2
Shoulder Range of Motion
Measuring the range of motion of any joint is a basic part of any musculoskeletal evaluation. Although measuring motion is relatively easy for a hinge joint, which has only 1 or 2 degrees of freedom, measuring motion of the shoulder complex presents some unique challenges. These challenges include making a determination of exactly which motions are most clinically important, which components of the shoulder complex are contributing to those motions, and how to measure them. There is increasing appreciation that there is a wide variety of factors that affect shoulder range of motion.
This chapter will discuss traditional methods of measuring shoulder range of motion and critically examine them in light of new biomechanical and clinical knowledge. Advances in the clinical techniques for measuring motion of the shoulder complex will be discussed. In this chapter, new recommendations for measuring shoulder rotations and distinguishing glenohumeral from scapulothoracic motions will be introduced.
Nomenclature
A common language regarding shoulder range of motion is important for several reasons. First, accurate measurements of motion is important for making an accurate diagnosis. A stiff shoulder can often simulate rotator cuff tendinitis, or it may aggravate a preexisting rotator cuff condition, and treating the tendinitis alone may not resolve the loss of motion. Second, health care providers need to be able to communicate patient motions to each other reliably and reproducibly. This is important whenever treatment is initiated and specific motion goals are set by the provider. For example, if the surgeon sets a limit of 30 degrees of external rotation as the limit for 4 weeks after a shoulder stabilization, the physical therapist needs to know if that is glenohumeral motion or combined glenohumeral and scapulothoracic motion. Likewise, if a physician thinks that an athlete has lost internal rotation of the shoulder, the trainer, therapist, and physician should be measuring the same motion.
This system of measurement should allow international communication about motions and comparison of patients. Reproducible measurement of motion is necessary for making the diagnosis and to monitor treatment. Motion of the shoulder complex is an important component of outcome measures, including shoulder scores. Shoulder motion is currently an important part of calculating impairment ratings.1
The function of the upper extremity is to position the hand in space, but any measure of hand position will necessarily involve a contribution by the other joints in the upper extremity, including the elbow and wrist. As a result, a standard was needed that all practitioners could use that would have the same reference points and be reproducible. The evolution of the measurement system of the upper extremity motions had its origins in the early 20th century.
The first attempt to provide order to measuring shoulder motion was by Silver in 1923.2 He proposed a system of zero positions for every joint from which a particular motion would be measured. This was modified by Cave and Roberts in 19363 (Fig. 2-1), who felt that the best starting position was with the arm at the side with the elbow flexed at 90 degrees. Although they provided a system for measurement of range of motion of the shoulder, they did not provide normative values. The system recommended by Cave and Roberts included exhortations to measure the other extremity for comparison. They also recommended the use of a goniometer and noted that motions of joints above and below the affected joint should be recorded.3
In 1958, the American Medical Association (AMA) published “A Guide to the Evaluation of Permanent Impairment of the Extremities and Back.” This guide inferred that disability and impairment could be determined by measuring joint motion alone. It provided measures for shoulder range of motion and the disability rating for lost motion as a percentage of the extremity (Table 2-1).
FIGURE 2-1 The zero positions of Cave set the standards still used today. (Adapted with permission from Cave ER, Robert S. A method for measuring and recording joint function. J Bone Joint Surg Am 1936. 18(2):455–465.
In 1959, Carter Rowe was appointed by the American Academy of Orthopaedic Surgeons to be the chairman of a committee to study the best way to measure joint motion. The Committee on the Study of Joint Motion published its findings in 1963.4 The American Academy of Orthopaedic Surgeons (AAOS) adopted the concept of Cave and Roberts3 for defining a zero position of the joint and published it in pamphlet form in 1965.5 The recommended system was modified by the AAOS to include internal rotation up the back (Fig. 2-2). The AAOS averaged the measures of shoulder motion from four sources (Table 2-1), and it should be noted that these values were different from those published by the AMA.
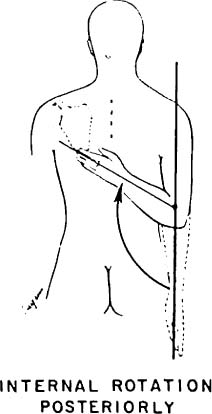
FIGURE 2-2 Internal rotation up the back was added by the American Academy of Orthopaedic Surgery as a helpful measurement. (Adapted with permission from the American Academy of Orthopaedic Surgeons. Joint Motion: Method of Measuring and Recording. Chicago: Author; 1965.)
These publications from the AMA1 and AAOS5 established the standards for measuring shoulder range of motion utilized for many subsequent generations of clinicians and providers. The important clinical measures were elevation in abduction, elevation in flexion, extension of the arm at the side behind the body, internal and external rotation with the arm at the side, external and internal rotation with the arm at 90 degrees of abduction, and adduction of the arm across the body.6 The normative values in Table 2-1 have been the standard since their publication in 1958 and since being accepted by the AAOS in 1964.
It has become increasingly appreciated over time that motion of the upper extremity is a complicated set of movements by multiple joints, and new knowledge has changed how we think about shoulder motion. As a result, it has become appreciated that there were several problems with the recommended standards promulgated by the AAOS. It should be noted that this system provides a measurement that is the summation of motion of the glenohumeral joint, scapulothoracic joint, and the other joints of the upper extremity. It was not specified whether the patient was to be sitting or supine, nor whether active or passive range of motion was measured. The body references for making the measures were implied by the diagrams, but never really specified. Values for internal and external rotation of the shoulder did not specify if the measures were to be made with the arm in the plane of the body or in the scapular plane.
Variables Affecting Range of Motion Measurements
Since the original descriptions, numerous studies have addressed the subject of measuring shoulder range of motion, and there are textbooks devoted to these measurements.7,8 There are many factors that influence the range of motion measured by health care practitioners. These include the type of measuring device, the complexity of the joint motion being measured, whether the motion is measured actively or passively, the sex of the subject, the age of the subject, the experience of the examiner, the dominance of the extremity, whether the subject has exercised, and what time of the day the measurement is performed.9,10
The first dilemma in reporting shoulder motion is what to call motions in certain directions. The nomenclature for describing shoulder motion has a long history, but most sources make a distinction between forward flexion, where the arm is raised in the sagittal plane, and abduction, where the arm is elevated in the coronal plane (Fig. 2-3). The scapula sits on the convex thorax and results in an orientation that is ~30 degrees anterior to the coronal plane. This position has been referred to as the plane of the scapula, and elevation occurring in this plane is termed scaption or elevation in the scapular plane (Fig. 2-4).
Extension of the arm is typically reported as being performed with the arm in an adducted position; however, adduction of the arm can be reported with the arm at the side or with the arm elevated at 90 degrees. When adduction is performed with the arm in elevation, it is typically called horizontal adduction, and it is sometimes referred to as “cross-body adduction” (Fig. 2-5). If the arm is elevated 90 degrees and the arm is extended, it is called “horizontal extension.”
Rotations of the arm also were sometimes initially described as medial (toward the body) or lateral (away from the body). This nomenclature makes sense with the arm in an adducted position at the side of the body but not with the arm elevated. As a result, these arm rotations are typically described now as internal or external rotation. Thus, with the arm adducted at the side, external rotation with the arm at the side is typically measured. Internal rotation up the back has become the standard measure of internal rotation in the adducted position. Likewise, rotations with the arm abducted 90 degrees are also described as internal or external rotation.
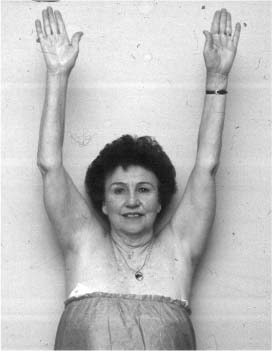
FIGURE 2-3 When the arm is elevated in abduction or in flexion, the end point is the same position where the arm is fully elevated.
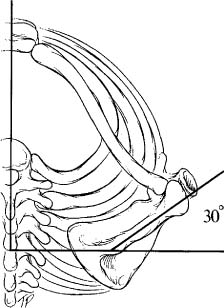
FIGURE 2-4 The plane of the scapula is due to the angled position of the scapula on the thorax and is typically 30 degrees to the plane of the body.
FIGURE 2-5 Horizontal adduction is when the arm is brought across the body in a specified degree of elevation of the arm.
The complexity and ambiguities of this system prompted some to suggest a global coordinate system to describe shoulder motion11 (Fig. 2-6). This would allow the examiner to describe exactly where the arm could be positioned in space. This system did not gain widespread acceptance, however, because of its complexity and lack of practicality.
Validity of Measurements
An important issue in any measurement system is the validity of the measurements.9 In other words, does a particular system really measure what it says it does? For most joints, the motion measures are supposed to be a representation of the central axis of the limb, which is usually assumed to be roughly the center of the long bones making up that segment. When measuring knee motion, it is assumed that the measure is actually of the femur where it articulates with the tibia, but overlying soft tissue, swelling, or variations in the bony anatomy can significantly affect the measurement.
In the shoulder, the validity of the measure is hampered due to the variability of the bony anatomy and because there are several moving parts that contribute to the motion. Additionally, the motions are often measured in relationship to the thorax, which can have variable size and shape. As will be discussed later, it has always been assumed that the shoulder elevates to 180 degrees in most people, but very accurate studies using sophisticated three-dimensional electronic tracking devices and computed tomography (CT) scans have demonstrated that true elevation of the humeral shaft relative to a vertical line in the thorax is around 150 to 170 degrees.12–14
The validity of the traditional measurements of shoulder rotations also has been questioned by these studies: what exactly are we measuring when we measure shoulder internal and external rotation? Are the measures actually measuring the bone movements or the bone and soft tissue movements? Another important issue when measuring shoulder motion is the reliability of the measurements. Reproducibility or intratester reliability refers to the ability of a single examiner to have a repeat measurement that is the same as the first measurement, and it is called test-retest reliability. A second type of reliability is the ability for two examiners to measure the same thing and is known as intertester reliability (see Appendix).
