Pressure Ulcers
Chester H. Ho
Kath Bogie
SCOPE OF THE PROBLEM
Definition
Tissue necrosis and breakdown is referred to by many terms including decubitus ulcers, bedsores, ischemic sores, and pressure sores. The term “pressure ulcer” is the most accurate nomenclature to describe both the cause and nature of soft tissue wounds, caused primarily by excessive applied pressure. This term will be used throughout this chapter. A critically important precursor to appropriate care is an accurate identification and classification of a soft tissue wound as a pressure ulcer. This definition has recently been updated by the National Pressure Ulcer Advisory Panel (NPUAP) to be a localized injury to the skin and/or underlying tissue usually over a bony prominence, as a result of pressure, or pressure in combination with shear and/or friction (1). This change takes into account an increasing body of evidence that pressure ulcers are generally multifactorial in origin. Furthermore, pressure ulcers are frequently associated with deep tissue injury that can be difficult to detect on initial examination, particularly in individuals with darker skin tones. The NPUAP has, therefore, also updated the guidelines for staging in order to provide clinicians with a standardized and reliable framework for classification (see “Assessment” below). Appropriate and effective care of pressure ulcers is essential in order to minimize further medical complications that can include amyloidosis, osteomyelitis, septicemia, and even death.
Economic Implications
The presence of one or more chronic pressure ulcer has a significant negative impact, both on the individual’s health status and on the overall health care system. The total economic impact is hard to quantify. The most recent figures in the United States date from 1999 when the treatment costs were estimated to be in excess of $1.3 billion annually (2). More recently, in 2004, Bennett et al. (3) found that the annual cost of treating pressure ulcers in the United Kingdom was approximately 4% of the total health care expenditure (1.4 to 2.1 billion GBP), equivalent to around $4 trillion per annum in February 2008. This major trend for increasing costs may continue as the overall population at risk for chronic wounds increases with changing demographics, in particular an increasing aged population with reduced mobility. Cost containment will require continuous improvements in clinical care for early identification, prevention, and treatment of pressure ulcers. Lyder et al. (4) found that implementing comprehensive prevention programs can significantly reduce the incidence but that costs are not significantly reduced. Prevention intervention strategies should, therefore, be based on risk stratification.
Incidence and Prevalence
Pressure ulcers are a very common cause of morbidity and even mortality. No central registry exists and many pressure ulcers may go unreported, leading to higher rates of both morbidity and mortality (5). Incidence and prevalence vary widely, both between studies and across different health care settings. For example, in the United States the prevalence of pressure ulcers in acute care hospitals has been reported to be between 3% and 69%, with annual incidence rates of 1% to 29% (6, 7, 8, 9, 10, 11). More recent studies in Swedish and Canadian acute health care settings have found the overall prevalence rates of 29% to 33% (12,13).
In the U.S. long-term care facilities and nursing homes, prevalence rates from 1% to 35% have historically been noted, with annual incidence rates of 10% to 38% (14, 15, 16). Brandeis et al. reported that pressure ulcer development was associated with a 4.5 times greater risk of death for elderly nursing home residents than for those who did not have pressure ulcers but had the same risk factors (17). The prevalence of pressure ulcers among the outpatient population has not been well-defined, although studies have reported similarly varying rates (18).
These figures should be viewed cautiously; some variations, particularly in smaller studies with lower reported incidence rates, may be due to factors such as pressure ulcer definition, study population characteristics, and data source, for example, retrospective chart review or self-report (19). For example, in some studies over 45% of reported pressure ulcers were classified as Stage 1, whereas in others Stage I pressure ulcers were not included. Although Stage I pressure ulcers may not appear clinically severe, and are generally readily treatable, it has been found that these ulcers are an important warning of potential future deterioration (20), and they should therefore always be taken seriously.
