Neurophysiology and the Upper Cervical Subluxation
Kirk Eriksen
Learning Objectives
After studying this chapter, the reader should develop an understanding about:
Anatomy of the spinal cord and related structures
Connective tissue attachments to the spinal Dura mater in the upper cervical region
Concepts related to spinal cord tension and the Dentate ligament–cord distortion Hypothesis
Location and function of somatosensory receptors (i.e., Mechanoreceptors, muscle spindles, Golgi tendon organs, Nociceptors)
Proprioceptive pathways (i.e., dorsal column–lemniscal system, spinocerebellar, and cuneocerebellar tracts)
Pain pathways through the anterolateral system and neurological pain inhibition
Trigeminal nerve, the trigeminocervical nucleus, and Trigeminal neuralgia
Thalamic summation and integration
Role that the cerebellum and vestibulospinal pathways play in neurological integration
Upper cervical afferents synapse with brainstem nuclei
Importance of alignment integrity over motion (e.g., Adjustment vs. Manipulation)
Spinal cord compression and nerve root irritation
Neuroanatomy and physiology related to the upper cervical region is exceptional as the upper cervical cord represents a transitional area between the brainstem and subjacent spinal cord levels. In this chapter, models of upper cervical Subluxation will be reviewed that demonstrate the vulnerability of the upper cervical spinal cord to neurological insult and the potential impact of the chiropractic Adjustment on the Health of patients. The upper cervical region also has the greatest amount of Mechanoreceptive afferentation in comparison with caudal regions of the spine. Cervical articular dysfunction (i.e., Subluxation) has the potential of creating Dysafferentation into the Neuraxis, which can result in various adverse concomitants. This chapter will help explain many of the clinical signs (i.e., postural and functional pelvic distortion, paraspinal thermal asymmetry, muscle spasm, specific pain syndromes) doctors of chiropractic observe in patients.
Spinal Cord Anatomy
The spinal cord is an elongated cylindrical mass of nervous tissue that is the continuation of the medulla oblongata. It extends from the superior border of the atlas vertebra to the upper border of the second lumbar vertebra, and its length measures 40 to 45 cm in most adults. The spinal cord is as long as the vertebral canal until the third month of fetal life. Thereafter, the vertebral column elongates faster than the spinal cord, until the time of birth, when the cord extends to about the level of the third lumbar vertebra. The spinal cord is surrounded by three membranes: Dura mater, arachnoid mater, and Pia mater. The outermost membrane is the Dura mater. It is a tough, fibrous, tubular sheath that extends to the level of the second sacral tubercle. The epidural space separates the dura from the vertebral neural canal and contains loose areolar tissue and venous plexuses. The subdural space is a potential thin area between the Dura mater and the arachnoid that contains sufficient fluid to lubricate the two membranes. The arachnoid forms the middle layer of the three membranes, and it is a system of supporting fibers forming a weblike structure. The subarachnoid space lies between the arachnoid and the Pia mater, and it contains cerebrospinal fluid. The Pia mater intimately adheres to the spinal cord, and it is a highly vascular layer.
The structure of the spinal cord is made up of an outer band of white matter, which uniformly surrounds an inner core of gray matter. However, the size of the white and gray matter varies between segments of the cord. The white matter consists primarily of myelinated nerve fibers grouped in bundles. The gray matter consists of large and small neurons, their dendritic processes, and the numerous synapses between the nerve cells. This region is shaped like a butterfly whose wings are called “horns,” and it is divided into three functional zones: dorsal horns (sensory zone), ventral horns (motor zone), and the middle zone, which carries out associated functions between the sensory and motor zones.
Spinal Cord Tension
Epidural Attachments
The Dura mater of the upper cervical spinal cord is directly attached to the circumference of the Foramen magnum, to the second and third cervical vertebrae and by fibrous slips to the posterior longitudinal ligament. Hinson and Zou1 noted dense fibrous connective tissue slips binding the dura to the atlas posterior arch all the way around to the posterior margin of the lateral mass in all specimens (Fig. 15-1). They only observed variations in the density of the Epidural attachments. The Dura mater was found to be so firmly adherent to the atlas by these attachments, that the authors found it difficult to separate the dura from the posterior arch without tearing the dural tissue.2 The atlas posterior arch constitutes most of the posterior and lateral aspects of the neural canal, and the axis dens represents the anterior margin, whereas the lateral masses are located in the anteriolateral aspect of the neural canal at this level. It is possible that most anatomy texts have ignored the Epidural attachments to the atlas because they do not appear to have surgical significance. However, this chapter will provide compelling evidence for how these anatomical structures play a potential role in the transfer of deleterious tension to the spinal cord as a result of upper cervical Subluxations.