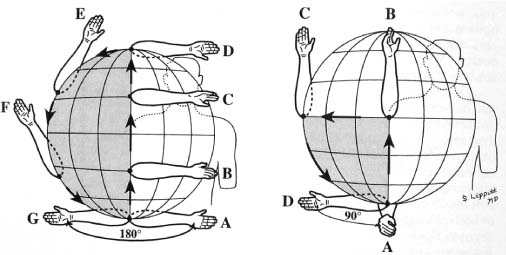
FIGURE 2-6 A global coordinate system was recommended by Matsen et al to define arm position specifically in space. (Adapted with permission from Pearl ML, Jackins S, Lippitt S, Sidles JA, Matsen F. Humeroscapular positions in a shoulder range-of-motion-examination. J Shoulder Elbow Surg 1992;1:296–305.)
In other words, are the measurements going to be the same if repeated by the same examiner or another examiner? The validity, reproducibility, and reliability of the measurements are critical issues when discussing range of motion of the shoulder complex.
Techniques of Measurement
An important element of measuring shoulder motion is the technique used for measuring the motion. Upon clinical examination, there are basically three methods that can be used to measure shoulder motion: visual estimation of the motion, goniometric measurement, and measurements obtained using inclinometers.
Visual Estimation
Visual estimation of motion is probably the most commonly used method in the office setting because it is cheap, easy, and quick. Several authors have suggested that visual estimations of range of motion of joints are not as accurate as using instrumented measuring devices, but there are a few studies that actually study this issue. In the lower extremity Watkins et al15 found that goniometers were better than visual estimates for measuring passive motion of the knee. Williams and Callahan10 studied the results of measuring a fixed angle of shoulder flexion by 22 physical therapists of a single male model. They found that there was no difference between the visual estimate and the goniometric measure by the therapists and concluded that experienced examiners can reliably estimate some shoulder motions without a goniometer. Most researchers would agree that visual measurement is acceptable in the office evaluation of the shoulder range of motion of patients, but goniometric measurements are necessary for studies or other special applications.
Use of Handheld Goniometers
The standard handheld goniometer with a stationary and a movable arm remains the most frequently used device for measuring shoulder motion because it is inexpensive, portable, and reliable and has been extensively studied.16,17 The handheld goniometer comes in many models and shapes, with long arms, short arms, telescoping arms, and metal or plastic arms, and may measure in 1- or 5-degree increments. There are many variables that affect the use of the hand-held goniometer, but probably the most important is the experience of the examiner. Identification of the “zero position,” as advocated by Clark et al18 and subsequently the AAOS, is important. The ability to identify a reliable bony landmark is another important variable.
Multiple studies have demonstrated that the reproducibility of handheld goniometers for repeat measurement of joint motions is high for a single examiner. Studies have demonstrated that the intertester reliability using handheld goniometers is only fair to moderate (Table 2-2). High intratester reliability and low intertester reliability when using handheld goniometers to measure joint motion are common findings of studies of other joints.19,20 As a result, when reading the literature regarding shoulder range of motion measurements, it is important to note both “how” the motions are measured as well as “who” performed the measurements. Studies that employ multiple examiners are likely to have some error due to this variable, which should be taken into account when assessing their results.
FIGURE 2-7 An example of a mechanical inclinometer available on the market. (Courtesy of Sammons Preston Rolyan.)
Use of Inclinometers
Another technique for measuring shoulder range of motion involves the use of inclinometers. These are basically leveling devices that are commonly used by engineers to determine the relationship of a structure to the horizontal plane. These devices were initially designed for use in the spine to determine the amount of deformity present in the coronal and sagittal planes.21–23
Two types of inclinometers have been described: mechanical and digital (Figs. 2-7, 2-8). Mechanical arthrometers use a weighted pendulum or a fluid-level indicator (hydrogoniometers) to measure the range of motion. These inclinometers work like a plumb line, using gravity as the mechanism for determining the relationship to a horizontal position. Technically, inclinometers must be firmly applied to the skin so that there is no motion or tilting of the device while the measurement is taken.
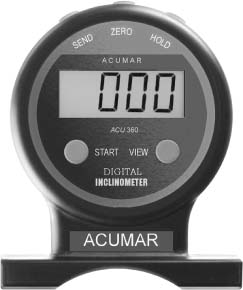
FIGURE 2-8 An example of a digital inclinometer available on the market. (Courtesy of Acumar Technology.)
Clarke et al18 in 1975 were the first to report the use of a based inclinometer to measure glenohumeral and scapular range of motion. They reported satisfactory results by using this method, with less than 7% interobserver error. Dover et al24 used a mechanical inclinometer with a needle to measure the range of motion in 100 female athletes to measure the external and internal rotation and the flexion extension of the shoulder. They calculated the reliability of this device for measuring range of motion and obtained an Intra Class Correlation Coefficients (ICC) value of a mean of 0.99. These devices generally have relatively good reliability for both intraobserver and interobserver reliability (Table 2-2).
Digital inclinometers have been used more recently by many authors for measuring shoulder range of motion.25–29 These inclinometers have internal gravity sensors that indicate the range of motion by calculating the angle from the vertical. Johnson et al28 studied the reliability and validity of a digital inclinometer to assess scapular motion. They compared the data taken by a two-dimensional digital inclinometer to those taken by a three-dimensional magnetic tracking device. The digital inclinometer demonstrated good to excellent reliability and validity for the measurement of scapular motion with ICC, which varied from 0.89 to 9.96.
Factors Affecting Measurements
Age and Range of Motion
Another factor influencing the results of the measurements of shoulder motion is the age of the patients. Several studies have addressed this issue and reported that there is decreasing shoulder range of motion with age.18,30 Murray et al31 measured shoulder range of motion in younger men and women (range 25–36 years old) and older men and women (range 55–66 years old) using handheld goniometers. They did not find differences between the two age groups, but they did find differences between men and women for several measures.
Boone and Azen30 divided their cohort of 109 healthy males into two groups: 53 subjects under age 19 and 56 subjects over age 19. They found the biggest changes between the two groups were with horizontal extension, forward flexion, backward extension, and both internal and external rotation of the shoulder with the arm elevated 90 degrees.
Walker et al32 evaluated 60 subjects between the ages of 60 and 84. They found that measurements were significantly different from those reported by the AAOS, and suggested that older individuals required revised standards for range of motion. Boone and Azen30 examined 50 high school athletes and found that that age was a factor in external rotation only, with the younger age group (age 12–15) having more external rotation than the older group (age 16–18).
The most comprehensive study of the relationship of age to shoulder motion was reported by Barnes et al,33 who measured shoulder motion in 280 subjects, with 40 subjects in each decade from 0 to 70 years of age. They found a nonlinear decline in motion with age for both passive and active motions measured except for internal rotation, which seemed to increase with age. The amount of change per year was the greatest for abduction and external rotation with the arm adducted at the side. Loss of flexion over time was not linear; the researchers thus concluded that a loss of forward elevation in subjects under age 40 should not be attributed to the aging process alone.
Gender and Range of Motion
Another factor influencing measurement of shoulder motion is the gender of the subjects. Allander et al34 examined a general population and found that women had significantly increased shoulder motion compared with males for most age groups. Clarke et al18 reported that, in general, males had 92% less motion than females, and that this difference was most marked in abduction (females 85.6 degree vs 77.4 degree for males) as compared with internal and external rotation. Walker et al32 found significant differences between men and women for all measures except internal rotation (Table 2-3). Murray et al31 found that women had a greater total arc of internal and external rotation of the shoulder compared with men, but their study examined only two age groups, with a large discrepancy in ages between the two groups. Barnes et al33 found significant differences between all motions measured between men and women for both passive and active range of motion.
Dominance of the Extremity and Range of Motion
Another factor influencing the shoulder range of motion is the dominance of the extremity. Clarke et al18 found no difference based on arm dominance between males and females for any age group or any measure of shoulder motion. Boone and Azen30 found variable differences between right and left extremities depending on age, but they did not make note of the dominance of the extremity. They concluded that overall, both extremities were similar and that, in general, the opposite side could be used for comparison for most joints.
Kronberg et al35,36 found no difference in range of motion between the dominant and nondominant shoulders for both males and females. Barnes et al33 found that the nondominant shoulder had statistically significantly greater active internal rotation, passive internal rotation, active extension, and passive extension compared with the dominant side; however, the dominant arm had significantly more external rotation both passively and actively with the arm abducted and at the side compared with the nondominant shoulder. The researchers found no relationship between shoulder range of motion and sports participation, and they concluded that using the opposite shoulder for comparison of rotations was questionable.
Patient Positioning and Range of Motion
There is increasing evidence that body position can affect range of motion measurements, particularly in the shoulder. The main practical concern is whether the examination may be different if the patient is in a sitting or standing position. Another issue is whether the scapular motion is influenced by contact to a rigid surface, such as when lying on a flat surface or when sitting with the thorax against the back of a chair. It is possible that patients who have pain will obtain better range of motion supine if the effects of gravity are eliminated. Some patients in wheelchairs may find examination in the supine position difficult.
Studies support the idea that measures of shoulder range of motion are influenced by whether the patient is supine or sitting. Sabari et al37 studied flexion and abduction of the shoulder in 30 normal volunteers using handheld goniometers by one examiner with the subjects supine and then sitting. They found that the intrarater reliability between trials was high (0.94–0.99) for the measures, so there was good reproducibility of the measures whether they were done with the patient sitting or supine when done by one person.
There were significant differences in the measures obtained supine versus standing, however, even when measurements were done by just one examiner. Sabari et al found that with patients sitting, the examiner had to be diligent to watch that the patients did not use compensatory motions of the thorax or pelvis to influence motion. They concluded that either position was useful for measuring motion, but that the exact position of the subject should be noted when reporting or studying shoulder motion.
There have been several studies that have evaluated the effect of positioning on the measurement of shoulder rotational movements.38,39 These studies will be discussed in detail later in this chapter because the measurement of shoulder rotations is a controversial and important subject; however, there are indications that shoulder rotational movements can be significantly influenced by whether the patient is supine or standing. We believe that this is due in part to the difficulty in stabilizing the scapula with the patient supine versus using visual and tactile cues with the patient standing.