Although there is paucity of recent, reliable, and widely available data for U.S. health care facilities, there is a growing assumption, particularly reflected in regulatory and quality management guidelines, that the development of any pressure ulcer is evidence of negligent care by a health care provider or health care system (21). Increasing public awareness has provided the perception that pressure ulcers are preventable
and readily treatable. The Centers for Medicare & Medicaid Services (CMS) guidelines mandate that any resident who enters a nursing home without a pressure ulcer must not develop pressure ulcers unless the individual’s clinical condition is such that they were “demonstrably unavoidable” (22). Any resident who has a pressure ulcer must receive necessary treatment to promote healing, prevent infection, and prevent new ulcers from developing. Both federal and state surveyors ensure compliance with all aspects of the mandate. Penalties for noncompliance and/or violations can be severe, including fines, loss of financial support, and even immediate closure of the facility. This environment dictates that all clinical practitioners be highly educated in all aspects of pressure ulcer care for their patients.
and readily treatable. The Centers for Medicare & Medicaid Services (CMS) guidelines mandate that any resident who enters a nursing home without a pressure ulcer must not develop pressure ulcers unless the individual’s clinical condition is such that they were “demonstrably unavoidable” (22). Any resident who has a pressure ulcer must receive necessary treatment to promote healing, prevent infection, and prevent new ulcers from developing. Both federal and state surveyors ensure compliance with all aspects of the mandate. Penalties for noncompliance and/or violations can be severe, including fines, loss of financial support, and even immediate closure of the facility. This environment dictates that all clinical practitioners be highly educated in all aspects of pressure ulcer care for their patients.
ANATOMY AND PATHOPHYSIOLOGY: NORMAL SKIN
The skin is the largest organ in the integumentary system, the external covering of the body. It has multiple functions, including protection of the body from trauma, dehydration, and microorganisms; thermoregulation, excretion of waste products through perspiration, and sensory perception. The skin also produces vitamin D through exposure to ultraviolet light. Therefore, it is vital to our health and damage to the skin may have potentially serious consequences.
There are two main layers to the skin: the epidermis and the dermis. There are subcutaneous tissues underlying the dermal layer. The dermis is about four times the thickness of the epidermis and consists of collagen fibers, blood vessels, lymphatic vessels, sweat glands, hair roots, and sensory nerves. Embryologically, this layer is derived from the mesoderm. The main function of the dermis is to support the epidermis, and provide strength and elasticity to the skin. There are two main layers in the dermis, the superficial papillary dermis and the deeper reticular dermis. The papillary dermis consists of loose connective tissues, capillaries, and elastic fibers, while the reticular dermis consists of dense connective tissues, elastic fibers, larger blood vessels, fibroblasts, mast cells, sensory nerves, and lymphatic vessels. This layer is essential for blood supply and sensory perception. The subcutaneous tissues lie between the dermis and the underlying muscle and bone. They consist of adipose tissues and their main function is to provide insulation and storage of nutrients.
The blood supply of the skin comes from the underlying structures, supplying the various structures in the skin along the way, and is crucial for thermoregulation. The blood supply from the bone travels into the skin, supplying the muscles, fascia, nerve, and connective tissues. The blood vessels in the skin anastomose and form a horizontal network of blood vessels in the dermis. Blood flow to the skin is very high and allows effective heat dissipation from the skin through vasodilatation of the blood vessels. Conversely, vasoconstriction in the skin will lead to diminished blood flow and thus reduced heat loss and a cool skin surface.
WOUND HEALING
Normal physiological wound healing is a complex, dynamic process that can be defined as comprising four main phases: hemostasis, inflammation, proliferation, and tissue remodeling (23,24) (Fig. 52-1). Inflammation can be further divided into early and late inflammation. Similarly, proliferation consists of both granulation tissue and extracellular matrix (ECM) formation. The entire sequence of events exhibits substantial overlap of the various phases, with each phase characterized by different predominant cell types.
Immediately following injury, the healing response begins with the activation of the coagulation cascade and the creation of a blood clot (25), producing hemostasis and preventing further blood loss (26). Various chemotactic factors, including platelet-derived growth factor (PDGF), stimulate the migration of blood neutrophils and monocytes, helping to initiate the second phase of healing, the inflammatory response (27).
