Multiple Sclerosis
Francois Bethoux
Alexander Rae-Grant
Multiple sclerosis (MS) is a complex, multifactorial disease that requires the expertise of neurologists, physiatrists, occupational therapists, and physical therapists, as well as countless other participants in the patient’s care. Rehabilitation physicians have an integral role during the disease course including the assessment and management of paresis, spasticity, gait, activities of daily living, bowel and bladder function, wellness issues, and pain. Since MS is a long-term and often progressive disorder, the focus is not only on improving function where necessary but also on maintaining function where possible. A firm understanding of the underlying pathophysiology of MS as well as the role and rationale of treatment is the key to the rehabilitative treatment of MS.
MS is the most common cause of nontraumatic disability affecting young adults in the Northern Hemisphere (1). Onset is usually in the 20s to 40s, a time when they are in the core of their working and childbearing years (2). The disease varies from patient to patient and the care needs change from year to year. Thus, it is crucial to have a team approach to maintain and improve function. There are presently six food and drug administration (FDA) approved medications that alter the disease course of MS, and there are more in the pipeline; however, none provides a cure or an alternative to rehabilitative approaches to MS care.
DEMOGRAPHICS AND EPIDEMIOLOGY
MS is a common disorder in North America. Prevalence varies in the United States and Canada and ranges from approximately 40 to 220 per 100,000 population (3). With some exceptions, the prevalence of MS increases further north and south of the equator one lives (4). Various theories have been proposed to explain this distribution, as well as the notable exceptions to this geographic observation in some populations (Inuit, Laplanders, etc.) (5). Theories include vitamin D deficiency due to reduced sunlight, dairy products, genetic populations, and exposures to a variety of environmental factors (6). MS is preponderantly a disease of persons of Northern European ancestry (7). Infections have been linked to MS, but no one infection has emerged as a specific cause or precipitant of disease activity (8). In pediatric MS, late infection with mononucleosis has been associated with MS (9). Exacerbations of MS are more common after various infections, but the type of infection appears to be immaterial (10).
ETIOLOGY
MS is considered to be an “autoimmune” diseases (11). Like all autoimmune disorders, it is more common in women (12), occurring about twice as often in women as in men (13). MS also appears to involve genetic factors with HLA-DR2 in DR-positive families—having a greater chance of developing the disease (14). The risk of concordant MS is 30% with monozygotic twins, 5% with dizygotic twins, and between 2% and 4% for first-degree family members of people with MS (15). Multiple genetic linkage studies have confirmed a linkage with the major histocompatibility region, as well as less well defined linkages to other zones that code for interleukins (16). MS is not increased in children adopted into families with MS (17), which indicates that family aggregation of MS is due to genetic factors rather than environmental (18). The specific cause of MS remains unknown.
PATHOLOGY, PATHOGENESIS, AND PATHOPHYSIOLOGY
The hallmark of MS pathology is the presence of multifocal plaques (lesions) of demyelination in the cerebral hemispheres, optic nerves, brain stem, and spinal cord (19). The early plaque has a demarcated area of demyelination with incomplete axonal injury, inflammatory infiltrates composed of lymphocytes and macrophages, and evidence of astrocytic proliferation and gliosis (20). Ultrastructural analysis of axons has shown an early reduction in axonal fibers and axonal transections in new demyelinating regions (21).
In acute active lesions, gadolinium leaks into tissue parenchyma due to blood-brain barrier (BBB) interruption that accompanies the inflammatory response (22). Perivenular lymphocytic infiltrates are evident in areas of demyelination (23). Macrophages are the most prominent inflammatory cells in the lesion and many are filled with myelin debris (24). Proinflammatory CD4+ T cells appear to be critical components in the demyelinating process (25). B cells from peripheral blood are recruited to the active plaque and differentiate into plasma cells that synthesize and release immunoglobulin (26). Plasma cells are more commonly encountered in chronic lesions (27).