Active or Passive Motion
Another important variable to consider when measuring shoulder range of motion is whether active or passive motion or both should be measured. In the clinical setting, it is important to measure if the patient has deficits in motion in any one direction. Whether the motion is active or passive, pain can influence the ability of the patient to move the shoulder. The variables that affect passive motion are discussed later in this chapter.
The influence of active versus passive motion of the shoulder was studied by Sabari et al37 using the same cohort as reviewed earlier. The researchers found that there were no significant differences in shoulder flexion or abduction with active or passive motion. They examined only normal volunteers, however, so this finding may not be capable of extrapolation to abnormal subjects. The experience of most clinicians is that active and passive motions can be significantly different depending on the pathology of the patient.
Clinically Relevant Biomechanics of Shoulder Motion
New knowledge of shoulder biomechanics and shoulder motion challenge the previously held misconceptions about how the shoulder moves and functions. Biomechanical studies have shown that shoulder motion is infinitely more complicated than previously appreciated. This is especially true for evaluating glenohumeral and scapulothoracic motions where there are movements in three dimensions that may have clinical importance.
Shoulder motion is a complex interaction of the bony anatomy, the ligamentous laxity, and the activity of the muscles. This interaction of static and dynamic components makes the shoulder motion highly variable and difficult to study. Each shoulder motion and translation places a different portion of the ligaments under stress, and pathological conditions may affect some parts of the ligaments but not others. It is our position that glenohumeral motions can be distinquished from scapulothoracic motions in rotation but not in elevation. The next section will present the biomechanical rationale for these conclusions and clinical support for a new method of measuring and reporting shoulder motions.
Measurement of shoulder range of motion in rotation can distinguish between glenohumeral and scapulothoracic measurements.
When the arm is moved in space, it is a combination of motion at the glenohumeral joint and the scapulothoracic joint. Although the resulting motion of the humerus is relatively easy to determine, the contribution of the individual components to the motion is more difficult. Early observational studies of shoulder motion estimated that glenohumeral motion occurred in the first 90 degrees of shoulder elevation and that scapular motion occurred after that.40,41 The 1964 AAOS handbook for range of motion suggested that by stabilizing the scapula, isolated glenohumeral elevation would be only 90 degrees.40 Subsequent authors have suggested that, with elevation, glenohumeral motion can be distinquished from scapulothoracic motion. We believe that this distinction is very difficult to make, however, and that its clinical utility is yet to be determined.
Radiographic Studies of Shoulder Elevation
Subsequent studies using radiological methods established the relationship of scapulothoracic motion to glenohumeral motion.14,42 These studies were performed by having the subject elevate the arm from the side to full elevation. These were semi-static studies, where the arm was held actively by the subject in one position, and radiographs were then taken. The contributions of glenohumeral motion and scapulothoracic motion to each level of elevation were measured from the radiographs (Table 2-4).
Inman and Abbott14 were the first to use this technique. They noted that over the entire arc of motion, the ratio of glenohumeral motion to scapulothoracic motion was 2:1; however, the ratio of glenohumeral to scapulothoracic motion was variable at lower levels of elevation. Inman and Abbott noted that “in the first 30 to 60 degrees of elevation, the scapula seeks, in relationship to the humerus, a precise position of stability which it may obtain in one of several ways. Either the scapula remains fixed, motion occurring at the glenohumeral joint until the stable position is reached, or the scapula moves lateral or medially on the chest wall, or in rare instances oscillates until stabilization is reached.”14 Their observation that the motion of the humerus and the scapula in the first 30 to 60 degrees of elevation is highly variable has been substantiated by several subsequent authors.12 Once this “setting stage” was reached, the ratio of glenohumeral motion to scapulothoracic motion was fairly constant at 2:1. Inman and Abbott also noted that external rotation of the humerus is necessary for full elevation of the humerus.14
Studies utilizing radiographs have inherent difficulties related to the technology. First, the radiographs can study motion only in one plane; although an anteroposterior radiograph can accurately measure the relationship of the humerus to the scapula, it cannot accurately evaluate the rotation of the scapula as it elevates. The scapula actually moves in all three planes with elevation of the arm, and more sophisticated methods are needed to accurately measure these movements.
The second deficiency of these studies is that if the subjects are standing, they have to move the arm to a specific position and then hold it there. This means that muscle activity is influencing the arm position, and it may not represent what occurs when the arm moves in a dynamic fashion. If the patient is supine, then the scapular position may be affected by the table; in this instance, radiographs taken of the static position may be influenced by the lack of muscle activity. These factors may influence both the position of the scapula on the thorax as well as the relationship of the humerus to the scapula.
New technology has allowed the more accurate study of the complex relationships of all of the component parts of the shoulder complex. Three-dimensional electromagnetic devices work by allowing the definition of the relationship of the bones to the thorax and to each other. Basically, the technology works by creating a reference source, and motion of the electromagnetic sensors around that reference are then measured. These devices are very precise, with accuracy of 0.15 degree for rotations and 0.8 mm for translations.
These three-dimensional electromagnetic devices can be attached to the skin with tape, but there can still be inaccuracies due to skin movement independent of the bony structure. To increase the accuracy of the measure movements of the bones, pins can be inserted into the bones and the devices attached to the pins (Fig. 2-9). The advantage of these devices is that the sampling rate is from 10 to 21 Hz, which makes them capable of studying dynamic motions.42 Although these devices can sample fast enough to track an extremity as it moves through space (e.g., performing range of motion), they do not sample fast enough to study a throwing motion dynamically or rapid motions seen in athletic activity.
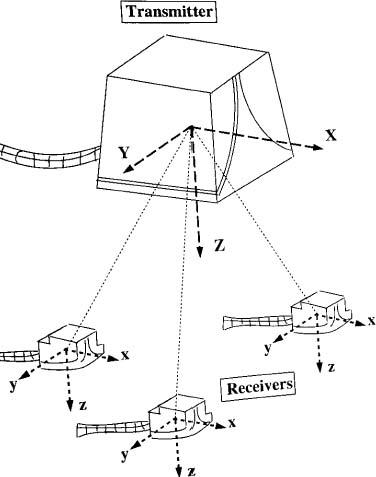
FIGURE 2-9 Three-dimensional electrogoniometers have electrodes that can be attached by pins in the bones or directly to the skin. (Adapted with permission from Fung M. Visualization and quantification of the coupled passive shoulder motion in vitro. In: Biomedical Engineering. Baltimore: Johns Hopkins University; 1997.)
The first studies using this technology were performed by the shoulder group at the University of Washington and comprised eight human volunteers who had pins inserted into their humeri, scapulae, and clavicles.11 Subjects then moved their arms to full elevation, and motions of all of the bones in relationship to the thorax could be described in detail. The researchers found that true elevation was not 180 degrees but 140 degrees plus or minus 7 degrees.11 Because they did not measure shoulder motion with handheld goniometers, it is unclear what is the exact relationship of true shoulder motion to goniometric measures of shoulder motion.
Another study using three-dimensional electromagnetic sensing devices in a cadaver model found a maximum elevation of the humerus to the thorax of 160 degrees in the sagittal plane, 158 degrees in the coronal plane, and 162 degrees in the scapular plane43 (Fig. 2-10). In that study, the average age of the cadaver specimens was 76, and the motion was performed passively by the examiner.12 Using digital fluoroscopy, Mandalidis et al44 found that maximum arm elevation was 162.4 plus or minus 6.6 degrees. A review of the literature reveals that most studies demonstrate that total elevation of the arm is less than 170 degrees.
Although shoulder abduction is reported to be 180 degrees, biomechanical studies show that it is rarely greater than 170 degrees.
In our laboratory we performed similar experiments by placing pins in the bones of cadaveric specimens. Once the sensors were in place, we passively moved the cadaver extremity in space in different directions. After the experiments were completed, the specimens underwent CT scans. This allowed exact definition of the bony anatomy of the cadavers, so that the motions and bony anatomy recorded were specific for that specimen. A three-dimensional coordinate system was designed for each bone (Fig. 2-11). The exact relationships of the bones to each other and to the thorax were now available in a dynamic, three-dimensional model.
We found the relationship of glenohumeral motion to scapulothoracic motion over the entire arc of motion to be 2:1; however, the ratio differed depending on the level of elevation of the extremity and on whether the arm was elevated in the plane of the body (coronal) or the scapular plane (horizontal flexion of 30 degrees) or in forward flexion (Table 2-5). In this model, the scapular motion was found to be largely dependent upon humeral motion, but because this was a purely passive model, there was no influence of muscle action on the motions.
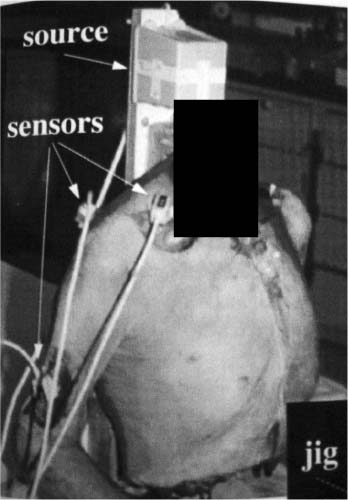
FIGURE 2-10 Three-dimensional electrogoniometers can be attached to the bones with pins to follow motion exactly. (Adapted with permission from Fung M, et al. Scapular and clavicular kinematics during humeral elevation: a study with cadavers. J Shoulder Elbow Surg 2001;10(3):278–285.)
Lease et al45 suggested that, in a passive model, the bones of the shoulder acted as a “three-link spatial kinematic chain,” such that above the “coupling point” described by Inman and Abbott,14 the scapula position and the clavicular position could be roughly predicted from the humeral position. The impact of muscle activity and other factors, such as fatigue, velocity, and resistance, however, was not considered. As a result, the differences between active and passive motion were not delineated in our study of shoulder motion.
These studies contribute to the controversy over what exactly controls motion of the bones as the arm is positioned in space. Does the position of the humerus influence the scapular motion, or does the scapula position determine the humeral position? Some clinicians suggest that scapular control is necessary before the athlete can control the humerus. Because the position of the humerus largely determines where the arm is in space, some argue that the scapula functions merely to provide a stable base for the humerus.
