!DOCTYPE html PUBLIC “-//W3C//DTD XHTML 1.1//EN” “http://www.w3.org/TR/xhtml11/DTD/xhtml11.dtd”>
Periprosthetic Osteolysis
INTRODUCTION
Since the introduction of low-friction total hip arthroplasty by Sir John Charnley over four decades ago, millions of arthritis patients have experienced substantial pain relief and enhanced quality of life. Although advances in surgical technique, implant design, implant fixation, and antibiotic prophylaxis have contributed to short- and long-term success for the majority of patients, the challenge of achieving long-term durability for many others can be limited by their own biological response to particulate debris.
Osteolysis refers to the process of bone resorption around either stable or unstable joint replacement prostheses.1,2 Charnley3 recognized this source of radiographic and clinical failure but felt that the process was related to culture-negative infection. Subsequent observers attributed the process as a reaction to polymethylmethacrylate (PMMA) cement,4,5 leading to the development of cementless implant technology. However, in spite of the elimination of PMMA with cementless reconstruction, the problems of aseptic loosening and focal osteolysis were not resolved.6–11
Over the past 20 years, the culmination of clinical and laboratory research has implicated particulate debris as the inciting cause and macrophage-mediated activation of osteoclastic bone resorption as the mechanism of both linear (aseptic loosening) and focal osteolysis. Although these two osteolytic variants bear different radiographic appearance, the underlying pathophysiology is currently understood to result from the same process.12 The recognition of the central role of particulate debris in the osteolytic process has directed interest into both alternative bearing surfaces and into enhancing the wear resistance of polyethylene in an effort to limit the generation of submicrometer-sized particles. Although preliminary results are encouraging, the effectiveness of these alternative bearing surfaces will not be fully delineated for several years.
Within the context of revision total hip arthroplasty, understanding the process of osteolysis is important for planning appropriate surgical approaches for both linear osteolysis and clinically significant focal osteolysis around either well-fixed or loose implants. Preoperative and intraoperative assessment of implant fixation and periprosthetic bone support combine with surgical judgment in selecting the most appropriate reconstructive approach for each individual patient.
The aim of this chapter is to outline the pathophysiological processes that result in osteolysis, to describe the clinical and radiographic presentation of both linear and focal osteolysis, and to delineate surgical approaches that will most predictably address the underlying osseous deficiencies.
PATHOPHYSIOLOGY
Generation of Particulate Debris Particulate debris can be generated by either mechanical wear or corrosion. General processes of mechanical wear include adhesion, abrasion, and fatigue.13 These mechanisms can occur at the articular bearing surface, the fixation interfaces, or from additional devices incorporated to enhance fixation (e.g., screws, wires, claws). Corrosion can occur between dissimilar materials,14 at modular component interfaces (e.g., Morse taper),14,15 or between implants and additional fixation devices.16 The types of particles that have been identified within periprosthetic membranes obtained at revision surgery reflect the materials used during the initial reconstruction: polyethylene, PMMA, titanium, cobalt-chromium, silicates, and stainless steel.17–21
McKellop et al.22 described the mechanical conditions underlying the generation of particulate debris as modes of wear. Mode 1 refers to expected wear that occurs at the primary articular bearing surface. Mode 2 wear occurs when the primary bearing contacts a secondary surface in an unintended fashion (e.g., femoral head penetration through a thin polyethylene liner). Mode 3 refers to third-body, abrasive wear. Mode 4 wear occurs between two nonbearing surfaces. Examples of mode 4 wear include backside wear, impingement of the Morse taper against the acetabular shell, and micromotion at modular connections or at the stem-cement interface.