With progression of the pathological process, chronic inactive lesions are less inflammatory and become hypocellular,
with relatively quiescent oligodendrocyte precursor cells (28). Degeneration occurs, as oligodendrocytes are destroyed and astrocytes proliferate. As demyelination occurs, there can also be some degree of remyelination in the lesion (29). However, as the disease progresses, demyelination at the plaque margin takes place in the newly remyelinated areas and leads to expansion of the lesion. This eventually results in permanent scarring.
with relatively quiescent oligodendrocyte precursor cells (28). Degeneration occurs, as oligodendrocytes are destroyed and astrocytes proliferate. As demyelination occurs, there can also be some degree of remyelination in the lesion (29). However, as the disease progresses, demyelination at the plaque margin takes place in the newly remyelinated areas and leads to expansion of the lesion. This eventually results in permanent scarring.
PATHOPHYSIOLOGY OF MS
The clinical symptoms of MS are often due to loss of axonal conduction. Demyelination of segments of conducting axons causes conduction block which varies depending on the extent of demyelination and whether compensatory mechanisms have intervened. Conduction block in experimental demyelination occurs at sites of demyelination and does not occur in otherwise unaffected segments (30). The block appears to be most severe in the first few days after experimental demyelination (31). Acutely demyelinated axolemma has a relatively low sodium channel density which may be insufficient for the action potential to be propagated effectively (32).
Another factor influencing conduction is inflammation (33). Cytokines have been shown to play a role in conduction block, particularly proinflammatory cytokines such as tumor necrosis factor-α and interferon (IFN)-γ (34). Both of these cytokines induce nitric oxide formation (35). Nitric oxide production is increased in MS (36) and has been shown to cause a dose-dependent conduction block (37).
Safety factor is a measure of the excess current allowing conduction divided by the minimum current necessary to depolarize an axon (38). In normal myelinated axons, this is usually a factor of three to five times. In demyelinated axons this is reduced, often measured at just above 1. Small changes in environmental factors can thus cause axonal block in such fibers (reduced safety factor). This may be the basis for worsening MS symptoms with fever and with exercise, both of which may reduce safety factor to the point where conduction block occurs.
Restoration of conduction occurs in demyelinated axons after a few days or weeks. This is likely related to the appearance of sodium channels along the demyelinated portion of the axon to allow microsaltatory conduction along these demyelinated segments (39). Remyelination occurs with improved conduction in previously demyelinated segments (40). In addition, the resolution of inflammation may also improve conduction block possibly due to normalization of nitric oxide levels (37).
Patients frequently note that with repeated activity they develop weakness, especially with walking. In experimental demyelination, a train of stimuli over time will elicit intermittent conduction block (30). This correlates with hyperpolarization of the membrane (41).
Restoration, Remyelination, and Neuroprotection
Recovery of function (remission) may be due to resolution of inflammation or the pressure of edema, removal of humoral factors, reattachment of paranodal myelin, or rerouting of nerve transmission through alternative pathways (brain plasticity) (42). Remyelination may also be a key component in the restoration phase, which usually requires days to weeks (43). Early studies of spinal cord MS lesions suggested that regeneration of central myelin is accompanied by Schwann cell invasion of the central nervous system (CNS) and the presence of peripheral myelin within the demyelinated region (29).
A classic neuropathological finding with remyelination is “shadow plaques.” These represent partial reduction of myelin staining as remyelinated axons have thinner myelin sheaths. Such remyelination may be extensive in some patients (44). Remyelination may be seen on magnetic resonance imaging (MRI) as intermediate lesions on T1 images and appear less dark compared to hypodense “black holes” (which represent significant axon damage).
TYPES OF MS
An international panel was convened in the late 1990s to develop a common classification of subtypes of MS (45) (Fig. 25-1). This classification is useful as a way to group patients, though separations between subtypes may be more artificial than real.
Relapsing-Remitting MS
Relapsing-remitting patients have discrete attacks of new or worsened neurological symptoms that emerge over a few days and may resolve over a 4- to 8-week period with or without corticosteroid treatment. These patients often return to their preattack baseline but may have residual disability. Relapsing-remitting multiple sclerosis (RRMS) is the most common form of MS (46). RRMS frequently begins with optic neuritis (ON)—transient unilateral visual impairment lasting days to weeks, which may be associated with retrobulbar pain (47). Others with RRMS may initially experience tingling or weakness of a limb; paresthesias are the most common initial symptom of MS (48). In general, patients with sensory symptoms, and patients whose symptoms fully remit after early exacerbations, demonstrate better long-term prognosis (48). FDA and internationally approved medications are available for patients with RRMS (49, 50, 51, 52, 53). These medications in general reduce new exacerbation frequency, reduce measures of MRI activity, and may slow progression of disability. Note that patients who have exacerbations and are left with residual disabilities and those with complete resolution are both still considered RRMS (Fig. 25-1). Nevertheless, from a functional perspective these two patient groups are dramatically different.