FIGURE 2-11 A three-dimensional coordinate system is set up for the individual bones of the shoulder and the spine to generate motion data. (Adapted with permission from Fung M, et al. Scapular and clavicular kinematics during humeral elevation: a study with cadavers. J Shoulder Elbow Surg 2001;10(3):278–285.)
These ideas are not entirely supported by the results in a passive model where the muscles are not firing (e.g., when an examiner passively elevates the arm or when the subject is under anesthesia). In these models the relative motions of the humerus and scapula are determined in part by the relationship of the bones in that particular subject (i.e., the version of the humeral head and of the glenoid) and in part by how the ligaments influence the bone movement. In a passive model, the humerus can be moved freely until the maximum length of the ligaments is reached. At that point the ligaments will become tight and pull on the bones to create movement. It is also possible that in a passive model the humeral head may push the glenoid and the scapula in one direction or another. In a passive model with no muscle activity, the scapula theoretically does not move until it is pushed or pulled.
Effects of Muscle Activity on Shoulder Motion
It can be argued that motion of the extremity is different when there are muscles actively influencing the relationship of the shoulder complex and the bones to each other; however, the impact of muscle activity on the relationship of scapular to humeral motion is less easily measured. This influence of muscles on shoulder motion can only be studied in dynamic experimental models where motion of the scapula and humerus is allowed without restraint. The effect of muscle activity on the shoulder complex movements has been studied by several researchers.
McClure et al13 studied active shoulder motion three dimensionally in living subjects. They used the same technology with three-dimensional electromagnetic sensors to study scapular and humeral motion by securing the sensors to pins placed into the scapulae of eight healthy volunteers. The researchers found that the ratio of glenohumeral to scapulothoracic motion was 1.7:1 over the entire range of motion in abduction and 2.0:1 for flexion. They also found that the scapular motion was minimal before 30 degrees of elevation, and there was almost a linear relationship between humeral and scapular motion for elevation overall; however, at the extremes of motion (low degrees of elevation and high degrees of elevation), the scapular motion was variable, and there was a nonlinear relationship between the two bones.
McClure and colleagues also found that maximum scapular rotation occurred at the extremes of elevation, suggesting that even in subjects actively elevating their arm, at some point the scapular position will be determined by the humeral position and tension on the soft tissues.13 They postulated that above 90 degrees the shoulder ligament tension may influence scapular motion, which is consistent with our findings in a passive cadaveric model.12,44 These studies support one school of thought, which postulates that the scapular and humeral motions are linked primarily by the ligament constraints and not the muscular components, particularly at low load.
Other studies would suggest that muscle activity does significantly affect the relationship of the humerus to the scapula and of the scapula to the thorax.46–49 Sugamoto et al47 studied these relationships using radiographic fluoroscopy performed at 7.5 frames per second. The researchers asked subjects to abduct their arms in the scapular plane and then adduct them back, completing the cycle in either 2 or 4 seconds. They found that the ratio of glenohumeral to scapulothoracic motion was fixed at 2.3:1 at the slow speed when the arm was abducted over 40 degrees. At high speeds the ratio was different due to a higher contribution of glenohumeral motion at the lower elevations. Sugamoto et al concluded that scapulohumeral rhythm was influenced by the velocity of arm elevation.
Others have demonstrated that the ratio of scapulothoracic to glenohumeral motion changes when the arm is subjected to a load as it goes through a range of motion. McQuade et al50 studied the ratio of glenohumeral to scapulothoracic motion when the subject was asked to abduct the arm to fatigue when lifting an 8-pound hand weight. They found that with muscle fatigue the ratio changed minimally in the frontal plane (from 2.1:1 to 2.8:1), but in other planes the ratio changed significantly, from 4.8:1 to 7.1:1 in the lateral plane and from 5.3:1 to 8.1:1 in the plane perpendicular to the scapular body. The researchers concluded that changes in the bony relationships could be a factor in the etiology of shoulder pathologies such as rotator cuff impingement (see Chapter 4). In a second study in which the arms of normal volunteers were stressed to the point of fatigue, McQuade et al51 found that there were significant changes in scapulohumeral ratios, particularly in midrange to full elevation (60 to 150 degrees).
Fayard et al52 used a fluoroscopic technique to evaluate shoulder range of motion with and without a 1.5-kg (5-pound) weight attached to the arm. They found that the glenohumeral joint contributed to elevation at lower degrees when the weight was applied; as a result, the ratio of glenohumeral to scapulothoracic motion changed, particularly at the lower elevations. These studies would seem to support the contention that the relationship of the humerus, scapula, and thorax is influenced by muscle activity. These observations also have relevance for the theory that scapular positioning may be part of the pathological processes of pain and dysfunction in the overhead athlete (see following).
Elevation: Flexion versus Abduction
When elevating the arm, there are important differences in the relationship of the humerus to the scapula. An et al42 studied elevation of the arm in a cadaver model and noted that maximum elevation occurred in the plane of the scapula with the humerus externally rotated. If one tries to elevate the arm in abduction with the arm internally rotated, the greater tuberosity cannot clear the acromion and superior glenoid, and there is bony contact between these surfaces; however, if the humerus is rotated externally, the arm can be elevated to full elevation when the plane of elevation is in abduction. An et al found that maximum elevation in an area 26 to 30 degrees anterior to the plane of the scapula was not affected by internal or external rotation.
Subsequent authors misinterpreted this to mean that the arm can be elevated only in maximum external rotation. Further study has demonstrated that for maximum elevation of the arm in flexion, the humerus is in an internally rotated position. Fung et al12 also found that with elevation in the scapular plane, the humerus tends to rotate externally, but with elevation in flexion (i.e., the sagittal plane), the humerus tends to rotate internally. These studies indicate the complexity of the relationship of the humerus and arm position to the scapula and thorax for elevation of the arm.
Measuring Shoulder Elevation
One of the most important functions of the shoulder is to elevate the arm overhead. This motion is important for many activities of daily living, occupations, and sports activities. The ability to reach overhead is an important part of rehabilitation after surgery and for satisfaction after surgical procedures. Although motion alone is important, alleviation of pain is a major concern of most patients. As a result, when examining elevation in a subject, the presence or absence of pain can significantly influence the results. Elevation of the arm can be done in the plane of the body (coronal plane), or the scapula (forward flexed 30–40 degrees) or in flexion (in front of the body).
Measuring Shoulder Abduction
Abduction of the shoulder is most commonly measured with the patient standing, although it can be done with the patient supine. It is best to have the patient undressed or in a gown that allows visualization of the shoulder complex as the patient lifts his or her arms. Although the patient may be able to lift his or her arms, he or she may use an altered scapulothoracic and glenohumeral rhythm to reach full elevation. Some have called this movement altered scapulothoracic rhythm, or “scapular dyskinesis.” The manner in which a patient elevates his or her arm is important and should be noted, along with the degree of elevation.
When patients abduct their arms, it is best to have them forward flex the arm ~30 degrees so that the arm is in the plane of the scapula. This position allows the greater tuberosity more room to clear the acromion process. It is also best if patients elevate the arm with the thumb up, which places the arm in external rotation (Fig. 2-12). This external rotation is necessary to reach full elevation with the arm in the plane of the scapula.
The goniometric measurement is performed by placing one arm of the goniometer vertically so that it is 90 degrees from the horizontal (90 degrees to the floor) (Fig. 2-13). It should be aligned over the center of the shoulder joint if possible; once the patient has reached full elevation, the other arm of the goniometer should be centered on the arm segment. If the patient experiences pain, an assistant may need to hold the arm in the position while the measurement is made.
FIGURE 2-12 Full elevation in the scapular plane is best accomplished with the thumb in an up so that the humerus is externally rotated as the arm elevates.
Once the patient has reached the highest level that he or she can reach, the examiner can passively assist the arm to see if further motion is available. This is necessary only if the patient does not reach full elevation. If the patient has full motion with passive motion but not with active, then it is possible that the shoulder is weak. If the active and passive motions are the same, then it is possible that the shoulder is stiff or that the patient is splinting from too much pain. When patients complain of pain with range of motion, it is advisable not to push too hard and to cause further pain. If it cannot be determined whether the patient is stiff or just experiencing pain, then consideration should be given to injections to decrease the pain. In some instances, the degree of stiffness cannot be determined without an examination under anesthesia.
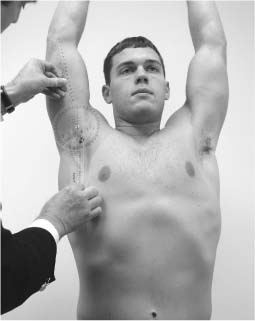
FIGURE 2-13 Abduction is measured with the goniometer centered over the glenohumeral joint.
When measuring elevation of the shoulder, some patients place their arms in a position that will automatically handicap their ability to elevate. The two most common maneuvers will be to try to elevate the arm in the plane of the body (coronal plane) or behind it (Fig. 2-14). Physiologically it is very difficult to lift one’s arm in this position even if the shoulder is normal. The second maneuver that will prevent elevation is attempting to raise the arm in abduction with the thumb or hand down (Fig. 2-15). In abduction, the arm must be in external rotation, or it will not move due to the greater tuberosity contacting the superior glenoid. Sometimes patients with secondary gain issues will attempt to elevate the arm behind the body with the thumb or hand down. It is important to make sure that the arm is brought forward and externally rotated to obtain full appreciation of the ability to elevate the extremity.
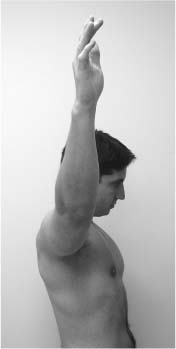
FIGURE 2-14 The arm can be elevated in the plane of the body (coronal plane), which is more constrained than elevating the arm in the scapular plane.
FIGURE 2-15 Full elevation in the plane of the scapula cannot be accomplished with the thumb down because greater tuberosity is restrained by the superior glenoid.
Measuring Elevation in Flexion
Elevation of the arm in flexion can be helpful because it typically is not as stressful to the shoulder as elevation in abduction. This is probably because it does not require much external rotation of the arm. When evaluating flexion of the arm, typically the examiner stands at the side and asks the patient to elevate his or her arm with the palm down (Fig. 2-16). When the patient has gone as far as possible, the goniometer is placed vertically related to the floor, and the center of the goniometer is placed over the lateral aspect of the shoulder joint. The second arm of the goniometer is placed down the center of the arm.