Inflammation is responsible for containing, neutralizing, diluting, or walling off the injurious agent or process (28). Acute inflammation lasts only minutes to days depending on the extent of injury as leukocytes, predominately neutrophils (28), invade the wound and clear it of debris (25,27). As the neutrophils diminish, the wound moves to the chronic inflammation phase with increased activity of macrophages and lymphocytes. Macrophages produce several biologically active enzymes and cytokines, including collagenases that phagocytose cell debris, senescent neutrophils, and devitalized tissue in the wound region. This debrides the wound and promotes wound healing. Interleukins and tumor necrosis factor (TNF) concurrently promote angiogenesis and stimulate fibroblasts activity (28), leading to the wound transitioning to the proliferative phase.
Granulation tissue formed during the proliferative phase of healing provides a temporary weak tissue layer in the wound. Granulation tissue is so called because of the pink, soft granular surface appearance (27, 28, 29). New blood vessels are produced by budding or sprouting from pre-existing vessels in a process known as neovascularization or angiogenesis (28).
Angiogenesis is essential for granulation tissue formation and improves the overall tissue perfusion (30). Another key aspect of the proliferation phase is reepithelialization: Migration and proliferation of keratinocytes from the wound edges leads to the formation of a thin epithelial layer over the developing granulation tissue (27). In a full thickness, nonsutured wound the rate of reepithelialization is thus inversely related to the wound size. Wounds left uncovered and open to the air develop a layer of eschar, or scab, over the granulation tissue. Although this layer of dried wound exudate and dead cells protects the wound from environmental contamination, it also prevents keratinocyte migration so that reepithelialization cannot occur. Reepithelialization may thus be accelerated by preserving an optimally moist environment.
Angiogenesis is essential for granulation tissue formation and improves the overall tissue perfusion (30). Another key aspect of the proliferation phase is reepithelialization: Migration and proliferation of keratinocytes from the wound edges leads to the formation of a thin epithelial layer over the developing granulation tissue (27). In a full thickness, nonsutured wound the rate of reepithelialization is thus inversely related to the wound size. Wounds left uncovered and open to the air develop a layer of eschar, or scab, over the granulation tissue. Although this layer of dried wound exudate and dead cells protects the wound from environmental contamination, it also prevents keratinocyte migration so that reepithelialization cannot occur. Reepithelialization may thus be accelerated by preserving an optimally moist environment.
Collagen repair starts in the proliferative phase and extends into the final remodeling phase. Remodeling of the ECM occurs as granulation tissue is reduced via apoptosis (31). Collagen is constantly being synthesized and degraded in order to gain strength through the reorientation of collagen fibers, as wound tissue approaches full strength (25). Collagen deposition in normal wound healing reaches a maximum by 21 days after the wound is created (27); however, it may take up to 2 years or more for a scar to completely form. If the remodeling process is disrupted, the equilibrium between ECM deposition and degradation is lost, leading to the development of fibrosis and excessive scarring (32). Scar tissue contains fewer cells than normal skin and never achieves the same tensile strength as the original skin it has replaced (27,29).
SITES OF PRESSURE ULCERS
Pressure ulcers can develop in any region of the body where regional risk factors are increased (see “Pressure Ulcer Pathophysiology”), and most frequently develop where soft tissue coverage over bony prominences is reduced. Common sites of pressure ulcer development will vary depending on the most prevalent posture. For example, bed-bound patients will predominantly be lying supine (Fig. 52-2A); thus, the occiput, sacrum, and heels are the highest risk regions. Mobilized wheelchair users will spend most of the time sitting and the highest risk regions will then be the ischia, sacrum, and heels (Fig. 52-2D).
PRESSURE ULCER PATHOPHYSIOLOGY
The term “pressure ulcer” may be misleading in that it implies that pressure is the only risk factor. In fact, the characteristic tissue breakdown and a subsequent failure to heal are well recognized to be multifactorial processes. For example, there is increasing evidence that shear forces are critically important. Clinical evidence regarding risk factors has been mostly correlative rather than causative (33), although animal models and in-vivo studies are providing further details. It can be useful to consider risk factors broadly as either extrinsic, that is, external to the patient’s body, or intrinsic, that is, within the patient’s body (Table 52-1). Psychosocial factors are complex and probably can be classified as both intrinsic and extrinsic.