Secondary Progressive MS
These are patients who initially have RRMS but after some years of disease have gradual worsening in between discrete attacks. They may have continued attacks or stop having attacks. They also tend to have fewer attacks and less MRI gadolinium enhancement than during the relapsing-remitting phase. A significant proportion of RRMS patients convert to secondary progressive multiple sclerosis (SPMS) at some time in their disease course. IFN-β1b and mitoxantrone slow the progression of SPMS if given in the early part of this stage, particularly when there are continued relapses (53, 54). However, in older patients who do not have continued relapse activity, the beneficial effect of these medications is less pronounced (55). Patient with accrued deficits such as gait disorder and spasticity may benefit from rehabilitative approaches.
Primary Progressive MS
Primary progressive multiple sclerosis (PPMS) patients are often older at onset and have a progressive course without attacks. They tend to have fewer MRI lesions than RRMS or SPMS patients. PPMS occurs in about 10% of patients with MS. PPMS tends to affect both sexes equally, unlike RRMS (56). It usually becomes clinically apparent when patients are in their 50s (57). PPMS more often starts with motor symptoms, often an asymmetric paraparesis, and tends to progress more rapidly, validating observations that patients starting with motor symptoms fare less well (vs. sensory symptoms), regardless of disease type (57).
There are no FDA-approved immunomodulating agents for PPMS. Although some have been tried, none has shown sufficient benefit for regulatory approval. Some randomized, blinded trial efficacy has been reported for methotrexate, but the effect was limited to upper limb function as measured by timed Nine-Hole Peg Testing (58). Pulse methylprednisolone has also been reported in some studies to be useful in this cohort of patients (59).
Progressive-Relapsing MS
Progressive-relapsing MS is a form of MS where the patient experiences progressive disease at the beginning but begins to have attacks later in their course. Probably no more than 5% of MS patients have this form. Effective medical treatment is unclear for this subgroup.
Fulminant MS
These are patients with rapid severe progression with relapses over a period of months. This type of MS affects a small percentage of patients.
OTHER ASSOCIATED DEMYELINATING DISORDERS
Optic Neuritis
Many patients with MS will present with ON, a sudden unilateral loss of vision, which can vary from a slight central scotoma to complete loss of light perception (47). In one long-term study of ON, 57% eventually developed MS (60). Both ON and ON/MS patients respond favorably to intravenous (IV) methylprednisolone (47). A recent Cochrane analysis of corticosteroid use for ON concluded that there is no evidence of a long-term benefit of high- or low-dose steroids; the improvement seen with high-dose corticosteroids in the ON trials appears to be limited to short-term outcomes (61). Data on patients with clinical isolated syndromes (CIS) such as isolated ON have shown that patients with multiple brain lesions on MRI are at higher risk of progressing to clinical MS within 1 to 2 years after presentation (62). Obtaining a brain MRI in patients with ON or other CIS is therefore an effective risk stratification methodology and potentially guides later therapy.
Transverse Myelitis
Transverse myelitis (TM) is an inflammatory disorder of the spinal cord (63). It may present after an infection in about half of cases. Most patients have rapid progression to a relatively complete spinal cord syndrome with paralysis, sensory level, and bowel and bladder involvement. Cerebrospinal fluid (CSF) may show a pleocytosis but usually does not show oligoclonal banding (64). Recovery is variable and often incomplete. There are no randomized trials of therapy for classic TM; therapies tried include IV solumedrol, IV immunoglobulins (IVIG), and plasmapheresis (65). A subset of patients, usually with incomplete TM, will develop MS (66). In addition, a subset of TM patients have a positive neuromyelitis optica-IgG (NMO-IgG), which is a subform of Devic’s disease (see below). TM patients who eventually develop MS typically present with asymmetric
and incomplete motor or sensory symptoms, whereas those with pure TM not converting to MS generally have symmetric findings and more profound disability (67). Key rehabilitation issues in TM include mobility, spasticity, bowel and bladder management, and avoiding decubitus ulceration and deep venous thrombosis during the acute illness.