Once this has been done, if full elevation has not been reached, then the arm can be pushed passively into more flexion. This should be done with care, particularly if the patient is experiencing pain. The ability to push the arm into more flexion passively than actively may indicate weakness. If the arm cannot be elevated further passively, then a stiff joint may be the cause. If the pain is severe, then injections may help decrease the pain to see the effect upon motion. In some instances it may be necessary to do an examination under anesthesia to determine if the shoulder is stiff or frozen.
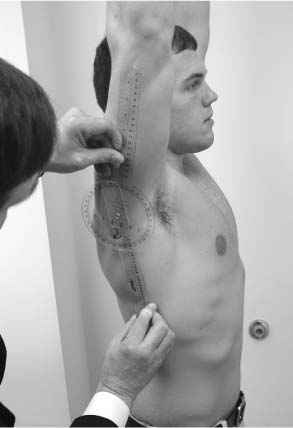
FIGURE 2-16 Flexion of the arm is measured from the side with the goniometer centered as much as possible over the glenohumeral joint.
Measuring Rotational Motions of the Shoulder
Rotational movements typically are measured separately from measures of elevation, but both of these components of shoulder motion have clinical significance. Rotational movements are the result of a complex interaction of the scapulae on the thorax and of the humerus on the scapulae. These interactions between the scapulae and the humerus have important implications for the normal and the impaired shoulder. Rotation of the humerus is necessary not only for placing the arm behind the head but also for placing the arm in a cocked position for sports activities. The relationship between the scapula and humerus also has implications for how we measure motion, how we communicate humeral position relative to the scapula, how we express capsular tightness after surgical procedures, and how we measure the results of surgery.
There has been an explosion of information in the past several years on shoulder rotational movements, primarily as they relate to pain in the shoulder of the overhead athlete. Most patients are not involved in overhead sports, however, and it is important to understand the concepts of measurement of shoulder rotation for individuals from all occupations, avocations, and age groups. This section will address the controversy over measuring rotational movements of the shoulders in athletes, the importance of distinguishing total shoulder complex rotations from purely glenohumeral rotations, and techniques for measuring shoulder rotation.
Rotational Range of Motion and the Overhead Athlete
The issue of the effect of arm dominance on range of motion is important for several reasons, but one of the more salient reasons is that it has been postulated that shoulder pathologies in athletes involved in unilateral overhead sports (e.g., baseball, tennis, and javelin throwing) may be due to alterations in shoulder rotations, particularly rotations in which the arm is elevated 90 degrees. Numerous studies have demonstrated that in overhead athletes there is an increased external rotation compared with the nondominant side, with a concomitant loss of internal rotation (Table 2-6).
This loss of internal rotation in the dominant arm was termed glenohumeral internal rotation deficit (GIRD). It has been speculated that this altered arc of rotation motion resulting in greater external rotation and diminished internal rotation causes abnormal shoulder motions and translations that predispose or contribute to pathological processes in the shoulder of the overhead athlete.53,54 These pathological problems include superior labrum anterior to posterior (SLAP) lesions, secondary impingement, partial rotator cuff tears, internal impingement, and pain.55
There are basically three theories on how these abnormal motions contribute to shoulder pathology in the athlete. The first theory was promulgated by Jobe and colleagues,56–59 who found that baseball players often not only had increased external rotation of the shoulder upon examination but also tended to be loose jointed. This looseness was manifested as increased translation of the shoulder. Jobe et al hypothesized that this increased laxity was exacerbated by placing the extremity in the extreme position of abduction and external rotation, as seen in the throwing motion, or using the arm over shoulder level in some sports.
This position of the shoulder and the forces generated by throwing-type motions of the shoulder contributed to the gradual stretching of the ligaments of the shoulder, particularly the anterior band of the inferior glenohumeral ligament. This caused an occult form of anteroinferior instability, as the humeral head would move too far forward on the socket or glenoid.
This shoulder joint instability was postulated to place extra stress on the rotator cuff and contributed to partial tears of the supraspinatus. This stress also resulted in superior labrum lesions, particularly SLAP lesions. Jobe et al suggested that this contact may be accentuated in patients who have increased laxity of the glenohumeral ligaments. This would result in a “hyperangulation” of the proximal humerus, which was manifested by increased external rotation upon physical examination. The authors recommended a capsular shift to tighten the anteroinferior capsule in patients who demonstrated this combination of findings.60
Another theory of how abnormal motions of the shoulder contribute to pain and dysfunction in the overhead athlete relates to contact of the bones of the proximal humerus with the posterosuperior glenoid when the arm is elevated. This theory is called internal impingement, based on observations by Walch et al61,62 at the time of arthroscopic surgery on the shoulders of patients with pain. While the patient was under anesthesia and with the arthroscope in the shoulder, they noted that with the arm in abduction and external rotation, the greater tuberosity of the humerus and the rotator cuff would make contact with the posterior and superior labrum.
Walch et al suggested that this contact between the humerus and posterosuperior glenoid may contribute to posterior and superior labrum tears and potentially to tears of the rotator cuff, particularly if the contact was frequent, as seen in an overhead athlete. They suggested that this contact would be increased in patients with too much external rotation of the proximal humerus and attempted humeral osteotomies to try to prevent this malady. Unfortunately, the results of this surgical procedure were not as good as hoped, and osteotomy is no longer recommended for treatment of internal impingement.63
The third theory regarding motion and shoulder pathology in overhead athletes suggests that loss of internal rotation contributes to the development of superior labral lesions. The theory suggests that contracture of the posterior capsule causes an obligatory migration of the humeral head superiorly and posteriorly.64 As the arm is placed in abduction and external rotation, this increased shift of the humeral head causes increased contact of the rotator cuff and humerus to the superior labrum, resulting in “peeling off of the superior labrum.65 This “peel back” is an abnormal movement of the labrum off the glenoid rim and theoretically contributes to the shoulder pain experienced by the athlete.
Once the labrum tears, it has been suggested that a superior instability pattern develops where there is too much laxity in the shoulder.66 Because this is not a true anterior and inferior laxity pattern as suggested by Jobe et al,58 this laxity as a result of a SLAP lesion has been called a “pseudolaxity.”53 The postulated treatment of the contracture or loss of motion depends on the degree of pathology. Physical therapy is directed toward increasing external rotation by stretching the posterior capsule. If nonsurgical treatments fail, then surgical treatment includes repair of any SLAP lesions and, in some instances, release of the posterior capsule to increase external rotation.
Several biomechanical studies have been performed in an attempt to see if this postulated sequence of events to the throwing shoulder could actually result in increased forces to the posterior and superior shoulder. Grossman et al67 conducted a study in which they first stretched the posterior capsule of cadaver shoulders. This resulted in an increase in external rotation, as may be seen in a thrower’s shoulder. This also resulted in an increased anterior translation of the shoulders tested. The researchers then performed a plication of the posterior capsule and found that with the arm in a cocked position, there was a posterior and superior shift of the humeral head compared with the normal shoulder.
Koffler et al68 performed a biomechanical evaluation using electromagnetic sensors in cadavers to compare humeral translations in the late cocking phase of pitching. The cadavers were studied by mounting the scapula to a board and removing all tissues except the rotator cuff and capsule. Three-dimensional kinematics were tested with the humerus in neutral, at 90 degrees of elevation, and in a simulated cocking position of throwing. When 20% of the posterior capsule was tightened, the humeral head moved in an anterosuperior direction compared with controls. When 40% of the capsule was tightened, the humeral head moved in a posterosuperior direction compared with controls. Although these findings were descriptive, Koffler et al could not demonstrate a statistically significant difference. They concluded that posterior capsular tightness may put other structures at risk, but this was not proven in their study.
Anderson et al69 also used cadaveric shoulders to induce posterior capsular contracture and reported significant reductions in internal rotation with the arm at the side and at 90 degrees of abduction. This was accompanied by a significant displacement of coupled anteroposterior translation of the humeral head anteriorly (7 mm). Although the head translated differently, the authors did not explicitly comment on the direction, nor did they comment on the effect of force on the shoulder structures of these alterations.
All of these theories depend in some part on accurate measurement of shoulder motion, especially rotations. A recent debate on the amount of diminished internal rotation (GIRD) seen in baseball players and its role in the pathophysiology of shoulder problems suggested that the different measures of arm rotation by different examiners were a critical issue in the evaluation of players, in the development of the theories of what causes the problems in overhead athletes, in the treatment of the problems, and in determining the results of both nonoperative and surgical treatments.70
In that debate, Kibler et al70 suggested that low levels of GIRD (less than 25 degrees) are normal, but that GIRD greater than 25 degrees predisposes the shoulder to injury. In a cohort of baseball players with arthroscopically proven SLAP lesions, Burkhart et al66 reported the GIRD in that cohort averaged 53 degrees, with a range of 25 to 80 degrees. In an unpublished study of 38 athletes with type II SLAP lesions, Burkhart et al66 found an average GIRD of 33 degrees, with a range of 26 to 58 degrees. It should be noted that the technique for measuring range of motion was not specified in these two studies. In contrast, Kibler et al70 reported that, in their evaluation of baseball players, it was rare to have a player with GIRD of over 25 degrees. These differences in opinion between the debaters on the amount of GIRD seen in baseball players may be based on different techniques of measurement of shoulder rotation.
There have been several studies of shoulder motion in overhead athletes that have supported the contention that different methodologies have affected these range of motion measurements. A review of these studies reveals that the results are not consistent between studies. This may be explained by the influence of multiple technical variables, as mentioned in the previous discussion (Table 2-6).
Brown et al71 found that, in 41 asymptomatic baseball players, pitchers had significantly more external rotation with the arm abducted 90 degrees than did position players. They also found that pitchers had decreased internal rotation and increased external rotation of the dominant arm compared with the nondominant arm with the arm abducted 90 degrees. However, in pitchers there was no difference in external rotation between the dominant and nondominant arm with the arm at the side. Chandler et al72 and Kibler et al73 observed similar findings in both elite and professional tennis players. They also found increasing loss of internal rotation with the age of the player, which they speculated may contribute to increased shoulder pathologies with tennis players as they age.