Grostic3 has also described dissection evidence showing numerous strong attachments of the spinal dura to the atlas lateral masses, the atlas posterior arch, as well as the lateral and posterior walls of the axis neural canal. Epidural connective tissue below the upper cervical spine has been described as connecting the posterior spinal dura to the ligamentum flavum.4,5,6 The ligamentum flavum is a strong ligament that connects the laminae of the vertebrae and serves to protect the spinal cord and neural elements, as well as stabilize the spine to prevent excessive motion between the vertebral bodies. Along with the vertebral laminae, this ligament forms the posterior wall of the spinal canal.
![]() FIGURE 15-1 Epidural attachments shown here as dense fibrous connective tissue slips binding the dura to the atlas posterior arch and the axis lamina. (Courtesy of Dr. Roger Hinson) |
Craniale Durae Matris Spinalis Ligament
In 1929, von Lanz7 described the ligamentum craniale durae matris spinalis (CDMS ligament) as fibrous strands between the Dura mater and the posterior border of the atlanto-occipital joints, the edge of the Foramen magnum, the atlas posterior arch, and the base of the spinous process and laminae of the axis (Fig. 15-2). Lang8 also discussed the CDMS ligament, although he failed to mention the connection between the dura and the arch of axis. Rutten et al.9 confirmed the attachments between the ventrocaudal side of the atlas posterior arch and the spinal dura. They also further described the CDMS complex as consisting of strands arising from the ligamentum flava between C1-C2 and C2-C3, although no connections were found below C3. These researchers were credited with first discovering median fibers of the CDMS ligament being continuous in the deep part of the nuchal ligament. Humphreys et al.10 have also reported connective tissue attachments from the base of the axis
spinous process and from the atlas posterior arch to the posterior cervical Dura mater.
spinous process and from the atlas posterior arch to the posterior cervical Dura mater.
![]() FIGURE 15-2 Hemisected head and neck specimen with brain and spinal cord removed. Posterior part of the dural sac has been pulled ventrally. A: Vertebral artery. B: RCPm muscle. C: axis. D: RCPm muscle attachment to Dura mater. E: Dura mater. F: Craniale durae matris spinalis fibrous strands. G: Ligamentum flavum. H: Epidural space. I: Suture material. (Modified from Rutten HP, Szpak K, van Mameren H, et al. Letters. Spine 1997;22(8):924.) |
Rectus Capitis Posterior Minor Muscle and Ligamentum Nuchae Attachments to the Spinal Dura Mater
The rectus capitis posterior minor (RCPm) muscle and the posterior atlanto-occipital (PAO) membrane extend from the atlas posterior arch to the occipital bone. Furthermore, the PAO membrane has been described as being intimately attached to the underlying spinal Dura mater. However, it was not until 1995 that Hack et al.11 revealed a connective tissue bridge between the RCPm muscle and the PAO membrane–spinal dura complex of the upper cervical spinal cord (Fig. 15-2). The team of researchers from the University of Maryland at Baltimore credit the discovery to the unorthodox angle from which the dissections were performed. This was done to fully appreciate the three-dimensional arrangement of the anatomic structures of the head-neck region. Instead of performing the dissection in the standard manner from the back of the neck, the researchers made their approach from the side of the neck.12 The discovery of this muscle-dural connection has been repeatedly confirmed by other independent researchers.9,10,13,14 The connective tissue bridge has even been observed on magnetic resonance imaging (MRI) scans.15 Research using the Visible Human Female cryosectioned data set from the National Library of Medicine has also demonstrated the muscle dural bridge.16 Interestingly, Hack and his research team have obtained cadaveric data suggesting suboccipital musculature traversing the atlantoaxial junction and attaching to tissue within the vertebral canal.9 However, more studies are necessary to identify this structure and determine if it is yet another “myodural” bridge.
Hong et al.13 have described a unilateral attachment between the RCPm and the dura in 11 cadavers and a bilateral attachment in two cases. One individual revealed a unilateral connection between the inferior border of the atlas posterior arch and the spinal dura. In two cadaveric specimens, a unilateral RCPm attachment was found, and a connection between the atlas posterior arch and the Dura mater was observed on the contralateral side. The mean length and width of the RCPm muscle connective tissue bridge was found to be 13.6 mm and 1.1 mm, respectively. The authors Hypothesized that patients with short and/or asymmetrical RCPm attachments to the spinal dura may have the potential of suffering from greater amounts of cord tension and cervicogenic headaches.