In the majority of appropriately aligned total hip replacements, the most significant source of wear should be mode 1, between the articular bearing surfaces.17 McKellop et al.22 estimated that as many as 500,000 submicrometer particles of polyethylene can be generated during each gait cycle. In failed total hip arthroplasties, either mode 1 wear rate may accelerate or additional modes of wear may contribute significantly to the generation of particulate wear particles. Motion at the interface between modular acetabular components,23–27 counterface abrasions,28 and generation of abrasive particulate debris22 have been associated with premature radiographic and clinical failure. Maloney et al.29 identified an average of 1.7 billion particles per gram of tissue within periprosthetic granulomas around loose cementless components in contrast to 143 million particles per gram of tissue around a control group of primary total hip replacements. In this study, the average size of particles reported was 0.5 µm for polyethylene and 0.7 µm for metal debris with 90% of all particulate debris <0.95 µm.29 Horowitz et al.30 demonstrated that PMMA particles <0.7 µm are generally retained within macrophages and induce cytokine production.
A number of factors have been identified that can contribute to increased volumetric wear. These include polyethylene component thickness <8 mm,31 inferior polyethylene quality,32 polyethylene machining,33,34 material weakness from sterilization processes,35,36 and prolonged shelf life.37 The use of titanium femoral heads38 and larger head size (32 mm)39 have also historically been noted to produce greater particulate debris. Recent studies have identified the importance of acetabular component positioning, particularly the influence of abduction angles >45 degrees, on increased polyethylene wear.40 The majority of these contributing factors can be recognized and controlled by the surgeon performing primary total hip arthroplasty. Optimizing component position and minimizing potential sources of impingement, abrasion and third-body wear are best managed at the time of initial joint replacement surgery.
Access of Particles to Bone-Implant Interface In 1992, Schmalzried et al.41 introduced the concept of the effective joint space. Particles produced as a product of mechanical wear or corrosion circulate through the joint during normal gait. Resorption of these products occurs from the capsular tissue into the reticuloendothelial system,14,42,43 and may provide limited protection from the local effects of these particles on the periprosthetic tissues. However, excessive generation of particulate debris can result in saturation of the reticuloendothelial system and circulation of substantial particulate debris within the joint fluid. Defects in the interface between implant and host bone can allow particulate debris to be pistoned into the host bone where the cascade of osteolytic histochemical interactions can occur.44–46
Conditions that allow increased access of joint fluid to the periprosthetic interface include cement mantle defects, screw holes in the acetabular shell, noncircumferential porous coating around components, and failure of biological fixation.
Biological Response to Particulate Debris At the level of the implant-bone interface, wear particles interact with cells within a fibrovascular periprosthetic membrane. The principal cell responsible for phagocytosis, the macrophage, responds to ingestion of particulate debris with the production of collagenase and prostaglandin E2 (PGE2)47,48 as well as cytokines (IL-1a, IL-1b, IL-6, and TNF-alpha)49–58 that may either act upon or influence osteoclastic function to cause bone resorption adjacent to the periprosthetic granuloma. While direct release from macrophages is probably the main source of cytokine production,30,59,60,61,62,12,58,20,21,63 additional cell types (e.g., fibroblasts) can respond to particulate debris and may indirectly stimulate macrophage cytokine production.64,65 Although these studies support a role for immune system in modulation of the macrophage response to particulate debris, this concept has not been universally accepted.12
The proposition that aseptic loosening was a macrophage-initiated response to particulate wear debris was initiated by Willert and Semlitsch.63,66 Retrieval studies performed from revisions for aseptic loosening of cemented acetabular components identified the presence of a macrophage-laden fibrous tissue interface between the cement and bone.4,18,19,67,68 Santavirta et al.69 noted the presence of predominantly giant cells and macrophages in the membrane taken from radiographically aggressive osteolytic lesions around failed cemented hip implants. Histochemical staining of this tissue interface around loose cementless acetabular components identified macrophage migration, adherence, and phagocytosis in response to particulate titanium and polyethylene.70 Staining for IL-2 receptors was negative, suggesting that the response to particulate debris more closely resembles a foreign body response than an immune system–mediated response.70 The identification of mRNA production in macrophages and not in fibroblasts during in situ hybridization studies with IL-1b provided further support to the proposed central role of the macrophage in the direct response to wear debris.53
Goldring et al.47 identified the production of PGE2 and collagenase from periprosthetic granuloma tissue placed in organ culture. Subsequent studies have shown other potentially significant histochemical mediators including gelatinase, stromelysin, metalloproteinases, prostaglandins, and inflammatory-mediating cytokines (IL-1a, IL-1b, IL-6, and TNF-a) around loose total hip arthroplasty components.49–58 The exact importance of each of these potential contributors in the osteolytic process has not been defined. Merkel et al.71 demonstrated that genetically engineered mice deficient in TNF-alpha receptors were protected from particle-induced bone resorption as occurred in normal mice controls.