and incomplete motor or sensory symptoms, whereas those with pure TM not converting to MS generally have symmetric findings and more profound disability (67). Key rehabilitation issues in TM include mobility, spasticity, bowel and bladder management, and avoiding decubitus ulceration and deep venous thrombosis during the acute illness.
Devic’s Disease (Neuromyelitis Optica)
The traditional concept of Devic’s disease consists of the subacute combination of relatively severe ON and TM with sparing of the brain (68). With new research, the classification of neuromyelitis optica has undergone significant revision and likely will continue to evolve (69). In general, patients have a combination of ON and TM, both of which can be severe, with initial relative sparing of brain involvement. The spinal lesions tend to be longer (three or more cord segments) than typical MS lesions in the cord (usually one segment only) (69, 70). CSF may show a striking pleocytosis or a neutrophilic predominance which would be unusual for MS (71). A recently developed antibody, the NMO-IgG antibody, is positive in 70% of clinically defined neuromyelitis optica and is rarely positive in MS.
DIAGNOSIS OF MS
The most common presenting course for MS is relapsing symptoms affecting different neurological structures occurring over time (“lesions distributed in time and space”). Common symptoms include fatigue, ataxia, weakness, numbness and tingling, bladder dysfunction, spasticity, cognitive problems, depression, ON, and pain. Notwithstanding multiple revisions over time, the diagnosis of MS still requires the presence of signs and symptoms separated in space and time, or the presence of progressive symptoms with appropriate paraclinical evidence of demyelination (72, 73, 74, 75).
Diagnostic Criteria
Over the years, several sets of diagnostic criteria have been developed. In the 1960s, Schumacher et al. based the diagnosis of MS entirely on clinical findings (72). In the early 1980s, these were modified by Poser et al. to allow laboratory tests to substitute for some clinical criteria (73). Much more recently, as laboratory testing—especially MRI—has improved, laboratory results have been allowed to substitute for even more clinical findings (74, 75). Specific MRI criteria to support the diagnosis of MS have been elucidated (76).
In general, the diagnosis of MS continues to be a clinical one with supporting evidence from MRI, CSF, and evoked potentials as needed (75). MRI assists in the diagnosis when it shows characteristic white matter lesions. Lesions in the periventricular, juxtacortical, subcortical, infratentorial, and spinal cord regions are all helpful. The MRI can be used to demonstrate dissemination in space, replacing examination findings in two areas. Repeated MRIs over time showing new lesion formation can also be substituted for further attacks in making a diagnosis. CSF and MRI findings are helpful in PPMS, particularly in ruling out other causes of a progressive myelopathy such as spinal cord tumor (75). The McDonald criteria allow for consistent diagnostic criteria to be applied and are helpful in epidemiological studies as well as patient recruitment into clinical trials (75).
Magnetic Resonance Imaging
MRI has become the most important paraclinical tool in the diagnosis and monitoring of MS and related diseases (77). A variety of MRI measures have been used to analyze MS in terms of diagnosis, progression, the acute lesion, subtypes of MS, and monitoring treatment trials (78).
MRI has also been crucial in modifying our concepts of disease pathogenesis and course in MS. In the 1980s, sequential MRI trials showed that new lesion formation occurs five to ten times as often as there were new clinical events, altering the understanding of disease activity during clinically quiescent times (79, 80, 81). In addition, MRI has supported the notion of early axonal injury with MS lesions which pathological studies have introduced. Longitudinal MRI measures of atrophy have shown slow but definite atrophy with time in patients with otherwise relapsing MS (82, 83). Finally, MRI imaging particularly with FLAIR (Fluid Attenuated Inversion Recovery) has shown that cortical lesions are common in MS, a fact that went unrecognized prior to these imaging techniques (84, 85).
