating the role of interbody fusion in isthmic spondylolisthesis show substantial clinical and radiographic benefit.25,26 Finally, the most common indication for interbody fusion, but probably the most controversial, is ablation of the disc space in patients with discogenic pain.27,28 Many advocates for surgical treatment of this entity argue that only interbody fusion can completely remove motion of the painful disc and that complete removal of nuclear material is necessary to eliminate the anatomic source of pain.
Biomechanics of Interbody Fusion
An appreciation of the mechanical properties of the spine after interbody fusion is essential to understanding the benefits and pitfalls of this operation. It seems intuitively obvious that placing a solid block in the disc space would prevent flexion of the vertebral motion segment. This is what occurs—whether the graft is placed from an anterior or a posterior approach. This fact has important implications for protection of posterior instrumentation. Interbody grafts significantly decrease the strain in posterior spinal implants when they are subjected to compression or flexion loads, which are the common modes of failure of these constructs in clinical practice. Various authors have shown that placing intervertebral cages decreases forces and strain in posterior implants by 56% to 80%,29,30 and the clinical benefit of establishing anterior load sharing to prevent implant and construct failure is well established (Fig. 49–2).31
Increasing stability when the spine is subject to flexion loading does not mean that the spine is more stable when subject to forces in every direction. After interbody graft placement, the motion segment is more unstable in certain directions. The pattern of instability is determined to a great extent by the direction from which the graft is placed and represents the destabilizing effect of the surgical approach32 and the preparation of the disc space. Grafts placed from an anterior approach result in increased motion when force is applied in extension; this likely represents the effect of dividing the anterior longitudinal ligament. Grafts placed from a posterior approach result in increased axial rotation of the motion segment owing to the resection of the posterior facet complexes necessary to avoid excessive dural retraction.33–37 The design of the cage—vertical, box, or threaded and whether or not the cage engages the endplates—does not seem to matter with regard to the patterns of instability created by intervertebral placement. Only the structures sacrificed during the approach to the disc are important in determining instability of the final construct (Fig. 49–3).38,39
Most surgeons make the assumption that a PLIF procedure is a stabilizing operation. The biomechanical data show that this is not entirely true. Understanding that placing an interbody graft or cage can lead to destabilization of the motion segment in certain directions is important for understanding why interbody fusion done without supplementary fixation has a high failure rate, especially when done from a posterior approach. Proponents of threaded fusion cages, which were designed to be used without supplementary fixation, argued that distraction of the disc space would stabilize the motion segment through ligamentotaxis. This argument is true. Increasing the size of the cage used to distract the disc space results in greater stabilization of the motion segment on mechanical testing.40 After cyclic loading, some subsidence is inevitable, however, and biomechanical tests done after cyclic loading of threaded cage constructs reveal decreased stability in all directions.41
Cage Characteristics
The optimal cage material is unknown. Cloward41a advocated the use of ethylene oxide sterilized allograft bone. Brantigan and colleagues16 tested 18 tricortical iliac specimens obtained from bone banks and determined that 3 of them were not strong enough to sustain anticipated loads without collapse. Current allograft products available for intervertebral use are made primarily of cortical bone and have adequate compressive strength. In designing his cage, Brantigan chose carbon fiber–reinforced polymer because of its strength and because its modulus of elasticity is similar to that of cortical bone.13 In the United States, other materials commonly used in commercially available cages are polyetheretherketone (PEEK), a nonreinforced polymer, and titanium, which has a modulus of elasticity significantly greater than that of cortical bone. The stiffness of the cage is determined not only by the mechanical properties of the material but also by the shape and design characteristics of the cage. The ideal stiffness and its role in limiting subsidence or enhancing fusion are unknown. Finite element data have suggested that stiffer cages are more likely to subside,42 but animal data have not supported this hypothesis,43 and clinical reports have not convincingly shown that metal cages are more likely to subside than cages made of polymer (Fig. 49–4).