An attachment has also been found to the spinal dura via the ligamentum nuchae (LN).10,17,18 The connection of the LN to the Dura mater has been found between the atlas posterior arch and the axis spinous process, and it has been identified on MRI scans.10 Mitchell et al.17 have noted the significance of this finding related to an understanding of cervical biomechanics, particularly rotational movements of the head in the sagittal or transverse planes. However, Nash et al.14 have found that the nuchal ligament does not exist in the PAO interspace and question the findings of previous studies. Attachments between the LN and RCPm muscle have also been identified.10 It has been shown that tractioning (pulling) on one of these connective tissue attachments produces movement in the other attachments.10 These findings are significant because the spinal Dura mater is innervated and a possible source of pain and neurological dysfunction if chronic tension is applied to this structure.19,20
Macroscopic dissections have shown that the CDMS ligamentous strands are actually stronger than the RCPm connective tissue fibers in the PAO membrane-spinal dura complex.9 It appears that the RCPm and CDMS connections serve an important function for stability and resisting dural infolding during movements of the upper cervical spine. This is an important function to prevent spinal dura irritation, particularly during cervical Hyperextension that can occur during “whiplash”
type injuries. It has been suggested that the RCPm muscle may “monitor” the stress on the Dura mater, whereas the infolding is guided by the collagenous and elastic fibers of the CDMS ligament.9 The RCPm is a viable candidate for providing proprioceptive feedback because of the great amount of muscle spindles contained in the muscle, which is discussed later in this chapter. Unfortunately, the previously discussed anatomical attachments can potentially leave the cord vulnerable to upper cervical Subluxations, which can result in spinal dura tension that is due to muscle spasticity or structural misalignment. This could also explain why the posterior upper cervical dura has been found to be much thicker than the anterior Dura mater.21
type injuries. It has been suggested that the RCPm muscle may “monitor” the stress on the Dura mater, whereas the infolding is guided by the collagenous and elastic fibers of the CDMS ligament.9 The RCPm is a viable candidate for providing proprioceptive feedback because of the great amount of muscle spindles contained in the muscle, which is discussed later in this chapter. Unfortunately, the previously discussed anatomical attachments can potentially leave the cord vulnerable to upper cervical Subluxations, which can result in spinal dura tension that is due to muscle spasticity or structural misalignment. This could also explain why the posterior upper cervical dura has been found to be much thicker than the anterior Dura mater.21
Dentate Ligaments
The dentate(or denticulate) ligaments are 21-paired lateral bands of epipial tissue located midway between the dorsal and ventral attachments of the nerve roots. Their medial border is continuous with the Pia mater of the spinal cord and is fixed laterally to the Dura mater. The apices of most denticulate ligaments are composed of two prongs that are approximately 1 mm in length. Part of their purpose is to attach the spinal cord to the dura and keep it in a central position. The cervical Dentate ligaments are thicker than those ligaments seen in the thoracic and lumbar regions.22 The first pair of Dentate ligaments ascend upward and into the Foramen magnum to attach to the dura of the posterior fossa. They are located between the vertebral artery anteriorly and the Hypoglossal nerve posteriorly, and the spinal accessory nerve ascends on the posterior aspect. The second pair of dentates are short, stout (thickest), and arranged almost horizontally at the level of the atlantoaxial articular facets (Fig. 15-3). This is in contrast to the ligaments below C2, which become significantly more gracile and project from the cord at gradually greater caudal angles.
The most cephalad ligaments are the thickest and strongest (particularly the second pair) of the dentates to help anchor the spinal cord around the Foramen magnum. The denticulate ligaments are more resistant to caudal than cephalad stresses in the spinal cord. Anterior and posterior motion is also constrained by these ligaments but to a limited degree, especially as one descends inferiorly along the cord.22 A strong attachment at the Foramen magnum is vital to prevent significant axial forces from being transmitted to the brainstem because of cervical flexion. The Dentate ligaments appear to serve a protective role for the central nervous system (CNS) during normal spinal motion; however, during abnormal movement of cervical vertebrae (i.e., uncoupled Subluxation), they are capable of transmitting pathological forces to the spinal cord and brainstem.
![]() FIGURE 15-3 Photo from Hinson and Zou dissection study demonstrating first and second pair of Dentate ligaments. (Courtesy of Dr. Roger Hinson) |
These ligaments are so strong that they have been found to buckle or even sever the upper cervical spinal cord in cases of severe hydrocephalus.23 The denticulate ligaments have been implicated in the kinking of the medullary spinal junction seen in patients with a Chiari II malformation.22 It should be noted that the Dentate ligaments are affected by Davis’s law, which states that soft tissue will model according to imposed demands. Cusick et al.24 have previously discussed how the “stretch Hypertrophy rule” affects living fibrous tissue structures that are exposed to a series of intermittent elongating tension loads by undergoing additional formation of collagen. The Dentate ligaments have a tendency to Hypertrophy or thicken when placed under chronic tension.22,24,25,26 This loss of elasticity can potentially increase the amount of tension that is transmitted to the spinal cord as a result of long-term upper cervical displacement.