Sauers and Keuter74 studied 103 professional baseball players (49 pitchers and 54 position players) and found that the throwing shoulder exhibited significantly increased external rotation and decreased internal rotation compared with the nonthrowing shoulder. They also found that pitchers exhibited greater external rotation compared with position players.
Bigliani et al75 studied 148 baseball players and suggested that the active shoulder rotations were the same as passive rotations. They found that both position players and pitchers had increased external rotation and decreased internal rotation compared with the nondominant shoulder. Bak and Magnusson76 studied a small cohort of injured (N = 7) and not in pain (N = 8) swimmers and found no significant differences between the two groups in rotations with the arm abducted 90 degrees. They also found no statistically significant difference in rotation between the injured and noninjured shoulders.
Beach and Whitney77 studied 32 competitive swimmers (31% pain-free, 69% with pain) for internal and external rotation of the shoulder. They found nonsignificant changes in the range of motion of the shoulders in the two groups but did not find any relationship between shoulder flexibility and pain. They also found no significant difference between sides for range of motion in their cohort.
Sauers et al78 reported that nonoverhead recreational athletes exhibited significant side-to-side asymmetries for both external and internal rotation. Passive measures with the scapula stabilized were obtained with the subjects supine. Dominant shoulder external rotation was ~5 degrees greater and dominant internal rotation was ~9 degrees less than the contralateral, nondominant shoulder. These data cast further doubt on the ability to make reasonable side-to-side comparisons in the general population.
Rotational range of motion adaptations in overhead athletes have received a great deal of attention in the sports medicine literature. The body of evidence demonstrating that overhead athletes exhibit an increase in external rotation with a concomitant decrease in internal rotation is undeniable; however, it remains unclear exactly what causes these rotational range of motion adaptations in overhead athletes.
The current theories of shoulder dysfunction in overhead athletes summarized above are predicated upon the theory that capsular alterations are the cause of these range of motion changes. For example, Jobe et al’s micro-instability theory suggests that the underlying problem is attenuation of the anteroinferior capsuloligamentous restraints leading to secondary impingement and rotator cuff tears. Burkhart et al’s peel back theory conversely maintains that the underlying problem is posterior capsular contracture leading to altered translational kinematics and eventually to a SLAP lesion (see Chapter 6). Increased external rotation range of motion has been cited as supportive of Jobe et al’s theory of micro-instability, and reduced internal rotation range of motion has been cited as supportive of Burkhart et al’s peel back theory.
There is increasing evidence, however, that some of the alterations in shoulder range of motion seen in athletes may be due to alterations in the shape of the bones. Studies have demonstrated that collegiate and professional baseball players demonstrate changes in both humeral and glenoid retroversion in their throwing shoulder.79–81 These osseous changes are attributed to proximal humerus physeal changes that are thought to occur during the developmental years of young baseball players. The magnitudes of change in humeral and glenoid retroversion are similar to the observed magnitudes of change in rotational range of motion. These studies would suggest that the throwing shoulder is essentially “spun back” into greater external rotation due to these bone alterations. It has been suggested that the observed alterations of rotational range of motion in the overhead athlete are attributed at least in part to osseous changes and are not the result of anterior capsular attenuation and posterior capsular contracture.70
Total Arc of Motion and the Overhead Athlete
Support for the theory that rotational range of motion adaptations are caused at least in part by osseous changes can be found by looking at the total arc of rotational motion. The total arc of motion is the sum of external rotation and internal rotation with the arm at 90 degrees of elevation. Studies of healthy overhead athletes have consistently demonstrated that in spite of the observed changes in motion in the dominant shoulder (i.e., increased external rotation and decreased internal rotation), the total arcs of motion when compared between sides are nearly identical.74
Numerous studies have compared the total arc of motion between the throwing and nonthrowing shoulders of baseball players and have consistently reported bilateral symmetry of total motion (Table 2-6). These studies have been interpreted by some to mean that for every degree of external rotation gained, there is 1 degree of internal rotation lost, subsequently maintaining a symmetric total arc of motion. According to this idea of a maintenance of total range of motion to 180 degrees, if there were osseous changes such as increased humeral retroversion, then this would create symmetric changes in range of motion based on the mechanics of rotating a rigid bar. Thus, if the humerus is spun back 10 degrees into external rotation, then there would be a mandatory decrease in internal rotation of an identical magnitude in the absence of any soft tissue changes. Unfortunately, this concept that the total arc should be maintained has not been proven, and it is unknown whether reciprocal changes are necessary for normal shoulder function in overhead sports. In addition, it is currently unknown how much of total motion is altered by soft tissue changes and how much by bone changes.
Asymmetry between sides in the total arc of motion has been implicated as a causative factor in the development of shoulder pathology in overhead athletes. Wilk et al82 stated that the total arc of motion should be symmetric within 10 degrees of motion; that is, the throwing shoulder should not gain or lose more than 10 degrees of total arc of motion. They refer to this as the “total motion concept,” whereby side-to-side asymmetry may predispose the throwing shoulder to injury.
Morgan83 referred to the “180 degree rule,” where the shoulder can safely lose 1 degree of internal rotation for every degree of external rotation gained; however, when the shoulder loses more internal rotation than is gained in external rotation, the shoulder is at risk of developing injury. We are not aware of any prospective studies evaluating the relationship between the total arc of motion and injury in the overhead athlete.
It is of interest that not all overhead sports lead to the same changes in rotational range of motion. Studies of the total range of motion in baseball players have repeatedly shown that the healthy throwing shoulder demonstrates increased external rotation, decreased internal rotation, and a symmetric total arc of motion; however, range of motion studies in tennis players have consistently reported a decrease in the total arc of motion in the dominant shoulder. Ellenbecker et al84 studied pain-free elite male and female junior tennis players and found that both genders exhibited similar range of motion changes. External rotation was similar between the dominant and nondominant shoulders, but the dominant shoulder exhibited a significant decrease in internal rotation. As a result, the dominant shoulder exhibited a significant decrease in the total arc of motion compared with the nondominant shoulder.
Kibler et al73 reported similar findings in elite male and female tennis players, except they found significantly greater external rotation in the dominant shoulder compared with the nondominant shoulder; however, the deficit in internal rotation exceeded the external rotation gain, and this resulted in a diminished total arc of motion in the dominant shoulder. Both the Ellenbecker et al and Kibler et al studies reported a 10 degree or greater loss of the total arc of motion in the dominant shoulder of tennis players. Similar to baseball players, tennis players are unilateral overhead athletes; that is, their overhead shoulder activity is predominantly performed by their dominant shoulder; however, the range of motion adaptations between these two sports appear quite different. We are not aware of any studies that have reported on the humeral and glenoid version of competitive tennis players. At this time, it is unclear if range of motion in tennis players is the result of osseous changes, soft tissue changes, or both.
In contrast to the unilateral nature of baseball throwing and tennis, swimmers use both arms in their sport, albeit not always symmetrically. It may therefore be expected that swimmers exhibit different range of motion adaptation patterns than athletes in sports where one arm is clearly used more than the other overhead. Like unilateral overhead athletes, swimmers exhibit increased external rotation with a concomitant decrease in internal rotation.76,77,85
Studies of range of motion of the shoulders of swimmers, however, have demonstrated that this shift occurs in both shoulders, as opposed to the unilateral change observed in the dominant shoulder of baseball and tennis players. As a result, the total arc of motion is symmetric between shoulders in healthy swimmers. Bak and Magnusson76 reported a symmetric total arc of motion in elite swimmers with and without shoulder injury. We are unaware of any studies reporting on the normal values of humeral and glenoid versions in swimmers. It is unknown whether the forces in swimming are large enough to result in osseous adaptations and whether the observed motion changes in swimmers are the result of capsular adaptations.
As a result of these studies, rotational range of motion adaptations in the overhead athlete should be considered normal to a certain degree. It is clear that no one factor may be responsible for determining the absence or presence of associated shoulder pathology. Similar to the issue of increased glenohumeral joint laxity, changes in rotational range of motion in the overhead athlete should not be considered problematic unless they are correlated to symptoms.
There are significant differences in the absolute values for shoulder rotations reported in all of these studies (Table 2-6). In many of these studies the conditions of the measurements are not defined, such as whether the subject is sitting or supine. Few of the studies mention who performed the measurements, and in many studies the measurements are performed by more than one examiner. Some of the studies stabilize the scapula, but it is not specified if the measurements were performed with or without stabilization of the scapula in all positions studied.
Future studies of shoulder range of motion must clearly identify the conditions under which the measurements were taken. Most importantly, studies must identify if the measurements obtained were of glenohumeral motion (i.e., the scapula was stabilized) or of shoulder complex motion (glenohumeral and scapulothoracic motion). As discussed in a later section of this chapter, studies have reported significant differences between shoulder range of motion with the scapula stabilized and unstabilized.27,38,86 Range of motion measurements should always be obtained bilaterally, and the total arc of motion should be compared between shoulders.
When measuring range of motion of the shoulder for GIRD, it is important to note whether glenohumeral motion, scapulothoracic motion, or both are being measured.
When measuring range of motion of the shoulder, it is imperative to measure internal rotation and external rotation bilaterally and to compare the total arc of motion.
Distinguishing Glenohumeral from Scapulothoracic Motion in Rotation
We noticed in our clinic that when examining rotational motions of the shoulder in patients, there was a distinct end point that occurred prior to full, combined rotational motion of the shoulder complex. After the first end point, there was increased resistance to arm movement. This increased resistance was found to correspond to subtle motion of the scapula and sometimes the patient’s body. This motion not only could be felt but also could be seen with visual cues.