As noted earlier, periprosthetic tissues around loose implants have been associated with higher concentrations of particulate debris.29 Significantly higher levels of PGE2 and IL-1 have been noted around loose femoral components, suggesting that these mediators are active in the process of osteolysis.51 Goodman et al.72 noted greater numbers of macrophages and T lymphocytes in periprosthetic tissues associated with combined loosening and osteolysis when compared to well-fixed stems or cemented stems with loosening alone. This study also noted increased levels of IL-1 and IL-6 containing cells associated with osteolysis in comparison with well-fixed prostheses.72 Membranes around loose bipolar components have been noted to produce greater amounts of PGE2 than around loose total hip arthroplasties.51 The conclusion that osteolysis occurs in response to particulate debris was further substantiated by the finding of similar levels of chemical mediators regardless of whether components were cemented or not or whether the implants were manufactured from titanium or cobalt-chromium.73
Other studies have demonstrated that the response of macrophages to different particulate substances is not uniform. Haynes et al.74 noted that particles composed of cobaltchromium have a more cytotoxic effect on macrophages than titanium alloy particles; and, while cobalt-chromium particles had a limited ability to stimulate release of inflammatory mediators, titanium particles stimulated significant release of both PGE2 and cytokines. Horowitz et al.59,60,75 demonstrated that submicrometer (<0.5 µm) PMMA particles inhibit DNA synthesis while Shanbhag et al.62 reported less toxicity from ultrahigh molecular weight polyethylene (UHMWPE) compared with PMMA particles. Catelas et al.76 reported greater release of TNF-a in response to polyethylene in comparison with ceramics. The effects of particle-mediated toxicity on macrophages have also been shown to be both dose-dependent and influenced by particle size and shape.62,77
Studies assessing the direct exposure of peripheral blood monocytes to titanium alloy, commercially pure titanium, membrane-retrieved UHMWPE, and fabricated UHMWPE demonstrated greater production of PGE2, IL-1a, IL-1B, TNF-a, and IL-6 in response to the titanium particles than UHMWPE particles.74,78 This observation correlates from the higher rate of clinical failure when 32 mm titanium heads were coupled with conventional polyethylene as a bearing surface. Macrophage-mediated osteoclast activation likely resulted from both titanium wear debris particles and UHMWPE from accelerated volumetric polyethylene wear against the larger femoral head.
Horowitz et al.75 noted the importance of particle size on cytokine production in response to PMMA debris. Particles small enough for phagocytosis (<0.7 µm) stimulated macrophage activation and TNF-a release, while larger particles did not. Gelb et al.50 using a rat model, noted that small, irregularly shaped PMMA particles (<20 µm) generated a greater inflammatory response than did larger (50 to 350 µm) particles. Kubo et al.79 using a rabbit model, noted a greater reaction around polyethylene (<11 µm), cobalt-chromium (0.03 and 3.9 µm), titanium (0.03 µm), and stainless steel (3.9 µm). Lesser reaction was seen around larger polyethylene and titanium alloy particles and alumina ceramics. Although a number of particulated materials have demonstrated the potential to stimulate macrophage response, conventional ultrahigh molecular weight polyethylene (UHMWPE) has demonstrated the greatest proclivity to result in osteolytic bone resorption and has become a principal subject of investigation.14,22,24,29,32,39,41,50,54,66,80–82
In general, the biological response to wear debris is directly dependent on particle size, composition, and concentration.12,17,77 However, the diverse spectrum of severity in clinical and radiographic findings may very well be influenced by other factors. Mechanical stability of the fixation interface between implant and bone normally limits the extent of the effective joint space. In the absence of stability, access of particulate debris to the periprosthetic bone interface is possible. Bone density and nutrition may affect the capacity of an individual to achieve durable fixation at the bone-implant interface. Additional genetically determined factors theoretically could influence individual susceptibility to osteolysis, although no specific predisposing traits have been identified.12 The ability to identify, target, and modify internal host responses to particulate wear debris is of particular interest to potentially limit or prevent the development of osteolysis.