TABLE 52.1 Risk Factors in Pressure Ulcer Development | ||||||||||||||
---|---|---|---|---|---|---|---|---|---|---|---|---|---|---|
|
Thorough consideration of the risk factors is of vital importance because they contribute to the development of treatment and rehabilitation strategies. In general, extrinsic risk factors respond more rapidly to clinical interventions, such as changing the seating system used, but this is not always feasible or easily optimized, for example, intraoperative pressure relief. Changes in clinical status can alter intrinsic factors and increase the risk of pressure ulcer development. For example, urinary incontinence will alter the microenvironment of the skin surface and make it more susceptible to maceration and breakdown.
Given these risk factors, it is not surprising that certain populations are especially prone to pressure ulcer development. These include the elderly with reduced mobility, hospitalized individuals with complex medical conditions, those with cognitive impairments, and those with motor and sensory impairment such as spinal cord injury.
Pressure
Applied isostatic pressure compresses the soft tissues equally along all axes and causes little or no tissue damage. However, in most real-life situations, applied pressure is not isostatic and the soft tissues will compress along the axes of loading. As applied pressure increases the local capillaries, deeper vasculature will become progressively occluded, impeding blood flow. Lymphatic circulation will be impaired, reducing local drainage of intracellular waste materials and increasing the risk of edema.
The soft tissues can be defined as a composite viscoelastic material, in other words, the response to applied loads varies with the duration of application. Relatively high loads can be tolerated for a short period of time, with complete elastic recovery on removal of the load. Over longer periods of applied load, the viscous nature of the soft tissues predominates, leading to irreversible tissue damage and increased full recovery time for reversible damage. Specific times and magnitudes of critical applied loads vary individually, dependant on the resilience of the local tissues, which is, in turn, dependant on intrinsic factors (34) such as those described in this chapter.
Removal of applied loads after a period of vascular occlusion results in a transient increase in regional blood flow. The duration of this phenomenon, known as reactive hyperemia, is directly related to the preceding duration of occlusion. During the hyperemic phase, tissue damage can occur due to oxygenfree radical activity, which is incompletely buffered in tissue under oxidative stress (35, 36, 37). This has led to the proposal that repetitive ischemia/reperfusion injury is a significant pathway to the development of chronic pressure-related wounds. Animal studies have found that repetitive ischemia/reperfusion causes more severe tissue damage than a single, prolonged ischemic insult (38,39). The response to cyclic loading varies for metabolic and myogenic components of skin blood flow, suggesting that optimization of alternating pressure support surfaces requires personalized evaluation of intrinsic blood flow (40).
Many clinicians have observed that patients may appear to have a minor wound or even only some localized discoloration, which then rapidly breaks down to reveal extensive subcutaneous damage, even down to and including the bone. Such wounds are the result of deep tissue injury, that is, tissue breakdown that has its origin in the soft tissues nearer to the bone rather than to the skin surface. Theoretical models using finite element analysis have indicated that peak pressures under applied load occur at the bone-muscle interface (41) and that the magnitude is strongly dependant on the local geometrical and biomechanical characteristics (42). Recent human studies have shown that internal tissue loads are significantly higher in individuals with paraplegia compared to age-matched able-bodied individuals (43), and that postural changes significantly affect these loads. These methods have yet to be applied to other at-risk populations, such as the elderly; however, similarities in tissue biomechanical properties would be predicted.
Shear and Friction
Shear force is defined generally as a force or a component of force acting parallel to the plane. For a patient, shear forces can originate from nonuniform intrinsic pressure distributions, surface shear, or tangential forces at the body/support interface and cause transverse tissue distortion, that is, shear stresses and strains. Although it has been found that shear force alone does not appear to induce tissue breakdown (44), shear in combination with normal applied pressure will occlude blood vessels at much lower applied forces than normal applied pressure alone (45). Shear strains within the tissue can also disrupt tissue layers. Shear is thus widely considered to be the most important risk factor because blood flow occlusion and tissue damage occurs much more rapidly when shear stresses and strains are present. In recognition of this, the NPUAP convened the “Shear Force Initiative” in 2005 (46). To date, this group has not issued specific guidelines and the direct measurement of shear forces in clinical practice remains challenging.