MRI has also shown us that there is a limited correlation between the extent of measurable brain disease and measurable disability. Some patients with limited MRI change have significant deficits, and some with extensive white matter and gray matter changes have limited clinical deficits. The explanation for this is unclear but may involve disease in areas not measurably affecting function; compensatory changes that mask functional deficits; spinal cord involvement not imaged on brain MRI causing deficits; and changes that do not cause noticeable neural transmission deficits.
Conventional MRI imaging of MS lesions identifies MS lesions as often paraventricular, oval-shaped lesions, sometimes oriented tangentially to the ventricular surface. Lesions of the corpus callosum are common and extend in a fingerlike pattern, known as “Dawson’s fingers” (78). With disease progression, lesions may coalesce. With more chronic and severe disease, brain atrophy becomes apparent. T1 lesions (black holes) are correlated with axonal loss in the affected areas (86).
For several weeks after the occurrence of the breakdown in the BBB, affected brain will be permeable to gadolinium. Consequently, MRIs taken shortly after the IV injection of gadolinium will show “enhancement” or opaque regions on T1-weighted images. A characteristic enhancement pattern for MS is the “open ring sign,” where gadolinium enhances in an incomplete peripheral pattern, often pointing away from the lateral ventricles (87).
Other techniques that at present have a primarily research application include brain parenchymal fraction (83), magnetization transfer ratio (88), diffusion tensor imaging (89), and MR spectroscopy (90). These techniques may be most helpful in documenting axonal tract fibers loss and in predicting disability.
Evoked Potentials
The original work in evoked potentials came from Dawson’s lab in the 1940s (91). Dawson and his colleagues showed that one could record neurological activity from the CNS with peripheral stimulation of a sensory pathway using summation of multiple stimulations by averaging. They and others demonstrated slowing of central conduction in MS patients. This was consistent with the concept of a demyelinating disorder as opposed to one with primarily axonal injury. Slowing in the visual evoked potential is the most classic example, but similar slowing can be seen with somatosensory evoked potentials and brainstem auditory evoked potentials (92). American Academy of Neurology guidelines suggested that visual evoked potentials are probably useful to identify patients at increased risk for developing clinically definite MS; somatosensory evoked potentials are possibly useful to identify patients at increased risk for developing clinically definite MS; and there is insufficient evidence to recommend brainstem evoked potentials as a useful test to identify patient at increased risk for developing clinical definite MS (93). There is no data to support repeated evoked potential testing for monitoring of ongoing disease.
Ocular Coherence Tomography
Ocular coherence tomography is a new technique which allows imaging of the retinal nerve fiber layer (RNFL) thickness (94). It is a sensitive, safe, and reproducible measure that allows analysis of features such as nerve fiber loss, ganglion cell loss, and macular edema. It is being used as an independent measure in clinical trials of medications in MS, and may be a robust marker of fiber loss indicating disease progression (95, 96). Its role in clinical decision making is still being evaluated (95).
Lumbar Puncture
Lumbar puncture and CSF analysis are less often performed today, as improvements in imaging have generally replaced this invasive procedure. However, when done, identification of oligoclonal bands and increased IgG synthesis are associated with a positive diagnosis of MS (97). These findings indicate activity of the immune system in and around the CNS compartment, and as such are not specific for MS. Other diseases such as lupus, Lyme disease, and neurosarcoidosis also may show oligoclonal banding. Studies of the diagnostic accuracy of MRI, evoked potentials, and CSF have shown that if two of three of these tests are positive, the third does not add significantly to the diagnosis (98). Neurologists are reducing the frequency of CSF tests in patients with otherwise typical MS and in whom MRI and evoked potentials are characteristic. CSF studies are most useful in atypical presentations, where the MRI is nonspecific, and where other diagnoses are being considered.
Electrodiagnostic Testing
In general there is no role for electromyography and nerve conduction testing in MS. The peripheral nervous system is usually spared in MS. There are some studies indicating involvement of the proximal aspect of the peripheral nervous system in patients with severe MS, sometimes affecting phrenic nerve function (99). There are rare patients with central and peripheral demyelination in which electromyography and nerve conduction studies would be useful (100).