Histodynamics of the Central Nervous System
The term Histodynamics has been used to define the study of the effects of dynamic forces on cellular elements. This field of study has shown that between full cervical extension and flexion, there is a change in the
length of the cervical neural canal by about 30 mm.26 Cervical lateral flexion elongates the convex aspect of the cord by 6 mm and shortens the concave side by the corresponding length. The spinal cord has been found to shift to the anterior in the prone position and to the posterior in the supine position. Likewise, the cervical spinal cord shifts anterior during forward flexion and posterior on extension.27 The cervical spinal cord accommodates this change by compressing somewhat in extension and stretching during flexion (prominently on the posterior aspect). However, because the Dentate ligaments are attached on each side of the cervical cord, they are capable of providing a transverse stretch of the spinal cord. Therefore, this causes the anterior to posterior (AP) dimension of the cord to be reduced, but the transverse diameter will slightly increase (Fig. 15-4).28,29,30 This is known as Poisson’s effect: a decrease in cross-sectional area with an increase in length, or vice versa, while the total volume remains constant.29
length of the cervical neural canal by about 30 mm.26 Cervical lateral flexion elongates the convex aspect of the cord by 6 mm and shortens the concave side by the corresponding length. The spinal cord has been found to shift to the anterior in the prone position and to the posterior in the supine position. Likewise, the cervical spinal cord shifts anterior during forward flexion and posterior on extension.27 The cervical spinal cord accommodates this change by compressing somewhat in extension and stretching during flexion (prominently on the posterior aspect). However, because the Dentate ligaments are attached on each side of the cervical cord, they are capable of providing a transverse stretch of the spinal cord. Therefore, this causes the anterior to posterior (AP) dimension of the cord to be reduced, but the transverse diameter will slightly increase (Fig. 15-4).28,29,30 This is known as Poisson’s effect: a decrease in cross-sectional area with an increase in length, or vice versa, while the total volume remains constant.29
![]() FIGURE 15-4 Flattening of the cervical spinal cord in the anterior to posterior dimension during neck flexion because of restraining effects of Dentate ligaments. (Modified from Eriksen K. Upper Cervical Subluxation Complex. Baltimore: Lippincott Williams & Wilkins, 2004:75.) |
Breig28 has provided photographs and discussion related to tension on the Dentate ligaments when the cervical spine is flexed. This can also occur with Cervical Kyphosis and osteophytic protrusions. This tension is then transmitted to the spinal dura, which can stretch the lateral branches of the central spinal cord arteries that supply the pyramidal tracts. Stretching the central spinal arteries can result in muscle spasticity that is typical of myelopathies. These conditions can result in other neurological signs and symptoms as well. Breig28 noted one patient who could provoke or increase the spasticity in both legs by forcefully flexing his neck. The patient apparently did this to enable him to stand on his weak legs, as this stimulus resulted in immediate spastic rigidity.
Bedford et al.25 conducted a postmortem examination of a patient who had suffered from severe Cervical spondylosis. The patient had reported pain and weakness of the legs, as well as numbness of the right hand and forearm, among other symptoms. Histological examination revealed degeneration of both the gray and white matter, with diffuse loss of nerve cells and severe gliosis. Demyelination was noted near the attachments of the Dentate ligaments, which were thicker and more cellular than usual. The condition of these ligaments was likely due to the chronic tension applied to these structures from the severe spondylosis and protruded disc material, which caused spinal cord displacement. Shimizu et al.31 surgically created kyphotic deformities in the cervical spines of birds and then performed histological evaluations to determine the effects of chronic spinal cord compression. This condition resulted in Demyelination of nerve fibers in the funiculi and neuronal loss in the anterior horn because of spinal cord compression. The histologic changes seem to be associated with both continuous mechanical compression and vascular changes in the spinal cord.
A report from the British Medical Journal revealed a court case in which the plaintiff was awarded $820,875 for posttraumatic multiple sclerosis (MS) that was apparently triggered by an automobile accident.32 However, the case was appealed, and the settlement was reduced. Chaudhuri and Behan33 have reported on 39 cases in which MS was precipitated or exacerbated by Hyperextension-Hyperflexion cervical trauma to the spinal cord. The worsening or onset of the MS bore a striking temporal relationship to the injury. Their data suggest that CNS-specific acute cervical spine trauma may aggravate latent clinical symptoms in MS. These findings are intriguing because upper cervical chiropractic care has been found to be related to clinical improvement in patients with MS.34,35,36,37
Dentate Ligament–Cord Distortion Hypothesis
Because of the complexity of the upper cervical spine from an anatomical and biomechanical (see Chapter 16)
standpoint, these Articulations appear to be quite susceptible to Subluxation. Dr. John D. Grostic’s Dentate ligament–cord distortion Hypothesis3,38 provides a compelling theory for how these previously described anatomical connections can lead to spinal cord distortion in the presence of upper cervical misalignment (Fig. 15-5). However, there are three main challenges to this Hypothesis.
standpoint, these Articulations appear to be quite susceptible to Subluxation. Dr. John D. Grostic’s Dentate ligament–cord distortion Hypothesis3,38 provides a compelling theory for how these previously described anatomical connections can lead to spinal cord distortion in the presence of upper cervical misalignment (Fig. 15-5). However, there are three main challenges to this Hypothesis.