If shear forces are overcome, slip will occur between the two surfaces. This friction may result in both epidermal abrasion and subepidermal blistering (47,48). Friction alone may not cause pressure ulcers, but in combination with other risk factors such as a compromised microenvironment, it would appear to heighten the risk of rapid tissue damage.
Immobility
Impaired mobility is commonly due to paralysis or other concurrent medical conditions, leading to an increased risk of pressure ulcer development, especially in the hospital setting (49,50). Motor paralysis will directly affect a person’s ability to respond unconsciously to potential noxious stimuli, for example, fidgeting while sitting or turning while asleep. Reduced mobility also profoundly alters the individual’s ability to consciously perform postural maneuvers necessary to relieve prolonged applied pressure, from weight shifting while sitting to walking. The loss or reduction of mobility may be further
complicated by sensory impairment, leading to the absence or alteration of normal perception of environmental stimuli such as pain or temperature. Patients with impaired sensation and/or proprioception are at an increased risk of pressure ulcer development because the individual cannot sense the warning signals that precede tissue damage.
complicated by sensory impairment, leading to the absence or alteration of normal perception of environmental stimuli such as pain or temperature. Patients with impaired sensation and/or proprioception are at an increased risk of pressure ulcer development because the individual cannot sense the warning signals that precede tissue damage.
Nutrition
Impaired nutritional status is another significant risk factor for the development of pressure ulcers (51,52). Moreover, the malnourished patient will also have an impaired response to healing. Protein depletion may lead to decreased perfusion and impaired immune response. Low serum protein level may be associated with interstitial edema, leading to decreased tissue elasticity, hence reducing tissue transportation of oxygen and nutrients from the blood to the skin (53). Increased tissue pressure as a result of interstitial edema may decrease blood flow to the skin, thus increasing the risk of pressure ulcer development (54). Furthermore, the presence of an exuding pressure ulcer may cause protein loss and the patient may then develop negative nitrogen balance.
Nutritional status can be measured by various means: food intake, body weight, body mass index (BMI), serum total protein, albumin, and serum prealbumin. Serum total protein level less than 6.4 g/dL has been associated with the development of pressure ulcers (10). Serum albumin level less than 3.5 mg/dL has also been associated with pressure ulcer development (55). Serum prealbumin has a shorter half-life than serum albumin, and therefore, is a more sensitive indicator for measuring nutritional status. The Mini Nutritional Assessment (MNA) and Mini Nutritional Assessment Screening Form have been found to be advantageous in the screening of nutritional status in elderly people with pressure ulcers (56,57). The severity of a pressure ulcer can be directly related to the degree of hypoalbuminemia (58). Fluid balance must also be considered in conjunction with nutritional status since dehydration will decrease the cellular nutrient delivery.
Local Microenvironment
The local environment at the skin-support interface, termed the microenvironment, has a localized effect on the risk to pressure ulcer development. This umbrella term covers several factors including temperature, moisture, and acidity.
Skin temperature provides an indicator of tissue perfusion. It has been found that immobile patients do not recover preloading skin temperatures after a period of prolonged loading (59). However, elevated body temperatures will raise metabolic activity increasing the need for cellular oxygen. Animal studies have found that deep tissue damage occurs at 10° above room temperature whereas even higher temperatures caused both cutaneous and subdermal damage (60). In some patient populations, such as those with SCI, dysfunctional temperature regulation may produce abnormal variations in microrenvironmental temperature.
Moisture from sweat or incontinence will both superhydrate the epidermis and alter the acidity. Skin maceration will both soften the stratum corneum and increase the friction coefficient of the epidermis. The net effect is rapid destruction of the epidermis and reduced antibacterial functionality (61). Both sweat lactate and urea concentrations become elevated with prolonged ischemia (62, 63), implying that sweat analysis may be a useful predictive indicator of early tissue damage and pressure ulcer development.