PRESENTATION
Radiographic Presentation Because the majority of patients with osteolysis and aseptic loosening will be asymptomatic at the time of initial radiographic findings, most reconstructive surgeons recommend routine serial radiographic assessment in order to identify patients early in the process of osteolysis when the residual bone stock is more optimal and the technical demands of revision surgery are likely to be more readily manageable.
The radiographic patterns of osteolysis are influenced by component location, implant stability, and access of particulate debris to the periprosthetic bone.12 The use of cemented versus cementless implants affect the pattern of wear particle access to the surrounding bone, and accounts for differences in radiographic appearance between failed cemented and failed uncemented reconstructions. It is also important to appreciate that the amount of bone loss identified at revision surgery typically exceeds the severity predicted from the appearance of plain radiographs.
Cemented Acetabular Components The path of particulate debris access around cemented acetabular components occurs between the cement mantle and underlying acetabular bone. This accounts for the progressive linear radiolucencies that have been noted around failed cementless acetabular cups. In an autopsy retrieval study, Schmalzried et al.41 noted that subchondral bone reconstitutes and forms a partial barrier to the access of particulate debris. In this setting, expansile lesions do not typically develop, because focal access to the underlying trabecular bone of the pelvis is limited. However, progressive destruction of the cement-bone interface, particularly in the superior and medial aspects of the acetabulum can lead to significant bone deficiency and superomedial component migration. As the component migrates, expansile lesions may also be noted in the ischium.83
Cemented Femoral Components The path of particulate access around cemented femoral components can occur at either the bone-cement or cement-implant interfaces. In comparison with cemented acetabular components, the surface area for particulate access to the proximal femur at the bone-cement interface is more limited.45 Although periprosthetic bone resorption can occur, it is typically confined to the proximal femur (Gruen zones 1 and 7) and does not typically result in femoral component loosening. However, debonding of the implant from the cement mantle can occur and several authors have demonstrated the potential for a passage to form at the cement-stem interface.9,84 Defects in the cement mantle occurring either due to technique or fracture may allow particulate debris to communicate with the periprosthetic bone as the debonded stem cycles within the cement mantle. The developing osteolytic regions may gradually compromise implant fixation.85,86
Improvements in the quality of the cement mantle between first and second generation cement techniques have been associated with a lower rate of linear osteolysis.87,88 The debonding of roughened femoral stems inserted with third generation cement technique has been associated with accelerated rates of linear and focal osteolysis in recent studies.89–91 Presumably, both access of polyethylene debris to the periprosthetic interface and increased production of PMMA debris played a role in the early clinical and radiographic failures noted in these studies.