Are the Dentate ligaments mechanically linked to the upper cervical spine by way of dural attachments to various structures?
Are the Dentate ligaments strong enough to deform the spinal cord?
Are the osseous misalignments large enough to cause mechanical irritation to the spinal cord?
![]() FIGURE 15-5 A: Cross-section of spinal cord at the atlantoaxial level. B: Proposed spinal cord distortion resulting from severe lateral and rotational occipitoatlantoaxial Subluxation. |
Clinical Significance of Osseous Misalignments
The first two points have been previously covered in this chapter. The clinical significance of the size of the upper cervical displacement still must be addressed. This can be determined if one knows the average lateral misalignment between the atlas and skull, as well as the average radius of curvature of the occipital condyles. A retrospective study involving 523 patients found that the average occipitoatlantal misalignment in the frontal plane is almost 3°, whereas the average condylar curvature radius is about 57 mm.39 This equates to about 1/8 inch of atlas linear movement in relationship to the occiput when utilizing the following equation:
Displacement = 2 • Radius • π • Angular Displacement/360
This amount of atlanto-occipital misalignment is potentially significant because the upper cervical spinal cord has a diameter of about ½ inch. This means that the upper cervical spinal cord could be deformed in a lateral direction, different from that caused by normal range of motion, by close to 25% of its diameter if the effects of this lateral displacement are transmitted unattenuated. However, it is likely that the limited elasticity of the Dentate ligaments and other dural-connective tissue attachments along with normal biomechanical coupling (see Chapter 16) will dissipate the stress to some degree. It must again be noted that denticulate ligaments have a tendency to thicken when placed under chronic tension; thus, their ability to dampen the tensile forces to the cord is decreased in these cases. Indeed, lateral misalignments between the atlas and axis (Lower angle) can potentially transmit more tension to the spinal cord because the superior articular surface of axis is usually flatter (larger radius of curvature) than the occipital condyles.
Cusick et al.24 studied the role of the Dentate ligaments in the pathogenesis of Myelopathy secondary to alterations of the spinal canal. The spinal cords of 14 dogs were elevated posteriorly in 0.8-mm increments and evaluated with somatosensory evoked potentials. Neurological function in the spinal cord was assessed before and after dentate ligament section. It was found at the initial increment of elevation that a deficit in the evoked potential amplitude was first detected. This distance approximately equates to the linear movement of an atlas that has subluxated 0.75° around the occipital condyles. It has been observed clinically for more than 60 years that this degree of atlas misalignment is the minimum amount required to cause neurological insult, although not all patients will reveal signs of Subluxation at this small degree of occipitoatlantal lateral displacement.
It would seem that this small degree of misalignment would be within the adaptability of CNS. However, Grostic has pointed out that a study by Jirout40 found the maximum AP movement of the upper cervical spinal cord in the Sagittal plane to be about 4 mm. Grostic used this maximum distance and the approximate measurements
of the atlas as published by Lang41 to calculate the lateral deviation of the spinal cord produced by this AP motion’s shortening effects on the Dentate ligaments. He determined that the upper cervical cord’s lateral deviation to be approximately 0.5 mm. Grostic noted:
of the atlas as published by Lang41 to calculate the lateral deviation of the spinal cord produced by this AP motion’s shortening effects on the Dentate ligaments. He determined that the upper cervical cord’s lateral deviation to be approximately 0.5 mm. Grostic noted:
It seems that even at the limits of normal motion the distortion of the cord does not equal that of even the smallest clinically significant misalignment. Traction on the cord by misalignments thus would seem to be significantly larger than those produced by normal movement.3
![]() FIGURE 15-6 Laminar arrangement of spinal cord tracts. Right side of spinal cord demonstrates potential stress on nerve tracts via Dentate ligaments secondary to upper cervical Subluxation. (Modified from Eriksen K. Upper Cervical Subluxation Complex. Baltimore: Lippincott Williams & Wilkins, 2004:83.) |
A study from the department of neurology of New York University Medical Center examined whether either of two mechanical theories predicted the topographic pattern of neuropathology in Cervical spondylotic Myelopathy (CSM).42 The compression theory states that the spinal cord is compressed between a spondylotic bar anteriorly and the ligamenta flava posteriorly. The dentate tension theory states that the spinal cord is pulled laterally by the Dentate ligaments, which are tensed anteriorly by a spondylotic bar. The predicted stress pattern of the dentate tension theory corresponded to the reported neuropathology, whereas the predicted stress pattern of the compression theory did not. It was found that the area of greatest stress was found in the lateral columns. This is the region where the spinocerebellar and spinothalamic tracts are located. The spondylotic bar may also increase dentate tension by interfering locally with dural stretch during neck flexion, the resultant increase in dural stress being transmitted to the spinal cord via the Dentate ligaments.