Anemia
Anemia has been identified as a risk factor in both the general (64) and the spinal cord literature (65,66). Hemoglobin level below 12.0 to 14.0 g/dL has been associated with the development of pressure ulcers (67). The literature suggests a correlative relationship, but presumably anemia will lead to impaired delivery of oxygen and nutrients to the local tissue, affecting both the development and the healing of pressure ulcers.
Impaired Vascular Status
Impaired vascularity of the soft tissues is increasingly recognized as a risk factor for the development of pressure ulcers. Tissue vascularity can be impaired as a result of different pathophysiology, for instance, the impairment of microcirculation due to diabetes mellitus (68), and ischemia to deep tissues as a result of compression or pressure. In fact, there is increasing evidence that deep tissue injury is a significant risk factor in the development of pressure ulcers, especially in conjunction with other risk factors (69). This concept is further discussed elsewhere in this chapter.
Muscle Atrophy
Muscle atrophy is a common consequence of aging and neuromuscular conditions, such as spinal cord injury and stroke. It may be due to muscle denervation, or simply due to disuse. Individuals with muscle atrophy are particularly at high risk of developing pressure ulcers because it leads to an increase in the interface pressure between the weight-bearing skin areas and the support surfaces. For instance, the gluteal muscles provide cushioning effect between the ischial tuberosity and the seating surface. Gluteal muscle atrophy following spinal cord injury will increase the interface pressure between the ischial skin area and the support surfaces such as the wheelchair cushion or the mattress. When this problem is compounded by other risk factors such as immobility, it puts the individual at high risk of pressure ulcer development.
Psychosocial/Lifestyle
Some psychosocial factors and lifestyle can be readily addressed by clinicians; however, there is often a need to address non-clinical problems (70). For example, smoking cessation and substance abuse programs can improve the overall physical and mental health. Other issues, such as familial support, access to specialized health care, and independence of community mobility impact social integration. Effective management requires a multidisciplinary team approach.
RISK FACTOR MEASUREMENT
Many of the risk factors described in the previous section can be evaluated using standard clinical procedures, such as blood work to determine the nutritional status. Quantitative measurement of specific pressure ulcer risk factors, such as interface pressures and blood flow, require more specialized approaches.
Interface Pressure Mapping
Interface pressure mapping has become more widely used as an assessment tool in wheelchair and seating clinics (71,72). Patients with complex seating needs and those requiring repeated evaluations routinely receive interface pressure assessments as part of the review of both posture and function. Several commercially available systems are reliable, accurate, repeatable, and user-friendly. All these systems include a pressure sensor mat placed between the patient and the support surfaces. This mat is thin and flexible in order to minimize disruption of the interface being measured and contains an array of sensing elements (sensels) that are rapidly scanned to complete a detailed image of the regional pressure distribution. The resulting pressure map is displayed in real-time on a computer screen and provides clinical information, such as location of high pressure points. In addition, real-time pressure maps can be used as biofeedback for patients, who can see the effects of various cushions and weight-shifting maneuvers.
Blood Flow Measurement
The measurement of interface pressures alone is not sufficient: Maintenance of tissue health requires an adequate supply of nutrients from the blood. Blood flow measurement is thus important for quantitative identification of pressure ulcer risk. Various noninvasive techniques have been used to measure blood flow under applied load.
Transcutaneous blood gas electrodes measure the partial pressure of oxygen and/or carbon dioxide in the skin. Localized heating ensures maximal vasodilation so that capillary blood gas tension is equal to arterial tension. Multisensor systems allow the simultaneous measurement of several at-risk locations. Transcutaneous blood gas measurement has been used in studies to determine both pressure ulcer risk (63,73,74) and healing (75,76).