Definite loosening of a femoral component is indicated by component subsidence or change in alignment, fracture of the cement mantle, fracture or deformation of the implant, or the development of a new radiolucency at the cement-metal interface.92–94 The presence of a circumferential radiolucency suggests probable loosening, while radiolucency around 50% to 99% of the component suggests possible loosening.93 Over time, radiolucencies associated with linear osteolysis are progressive.95
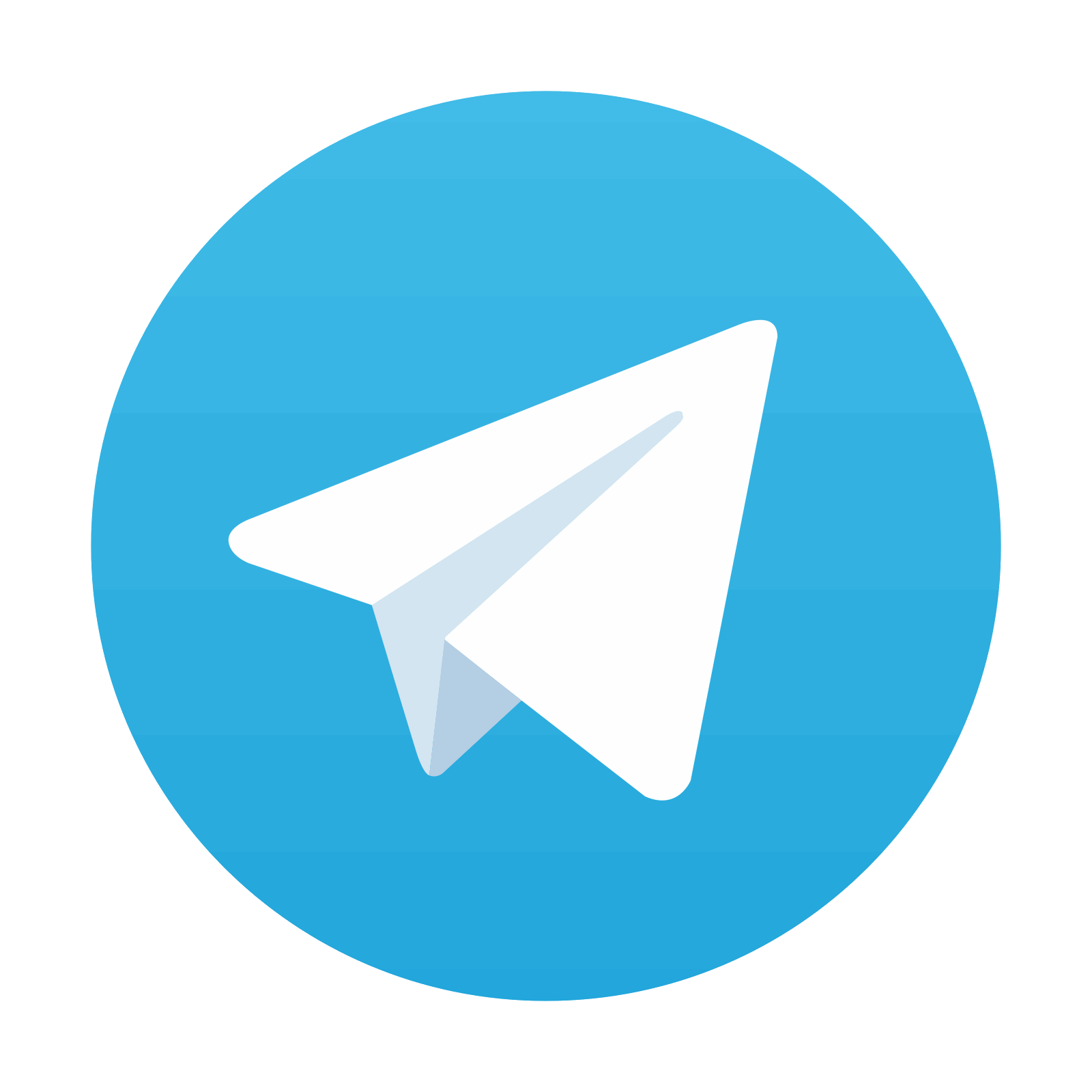
Stay updated, free articles. Join our Telegram channel

Full access? Get Clinical Tree
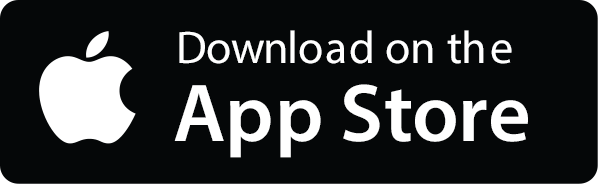
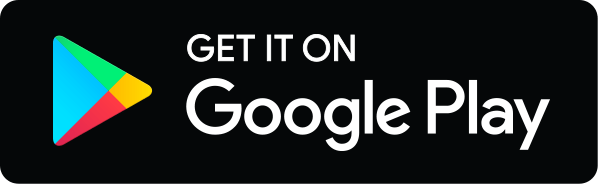