The design of the upper cervical spine has sacrificed stability for mobility as evidenced by about 50% of cervical rotation occurring between the atlantoaxial Articulation and the lack of facet restriction. It is posited that the neurological dysfunction can occur via two mechanisms: (i) direct mechanical irritation of the nerves of the spinal cord, and/or (ii) collapse of the small veins of the cord, producing venous congestion and resultant Hypoxia. This neurological dysfunction could be the cause of many clinical signs and symptoms observed in patients with upper cervical Subluxations.
Kobrine et al.43 have conducted experiments that measured neurological dysfunction after ischemia of the cervical spinal cord. They state that the spinal evoked response returned rapidly after blood flow was reestablished. This is an important statement, because some have argued that if a mechanical impingement of the nervous system exists, it would take days to return to normal. Lower levels of Hypoxia do not cut off the function of nerves, although this can initially make them more excitable and increase susceptibility to neurological dysfunction. Some spine surgeries use evoked potential and surface electromyelographic technology to assess nerve function during the interventions. An upper cervical Subluxation does not have to press on a nerve or even cause severe stretching to cause neurological insult.
Laminar Arrangement of Spinocerebellar and Spinothalamic Tracts
The spinocerebellar tracts (SCTs) are located along the lateral edge of the spinal cord (Fig. 15-6) and are at the most probable site of mechanical irritation via the Dentate ligaments.44 The origin of the second pair of dentates is just beyond the dorsal SCT and very close to the spinal tract of the trigeminal nerve and the superficial lamina of the dorsal horn. These proprioceptive tracts are primary pathways for regulating muscle tone and
joint position sense. Kahn45 has discussed how compression, tension, and anoxia affect the conductivity of large-fiber nerves (i.e., SCTs) before their smaller counterparts (i.e., nociceptive fibers). Large-fiber nerves include those conveying light touch, motor, vibratory, and position sense as opposed to pain-transmitting fibers. The SCTs are arranged in a laminar fashion, with the most lateral fibers innervating the most caudal structures (i.e., legs-sacral-lumbar-thoracic). Irritation of the lateral aspect of these tracts by the Dentate ligaments could lead to muscle tone imbalance of the pelvic girdle, resulting in a functional short leg (see Chapter 9).
joint position sense. Kahn45 has discussed how compression, tension, and anoxia affect the conductivity of large-fiber nerves (i.e., SCTs) before their smaller counterparts (i.e., nociceptive fibers). Large-fiber nerves include those conveying light touch, motor, vibratory, and position sense as opposed to pain-transmitting fibers. The SCTs are arranged in a laminar fashion, with the most lateral fibers innervating the most caudal structures (i.e., legs-sacral-lumbar-thoracic). Irritation of the lateral aspect of these tracts by the Dentate ligaments could lead to muscle tone imbalance of the pelvic girdle, resulting in a functional short leg (see Chapter 9).
The spinothalamic tracts are also located close to the attachment of the Dentate ligaments and are organized in a laminar arrangement as well (Fig. 15-6).46 These tracts are responsible for conveying pain and temperature into the Neuraxis. Mechanical irritation and/or ischemic compromise to the spinothalamic tracts could possibly explain cases of severe low back and leg pain responding dramatically to upper cervical Adjustments. This is a possible mechanism for the rapid (at times instantaneous) relief of sciatic-type pain, especially in patients without demonstrable clinical findings in the lumbosacral region. The author has observed this response in his clinical practice on numerous occasions, as well as in the offices of other upper cervical doctors. Grostic3 describes the experience of many patients under exclusive upper cervical care as feeling a sense of warmth or even mild electric shock sensations in the lower extremity simultaneously with the Adjustment. These sensations are typically indicative of spinal cord involvement and are a bit cumbersome to explain with the mechanoreceptive Dysafferentation models.
Kahn45 and Breig28 have reported how cervical spinal cord tension via the Dentate ligaments can cause lower spine and extremity symptoms. Cord compression that is due to lesions in the cervical and upper thoracic spine has been shown to cause lower extremity pain and other neurological symptoms as well.47,48,49 Surgery has been administered in the past to excise tethered Dentate ligaments to relax the cord and relieve symptoms. Breig28 noted the value of Kahn’s Dentatotomy and has reviewed 21 cases in which the surgical procedure led to reduction of spasticity. It has been speculated that the clinical symptoms likely result from involvement of the spinothalamic tract and possibly the posterior columns. The pain tends to be diffuse and usually radiates bilaterally with a burning or aching quality. Some authors have also described the patient’s pain as being deep and boring in contrast to the more superficial radiating pain of sciatica. Patients with cervical root sleeve fibrosis have noted radiating pains in the head, arms, and sciatic regions upon cervical flexion.28
Breig28 also explored the possibility of bladder dysfunction being caused by pathological tension of the spinal cord. Cervical spinal cord injuries are known to be related to serious gastrointestinal complications.50 This is possibly due to sympathetic visceral input being altered or lost because the preganglionic fiber cell bodies are located in the thoracolumbar intermediomedial and intermediolateral cell column (T4-L2). However, because parasympathetic innervation of the gut is provided by the vagus and pelvic nerves, it typically remains intact following spinal cord injury.51 It is posited that this sympathetic/parasympathetic imbalance can lead to disruption of autonomic innervation to the gastrointestinal tract, which can cause various complications.