Because we typically measured passive rotations of the shoulder in the office, we speculated that the first end point was that created by reaching the end of the excursion of the glenohumeral ligaments with the arm in the given position. This end point most likely represented an interaction of the bony anatomy of a particular specimen, but the first end point was felt to represent the end of the excursion of the ligaments under most tension in a given arm position. It has been demonstrated that the primary restraint to external rotation with the arm at the side is the middle glenohumeral ligament.87 With the arm abducted 90 degrees, the primary restraint to external rotation is the inferior glenohumeral ligament.88
Using our cadaveric model, we tested the hypothesis that glenohumeral motion can be distinguished from scapulothoracic motion in shoulder rotations. Our goal was to establish the validity of the concept of measuring glenohumeral motion only versus combined glenohumeral-scapulothoracic motion. To test this hypothesis, we used five cadaver shoulders instrumented with electromagnetic sensing devices. The senior author (EGM) externally rotated the shoulder until the first end point was reached and stopped moving the shoulder. Visual cues included motion of the shoulder or thorax, which indicated scapular motion was imminent.
The position of the bones in relationship to each other was calculated. Motion was then resumed until the second end point was reached. This second end point was the maximum motion possible and represented the total glenohumeral and scapulothoracic motion. Scapular motion was detected by the sensor, and the relationship of scapular motion to the first end point was calculated. The difference between the first end point and the beginning of scapular motion was felt to represent the error in estimating the beginning of scapular motion with the first end point.
The external rotation with the arm at the side of the cadaver shoulder to the first end point was 35.4 degrees (Table 2-7). On average, the difference between the first end point and the beginning of scapular motion was 1.2 degrees of humeral motion, which means that the examination accurately predicted glenohumeral motion within 1.2 degrees on average. With the arm abducted 90 degrees, when measuring glenohumeral external rotation, scapular motion was detected within 2 degrees. When predicting glenohumeral internal rotation, the scapular motion was predicted within 1.0 degree. The glenohumeral internal rotation was only 0.7 degrees, which was consistent with our clinical observations that isolated glenohumeral rotation is limited in adults.
When examining shoulder rotation, this experimental model demonstrates that glenohumeral motion can be distinguished from scapulothoracic motion.
This study validates the concept that upon examination, isolated glenohumeral motion can be distinguished from scapulothoracic motion for the rotations measured. In all three motions tested the glenohumeral motion was found to be less than the combined motions (Table 2-7). The results of this study also suggest that most measurements of shoulder rotations reported in the literature may be of combined scapulothoracic and glenohumeral motions, including those reported by the AAOS in 1964 (compare Tables 2-1 and 2-6). The next step was application of these concepts to the examination of human subjects.
To incorporate new knowledge of shoulder biomechanics and motion |
To create a consistent measurement system between observers |
To report accurately tightness of the glenohumeral joint from the scapulothoracic articulation |
To be able to report accurately progress with rehabilitation |
To be able to measure accurately motion before and after surgical procedures |
To be able to identify specific structures that are responsible for loss of motion |
To measure motion accurately and consistently in overhead athletes |
Clinical Studies of Glenohumeral Motions in Rotation
The preceding biomechanical model suggests that glenohumeral motion can be determined independently of scapulothoracic motions in rotations. The clinical implications of this distinction are important for anyone who treats shoulder conditions. There have been many occasions in treating patients with frozen shoulders when therapists reported excellent external rotation of 75 degrees when in fact the real glenohumeral motion is 10 degrees. It is important that reliable measurements be used for outcome studies and for calculating disability ratings. It is also important to distinguish glenohumeral from combined motion when testing concepts of GIRD for evaluating treatment of losses of internal rotation (Table 2-8).
The distinction between glenohumeral motion and combined motions has significance for the postoperative treatment of shoulder surgery patients. It is important that the therapist or trainer know exactly how much external rotation can be safely allowed in the postoperative period. After surgical correction of the instability, if the repair becomes tight at 20 degrees of external rotation, the therapist and surgeon must both know if that motion is glenohumeral or combined motions. If the therapist believes it is combined motions, then the patient could become quite stiff if protected in that range during the first 6 weeks after surgery. If the surgeon dictates that 30 degrees of external rotation was achieved in the operating room, and the therapist takes that to be combined motions when it is actually glenohumeral motion, then the patient will become stiff. This is especially true in the postoperative treatment of patients who have had a subscapularis detachment and repair as part of their surgical procedure. If there is a miscommunication, the patient may have the repair stressed prematurely or stressed too slowly. This distinction is also important when comparing results of surgical procedures that may affect shoulder rotation, such as capsular shifts or Bankart repairs.
We sought to evaluate in a clinical study whether the distinction between glenohumeral motion and scapulothoracic motion could be performed reliably and reproducibly. Twenty volunteers with no history of shoulder problems underwent informed consent as part of an Institutional Review Board (IRB)-approved study and were examined by the senior author (EGM) and a physical therapist (SF) instructed in the preceding technique of measuring shoulder motions.
The subjects were clothed in short-sleeved shirts and measured at two separate occasions 4 weeks apart. A handheld goniometer with 1-degree gradations was used, and motion was recorded to the nearest degree. The motions measured were external rotation with the arm at the side and with the arm abducted 90 degrees; internal and external rotations were measured. The measures were performed only once during each trial. The subject’s arm was passively moved until the first end point was observed. This involved a tactile feeling when rotating the arm, and also involved watching for rotation of the shoulder complex independent of the glenohumeral joint. Next, the arm was rotated as far as tolerated by the subject, and it was held there while the rotation was measured. The subjects were not allowed to use lumbar lordosis to increase the measure.
The measures were repeated with the patients supine. No attempt was made to restrict scapular motion with any of the shoulder measurements with the subject in either the standing or supine positions. This is different than the suggested technique, where the examiner places a hand under the shoulder to feel for scapular motion.
The results of the measurements are reported in Table 2-9. The isolated glenohumeral motion was significantly different from the combined motion for all three arm positions. This study substantiates that there are important differences between glenohumeral and scapulothoracic motion that can have clinical implications.
Isolated glenohumeral motions are less than combined motions in rotation and can be accurately determined upon clinical examination.
The interobserver reliability of the measurements was also studied. The correlations between examiners for all measures for both trials and both subject positions (i.e., supine and standing) are reported in Table 2-10. These data indicate that there is good to excellent agreement between examiners except for glenohumeral internal rotation at 90 degrees of elevation. Repeatability of the measures for each examiner was good to excellent when comparing trials with both examiners for both subject positions.
This study found a significant difference between the motion measurements between the dominant and non-dominant shoulders. The dominant-side shoulder (all subjects were right handed) was found to have increased glenohumeral external rotation and combined external rotation compared with the nondominant side (Table 2-11).
This study also examined the influence of the standing versus the supine position for these measurements. All of the shoulder measurements (both glenohumeral and combined) were significantly different between the standing and supine positions. The measurements were consistently larger with the subject measured in the supine position (Table 2-12). This finding seems to indicate that it is more difficult to detect scapular motion when the patient is supine. Also, there were significant differences between the surgeon and the physical therapist for most measures supine and standing. Because the therapist was not experienced at this examination, this finding suggests that detection of scapular motion can be a challenging skill to acquire.
The findings of our study are generally in agreement with a similar study performed by Boon and Smith38 at the Mayo Clinic. They measured glenohumeral motion and combined motions in 50 high school athletes with two groups of physical therapists who repeated the measurements 5 days later. They used handheld goniometers and measured passive rotations of the shoulder with the subject supine. The scapula was stabilized by one therapist by holding the shoulder stable with a hand on the coracoid and clavicle. Glenohumeral motion was taken to be the first end feel. The scapula was released, and then total rotation (no scapula stabilized) was measured, which was an indication of combined glenohumeral and scapulothoracic motion. External rotation at the side was not measured.
Boon and Smith found significant differences between glenohumeral and combined motions for all three positions tested (Table 2-13). There was a statistically significant increase in external rotation in the dominant arm over the nondominant arm and a decrease in internal rotation compared with the opposite side; however, there was no difference in the motions between athletes involved in overhead sports compared with those not involved in overhead sports.
The researchers found that the intraobserver or intrarater reliability was good for all measures except for combined or nonstabilized internal rotation; however, the interrater or interobserver reliability for internal rotation was poor for the stabilized position (interclass correlation coefficient of 0.13), but it was slightly better when the scapula was stabilized (ICC = 0.38). For external rotation, the intrarater reproducibility was better for both the stabilized (ICC = 0.58) and unstabilized (ICC = 0.79) scapular measurements. This was similar to our data, which showed that the ICC for interobservor or interrater reliability for external rotation at the side for glenohumeral motion was 0.52 and for combined motions was 0.74. The interrater ICC in our study for external rotation with the arm abducted 90 degrees was 0.57 for glenohumeral motion and 0.81 for combined motions. In our study the interrater ICC for internal rotation with the arm at 90 degrees of abduction was 0.28 for glenohumeral motion and 0.74 for combined motions.
We agree with Boon and Smith’s speculation that the interrater differences were due in part to the inexperience of one of the examiners with the technique. In addition, they examined the patients supine, which tends to make detection of scapular motion less reliable. The use of tactile and visual cues may increase the sensitivity of detection of scapular movement.
When reporting shoulder motion, the examiner should designate if glenohumeral or combined motions are being measured.
In our study it was felt that the differences in the supine and standing measures were because the beginning of scapular motion was difficult to detect with the patient supine. This observation was supported by Awan et al,27 who studied the effect of attempting to stabilize the scapula versus the use of tactile and visual cues for measuring internal rotation of the shoulder. They examined 56 athletes supine using three techniques to measure internal rotation. The first measurement was full internal rotation with the arm abducted 90 degrees without the scapula stabilized, which averaged 91 degrees on the right and 100 degrees on the left. With the scapula stabilized, which should be a measure of only glenohumeral motion, the measures of internal rotation averaged 63 degrees on the right and 70 on the left.
Awan et al measured isolated glenohumeral internal rotation with the arm in the same position by looking for visual cues of the shoulder lifting off the table, which meant that the scapular motion was beginning. They found that the measurements were lower than for the other three techniques, with an average of 60 degrees on the right and 70 degrees on the left. Although the measures using the visual method and the scapular stabilized methods were not significantly different, they were both significantly lower than the measurements when the shoulder blade was allowed unlimited motion (i.e., combined glenohumeral vs combined glenohumeral and scapulothoracic movement). The researchers concluded that the visual method was at least as good as traditional methods of measuring isolated glenohumeral internal rotation and that it had the advantage of needing only one examiner.