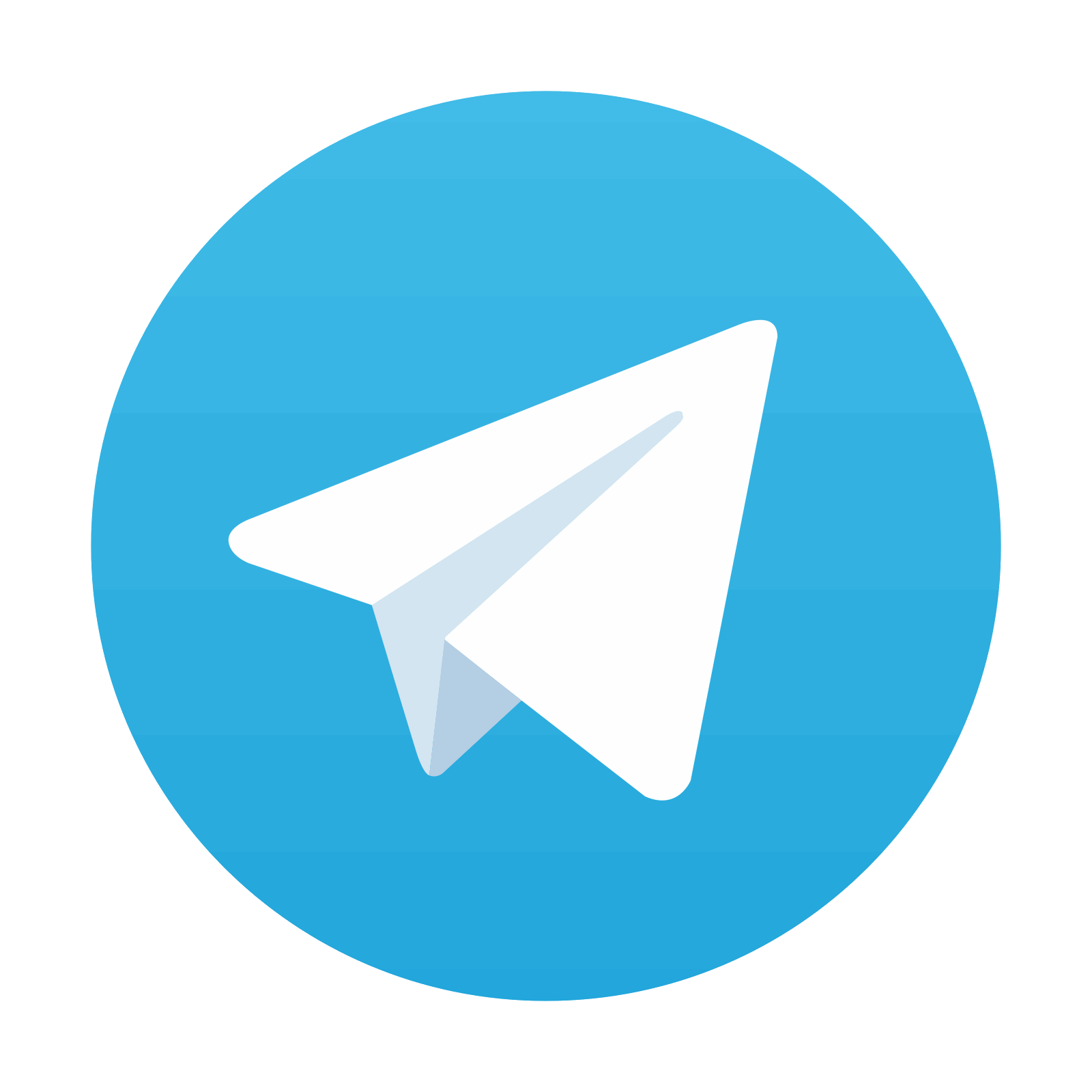
Stay updated, free articles. Join our Telegram channel

Full access? Get Clinical Tree
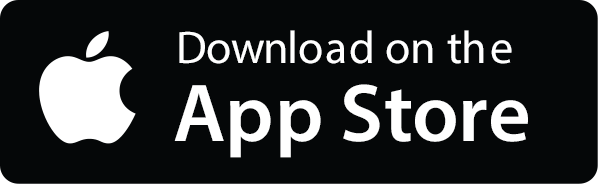
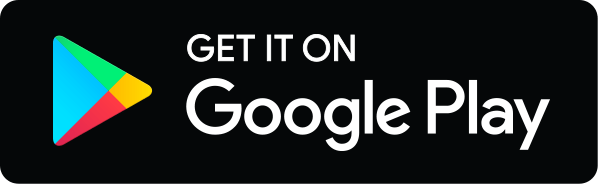