The historical position of the National Upper Cervical Chiropractic Association has been that upper cervical misalignment causes traction of the brainstem.52 It is proposed that this traction results in inhibition of medullary reticulospinal tract (inhibitory center), leading to (or causing) an imbalance with the pontine reticulospinal tract (facilitory center). This can result in an overfacilitation of postural motor tone because these tracts Work together to modulate the extensor or “antiGravity” muscles. It is thought that this helps to explain the resolution of the clinical signs and symptoms related to postural distortion that are observed after upper cervical Adjustments.
One may argue that if the misalignments measured on nasium and Vertex X-rays are the cause of spinal cord tension, then one could do the same or worse by putting the neck through normal Range of motion (ROM). However, the occipitoatlantoaxial Subluxation is a static misalignment, as opposed to transient minor movement of the Articulations through normal movement. Most of the limited amount of atlas slippage in relation to the occiput (in lateral flexion and rotation) occurs at the extremes of the spinal ROM. Because people do not hold their necks in these positions for long durations, spinal cord tension is typically not increased for a significant length of time. However, if they did, they would likely cause some degree of nerve dysfunction. Unfortunately, the upper cervical Subluxation remains with the patient 24 hours a day, 7 days a week, until a correction can be made. It is proposed that a Subluxation may be present for months or even years before significant symptoms develop. The nervous system appears to be able to adapt to short-term cord tension, but it is proposed that over time an uncoupled upper cervical misalignment will eventually produce symptoms.
Coupled motion should also be considered in the pathomechanics of the Subluxation. It is proposed that during normal spinal ROM, coupled motion helps reduce tension on the nervous system. This is accomplished by offsetting pure lateral flexion or rotation, with small amounts of movements in the X-, Y-, or Z-axes. However, upper cervical misalignment patterns
tend to displace in an abnormally coupled manner when signs of upper cervical Subluxation exist, which theoretically creates further stress on the Articulations and transmits more tension to the CNS (see Chapter 16).
tend to displace in an abnormally coupled manner when signs of upper cervical Subluxation exist, which theoretically creates further stress on the Articulations and transmits more tension to the CNS (see Chapter 16).
![]() FIGURE 15-7 Various types of somatic sensory nerve endings. (From Eriksen K. Upper Cervical Subluxation Complex. Baltimore: Lippincott Williams & Wilkins, 2004:62.) |
Mechanoreceptive Dysafferentation
Somatosensory Receptors
Mechanoreceptive Dysafferentation has important implications in the upper cervical region and its relationship to neurological integrity. The nervous system is separated into three functional divisions: sensory, motor and integration, and sensory receptors (i.e., Mechanoreceptors and Nociceptors) play a vital role in all of these categories. Mechanoreceptors derive their name because they are activated by mechanical deformation. These receptors are a morphologically diverse group that vary in size and function (Fig. 15-7). Mechanoreceptors are found in the skin, muscles, joints, and visceral organs; they are predominantly concerned with conveying tactile sensations and position sense to the Neuraxis.
Position sense can be divided into two categories: static position and Kinesthesia. Static position represents awareness of the orientation of different parts of the body with respect to each other. Kinesthesia is the sense that detects bodily position and movement of muscles, tendons, and joints. Mechanoreceptors are primarily responsible for the body’s position sense, which is particularly influenced by the existence in a Gravity environment. This requires the brain’s motor control centers to have detailed information regarding the momentary position and movement of each body part. This is particularly important in humans because of bipedal stance and an inherent instability of a high center of Gravity coupled with a narrow base of support. Proprioceptive organs include the ocular receptors (eye), labyrinth (inner ear), cutaneous receptors (skin), joint Mechanoreceptors, muscle spindles (MSp), and the Golgi tendon organs (GTOs).
The optic receptors provide visual information to the Neuraxis regarding the body’s relationship to the horizon. The vestibular apparatus (VA) informs the brain of the head’s position and Works to keep it Perpendicular with the ground by altering the tone of the cervical muscles. Small postural deviations of the skull are primarily detected by the membranous labyrinth, whereas the eyes play a more significant role in determining the head’s position in space with more extreme head tilts. Mechanoreceptors provide appropriate information depicting the orientation of the head with respect to the body to maintain equilibrium.