Visual observation of movement of the scapula and shoulder girdle in conjunction with feel for the end point is a sensitive technique for the measurement of isolated glenohumeral rotations.
Awan et al27 also noted a learning curve when beginning to use visual cues as part of the evaluation of shoulder motion. This is similar to our experience when teaching this technique to students and residents. When measuring glenohumeral and combined motions, it is recommended that the patient be standing and that the shoulders be exposed. This allows for visual cues that the scapula is moving. When the first end feel is reached, the scapula will typically become engaged and begin to migrate. This can be felt by the examiner, the patient’s trapezius will begin to elevate, or there may be a subtle motion of the thorax.
Learning the techniques for measuring glenohumeral versus total shoulder combined rotations takes practice and attention to subtle shoulder movements.
There are several inherent limitations of goniometric measurements that should be considered when distinguishing scapulothoracic from glenohumeral motion. When examining any joint, ligament elongation occurs as the end of joint excursion is reached. Thus an end feel may have an elastic portion that actually covers several degrees of motion. Also, when measuring combined motions, the amount of scapular motion can be affected by the flexibility of the scapula on the chest wall and by how hard the examiner pushes.
These factors may explain why the measurements obtained for isolated glenohumeral motion in our study differ from those reported by Boon and Smith.38 Also, there is a discrepancy in the rotations measured in our cadaver model compared with the measurements obtained in living human subjects in our clinical study and in Boon and Smith’s study. This could be due in part to the age of the cadavers (average age 76.3 ± 6.6 years)12 in our study compared with the subjects average age 22 ± 3 years in our study (Table 2-9; unpublished data) performed on healthy volunteers.
We have measured glenohumeral motion and combined motions for all patients undergoing shoulder surgery since 1998. These data can be evaluated by decade and by diagnosis. The range of motion of the affected arm of patients by diagnosis and type of motion measured are reported in Table 2-14. The results for the unaffected extremity by decade are reported in Table 2-15. It can be seen that isolated glenohumeral motion is less than combined scapulothoracic and glenohumeral motion for rotations regardless of decade.
There has been one study evaluating the concept that glenohumeral motion can be distinguished from glenohumeral motion in overhead athletes. The study by Sauers et al86 of 28 professional baseball pitchers was performed and compared isolated glenohumeral joint and total shoulder rotational range of motion.
This study involving professional baseball players demonstrated significant differences in motion between the isolated glenohumeral and the combined glenohumeral and scapulothoracic measurements both for internal and external rotation. The difference between glenohumeral external rotation and combined motions in external rotation averaged 25 degrees, for isolated glenohumeral internal rotation and combined internal rotation 23 degrees, and for the total arc of motion between the two sides 48 degrees (Table 2-6). Sauers et al86 found that the combined motions and isolated glenohumeral motion in both internal and external rotation were not different between the dominant and nondominant extremities.
Although not conclusive, the findings of this study suggest that the range of motion alterations in the throwing shoulder are predominantly the result of adaptations occurring at the glenohumeral joint. This study supports the contention that for research and clinical documentation in this population, it is important to differentiate between isolated glenohumeral joint motion and combined glenohumeral and scapulothoracic motions upon examination.
Measuring Shoulder Rotations
A brief description of the techniques for measuring shoulder rotations will be given here, but the practitioner is encouraged to understand the rationale for each measurement as described earlier.
Standing Examination
The patient should be undressed so that the entire shoulder complex can be visualized. This is important because slight movements of the scapula on the thorax cannot be seen, and rotation of the body cannot be seen without being able to see the upper body. When examining the shoulder with the patient standing, there are three possible measurements. The motion used depends on the information needed by the examiner both at that examination and upon subsequent examinations. The first movement that can be tested is active motion of the shoulder in rotation, and this measurement is of total or combined glenohumeral and scapulothoracic motion. The patient essentially moves the arm in rotation in the specified position until he or she cannot move it any further. The examiner measures and reports that motion while checking that the patient is holding the position without leaning or moving the arm out of position.
The next two possible measurements are passive measures of rotation. The first is passive glenohumeral rotation, where the first end point is established. The second measure of passive motion is of combined glenohumeral and scapulothoracic motion. Which of these two measures is used depends on the clinical situation and the needs of the examiner. We have found that isolated glenohumeral rotations are more important in detecting glenohumeral joint stiffness than measures of combined motion. In addition, it is important to distinguish glenohumeral motion from combined motions when examining athletes who have motion limitations.
External Rotation Standing with the Arm at the Side
The best way to perform this measurement is with the patient standing, but some patients may need to be measured sitting. It is important that the shoulders be exposed to observe any motion of the shoulder or thorax. We believe that the visual cues and tactile cues are necessary for accurate examination of glenohumeral motion. The patient should be relaxed and pain-free. Two examiners may be necessary in unique situations. To begin, the subject is asked to place the arms in a neutral position, with the elbows bent 90 degrees (Fig. 2-17). Three different measurements are possible.
I. Passive Isolated Glenohumeral External Rotation with the Arm at the Side
If the examiner is looking for glenohumeral rotation alone, then he or she supports the patient’s elbow with the hand and passively externally rotates the arm until the first end point is reached (Fig. 2-18). Hints that the end point has been reached include tightness at the shoulder, but also that the shoulder girdle will be seen to elevate as the scapula is engaged. Another sign that the glenohumeral motion has been reached is that the body will start to turn or the chest will start to turn. The measurement can then be made with a goniometer by asking the patient to hold that position, or a second observer can hold the arm in that position until the measurement is made. It is important that the arms of the goniometer are aligned with one along the long axis of the forearm and the other parallel to the floor.
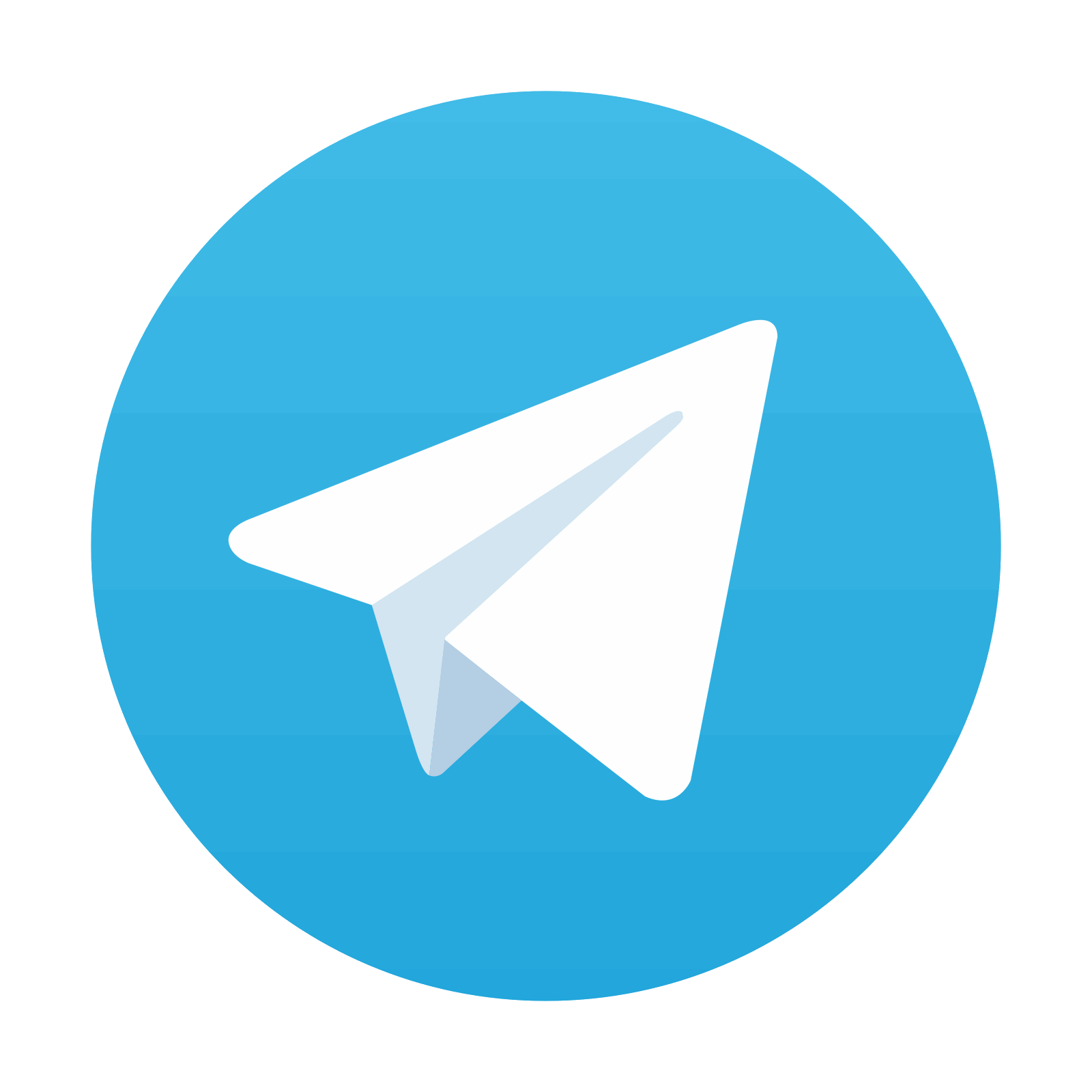
Stay updated, free articles. Join our Telegram channel

Full access? Get Clinical Tree
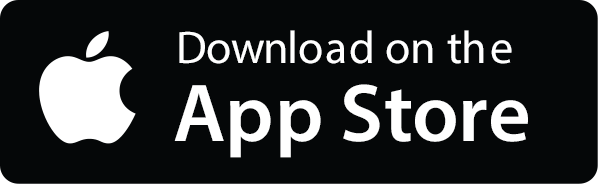
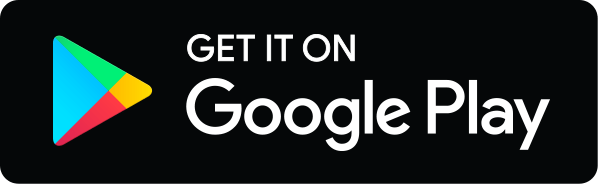