MEDICAL MANAGEMENT
Corticosteroids
Corticosteroids and adrenocorticotropic hormone (ACTH) have been used in MS therapy for years, well before randomized controlled trials were undertaken in MS therapy. An early randomized trial of ACTH versus placebo for exacerbations of MS showed improvements in disability scores at 4 weeks in patients treated with ACTH compared with patients treated with placebo (101). Over the past 20 years, various studies have shown a similar short-term effect of steroid therapy during exacerbations. Three major randomized equivalence studies showed that IV methylprednisolone was similar to ACTH in terms of efficacy with a more convenient dosing schedule (102, 103, 104). Questions about the efficacy of IV methylprednisolone lead to three randomized trials versus placebo that reconfirmed the significant short-term benefit of various IV methylprednisolone regimens versus placebo on neurological function (105, 106). A systematic review found a sufficient body of evidence to support the use of IV methylprednisolone in acute exacerbations of MS (107). The usual place for IV steroids is for acute exacerbations that cause functional deficits. The use of steroids has to be balanced against the considerable long-term risks which include occasional anaphylaxis against IV methylprednisolone, osteoporosis, cataracts, aseptic necrosis of the hips or shoulders, diabetes mellitus, etc.
Interferons
Three IFN-β agents are FDA approved for MS. They have similar efficacy in reducing relapse frequency and new MRI activity and have similar side effect profiles.
IFN-β1b (Betaseron) was approved by the FDA for use in RRMS and became available in limited quantities in the United States in late 1993 (49). IFN-β1b was tested in patients with up to a moderate degree of disease severity. A three-armed phase III trial clearly demonstrated a dose-effect curve. (Comparison groups placebo, 1.6 million international units, 8 million international units s.c., alternate day dosing.) In the high-dose group, a 34% reduction in exacerbations and an 83% reduction in active MRI lesions were noted. Side effects of IFN-β1b include injection site reactions, elevation of liver function tests, and rare incidences of autoimmune hepatitis, glomerulonephritis, and immune thyroiditis. There may be an increase in depressive symptoms in patients on IFN therapy. Common side effects include flulike symptoms the night after the injection, which can usually be reduced by anti-inflammatory medications or
acetaminophen. Up to 35% of patients may develop neutralizing antibodies to IFN-β1b which seem to inhibit the efficacy of this medication. In patients with more severe functional symptoms spasticity may increase in IFN therapy.
acetaminophen. Up to 35% of patients may develop neutralizing antibodies to IFN-β1b which seem to inhibit the efficacy of this medication. In patients with more severe functional symptoms spasticity may increase in IFN therapy.
In 1996, IFN-β1a (Avonex) became FDA-approved for RRMS, based upon a phase III trial demonstrating 18% reduction in exacerbations and a 33% reduction in MRI activity (50). In this study, there was a 37% lower risk of sustained progression of disability as measured by the Expanded Disability Status Scale (EDSS). Side effects include flulike symptoms after the injection, and a lower rate of side effects similar to IFN-β1b. Five to ten percent of patients may develop neutralizing antibodies to IFN-β1a (50).
A third IFN-β is approved for the US market is IFN-β1a, s.c., three times a week (Rebif) (51). This agent demonstrated efficacy similar to IFN-β1b.
Glatiramer Acetate
Glatiramer acetate (GA) (Copaxone) is a mixture of synthetic polypeptides consisting of four amino acids in a specific molar combination. The proposed mechanism is binding to class II major histocompatibility complex with consequent inhibition of myelin-reactive T cells. GA was tested in RRMS patients with an EDSS of up to 5.0 and demonstrated a 29% reduction in exacerbations (52). A subsequent study has shown GA to reduce active lesions on MRI by 35% (109). Side effects include skin injection site reactions, rare arthritic symptoms, and an idiosyncratic reaction in 1/3,000 injections with chest tightness and shortness of breath lasting a few minutes.