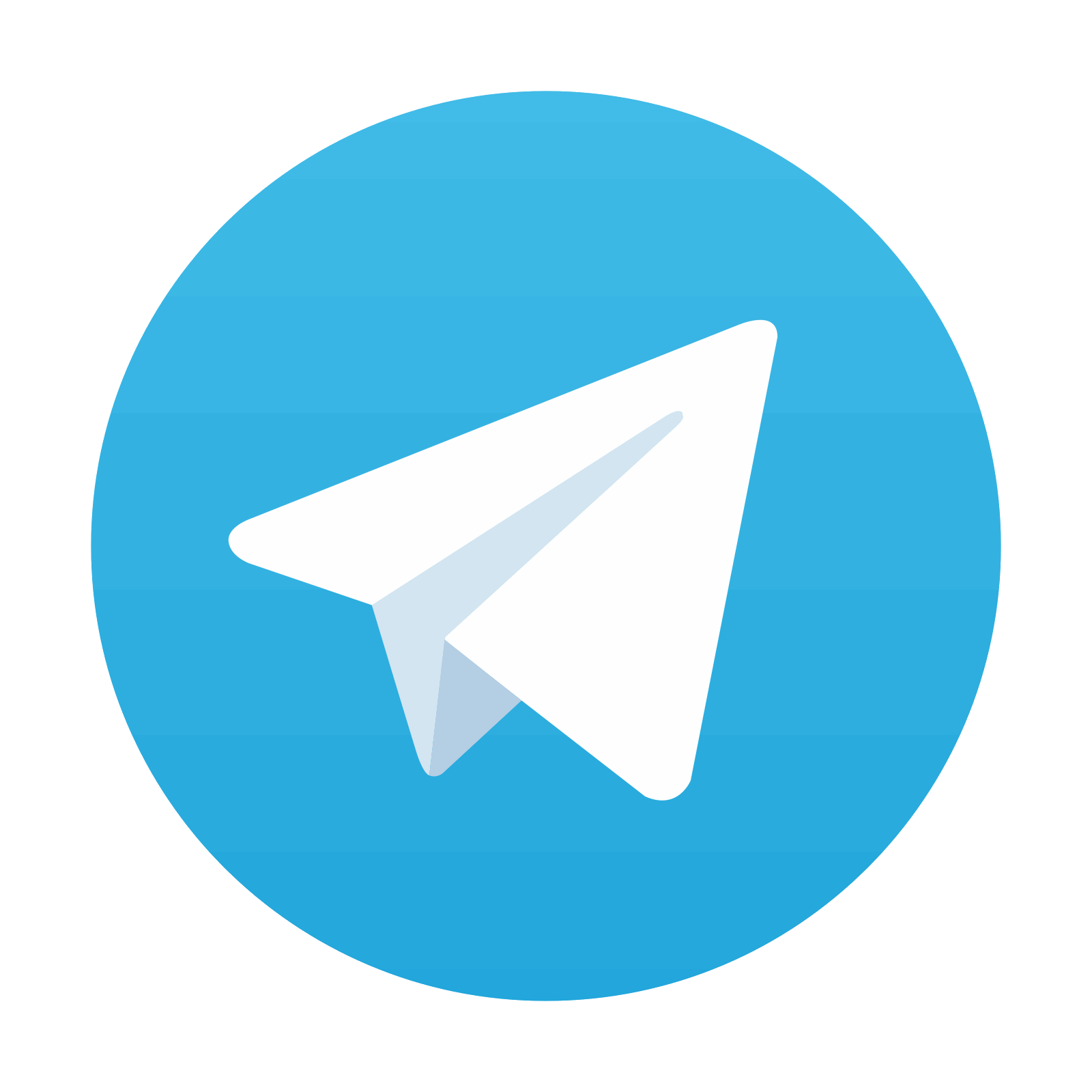
Stay updated, free articles. Join our Telegram channel

Full access? Get Clinical Tree
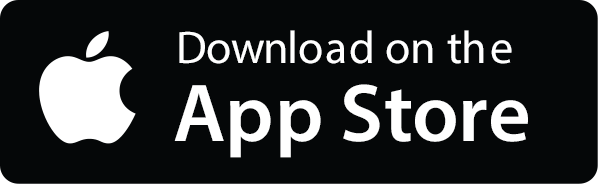
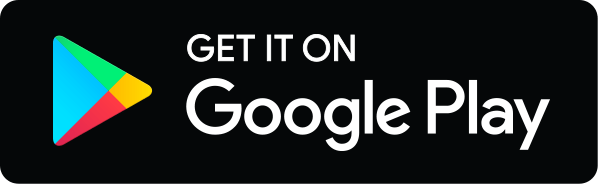