Kanayama and colleagues44 studied the effect of cage design on stress shielding of the graft inside the cage. They determined that it was the pore size, not the stiffness of the material, that determined the load experienced by the graft. The total pore size was not important; rather, it was the size of the largest contiguous opening that determined how much force was transferred to the graft. It was implied that minimizing stress shielding is important to promote fusion, but no data were presented to support that presumption. One clinical study consistent with this hypothesis compared narrow and standard carbon fiber–reinforced polymer cages. The pore in the narrow cage was 36% smaller than the pore in the standard cage. There was a small but statistically significant reduction in fusion rate, 91.1% compared with 98.9%, but the conclusions have limitations because of methodologic flaws.45 A larger pore size seems desirable as long as the design of the cage provides adequate structural support for the endplate.
Cage design also influences the alignment achieved. An increasingly large body of data show that maintaining or restoring lumbar lordosis is an important surgical goal and that lordosis correlates with the outcome of surgery.46 Early cage designs were rectangular in shape and tended to force the vertebral endplates into parallel alignment. It is possible to achieve lordosis with rectangular cages, either by resecting bone posteriorly or by compressing the posterior disc space and causing the posterior portion of the cage to subside.47 A disadvantage of this approach is that it results in loss of posterior disc space height, which can lead to foraminal narrowing. A better solution is to use a cage that is tapered to achieve lordosis.48–50 It is commonly said that anterior distraction is necessary to achieve lordosis, but distraction of the disc space with an implant that is not tapered flattens the spine. Placing large cages to achieve lordosis is a mistake. They require more root retraction to place, and this is more likely to result in nerve injury. A better solution is to use a tapered device and not to strive for maximal anterior height restoration (Fig. 49–5).
Subsidence
In addition to achieving fusion, avoiding subsidence to maintain alignment is an important goal of interbody fusion surgery. In mechanical testing, endplate failure has a linear correlation with decreased bone density,51 and severe osteoporosis is considered to be a relative contraindication to interbody fusion because of the risk of endplate collapse. The strength of the endplate is not uniform, and the central portion, where most surgeons place their grafts, is the weakest. Mapping studies have shown that the posterolateral portion of the endplate is most resistant to compression.52,53 Placing the cage or graft in the area where the bone is strongest makes biomechanical sense54 but may not be consistent with the goals of an individual surgical case. If it is necessary to increase lordosis, better results are achieved by anterior placement. Putting the graft in the posterolateral position might be stronger but would block compression of the posterior disc space and limit lordosis. This is especially true with rectangular cage designs or vertical titanium mesh cages.
Cage designs that optimize contact with the strongest portions of the endplate are desirable and include cloverleaf designs and large round cages, both of which have peripheral endplate contact.55 Practical use of large round cages is limited to anterior surgery because of the excessive dural retraction that would be necessary to place such an implant. Lordotic cages placed laterally also achieve near-optimal endplate contact. The surface area of the endplate that needs to be in contact with the graft is unknown but is commonly stated to be 30%. This percentage is based on a study of thoracic vertebrae subjected to a physiologic load of 600 N. Failure of the endplate occurred in 80% of specimens subjected to that load when the grafts were 25% or less of the endplate surface area. When the grafts exceeded 30% of the endplate surface, 88% of the specimens remained intact (Fig. 49–6).56
< div class='tao-gold-member'>
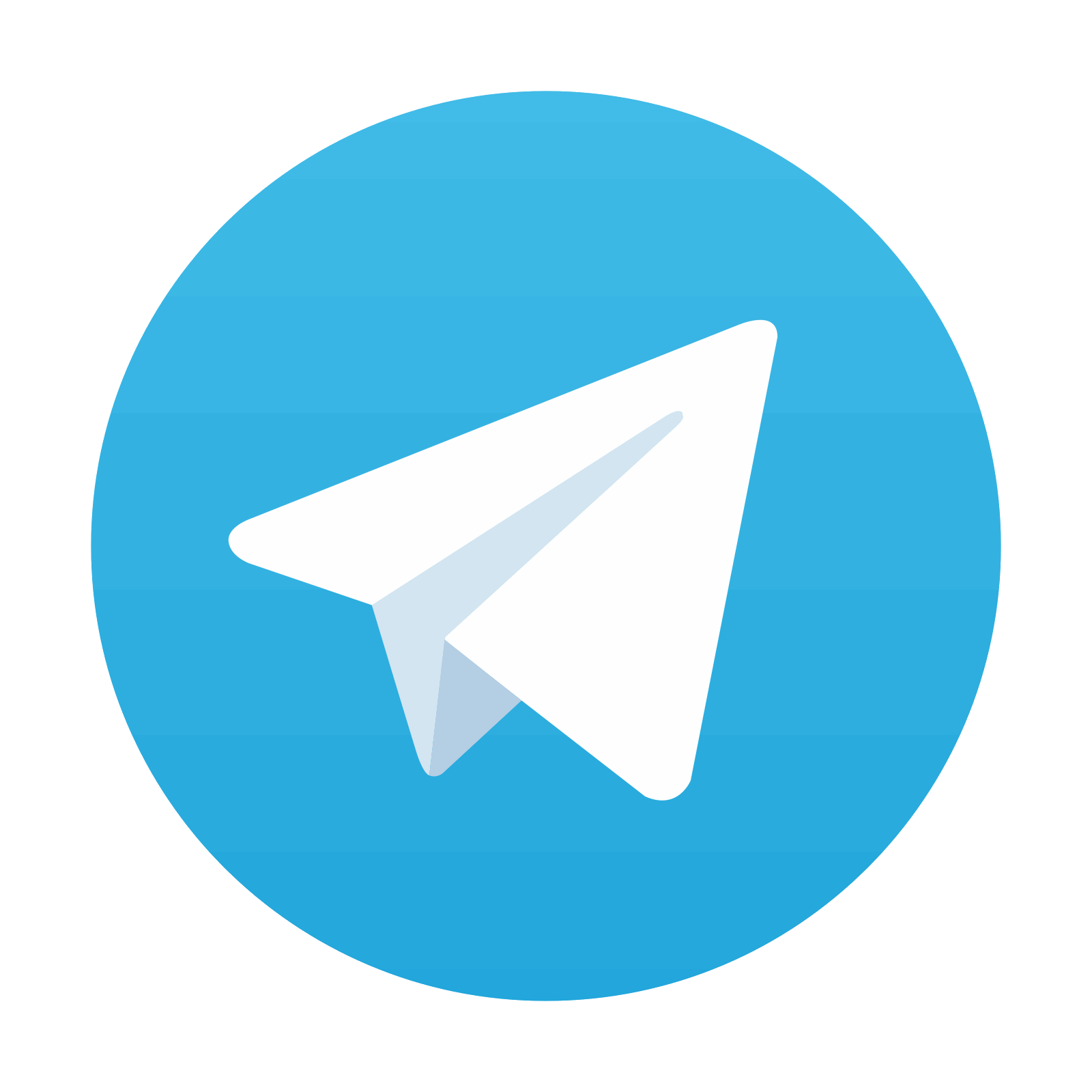
Stay updated, free articles. Join our Telegram channel

Full access? Get Clinical Tree
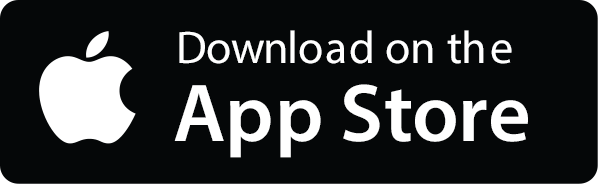
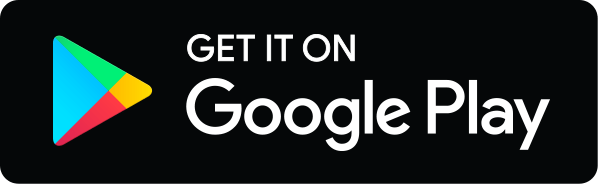