Natalizumab (Tysabri)
Natalizumab contains humanized neutralizing IgG4-κ monoclonal antibodies against leukocyte α4 integrins. By blocking α4 integrins, natalizumab reduces the movement of mononuclear leukocytes into the CNS and small intestine. This medication is given as a monthly IV injection. One pivotal trial of natalizumab showed a reduction in sustained disability from 29% to 17% at 2 years, as well as a reduction in relapse frequency by approximately 70% and reduction in MRI new gadolinium enhancing lesions at year 2 by 92% (110). Two MS patients who were also on IFN therapy developed progressive multifocal leukoencephalopathy (PML) (111
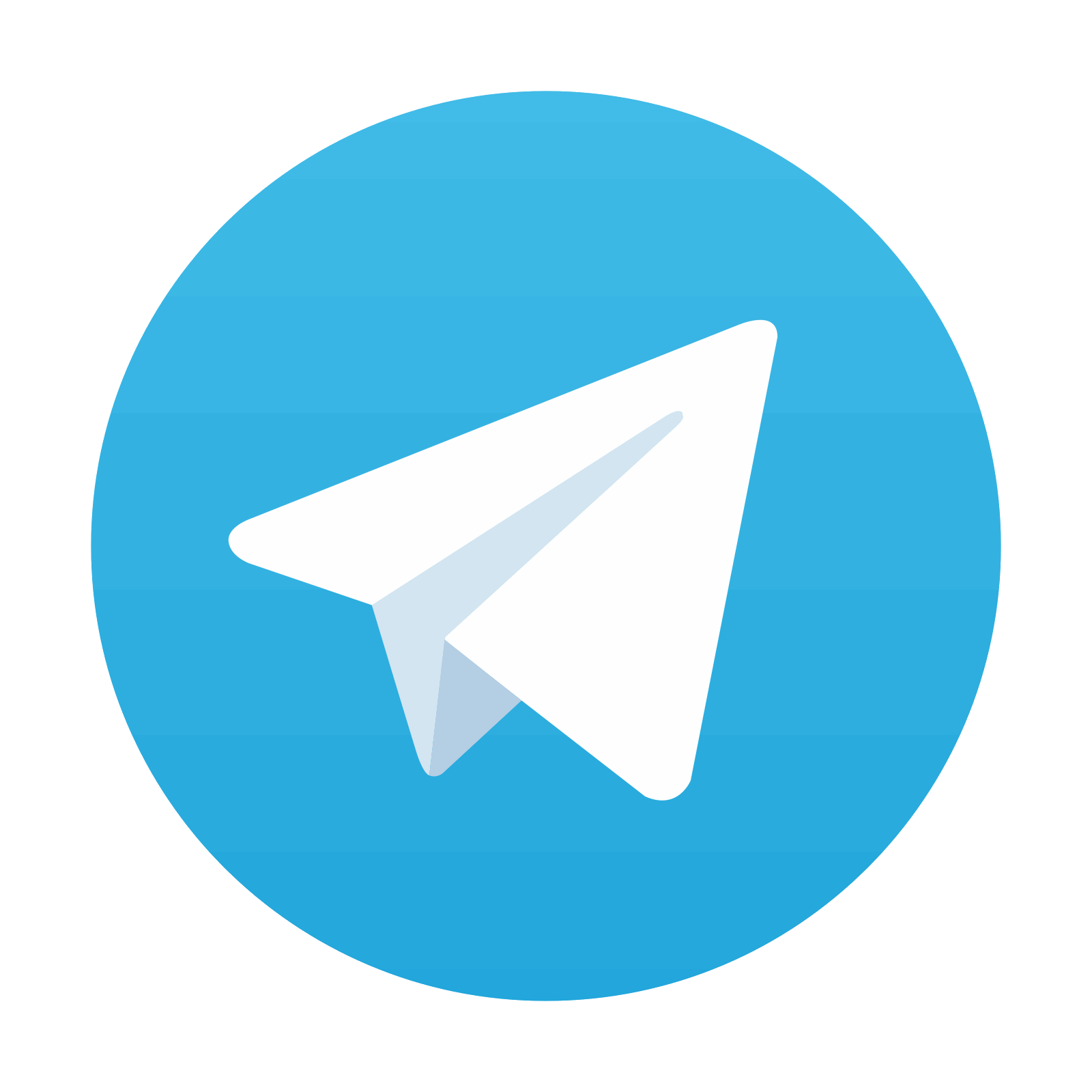
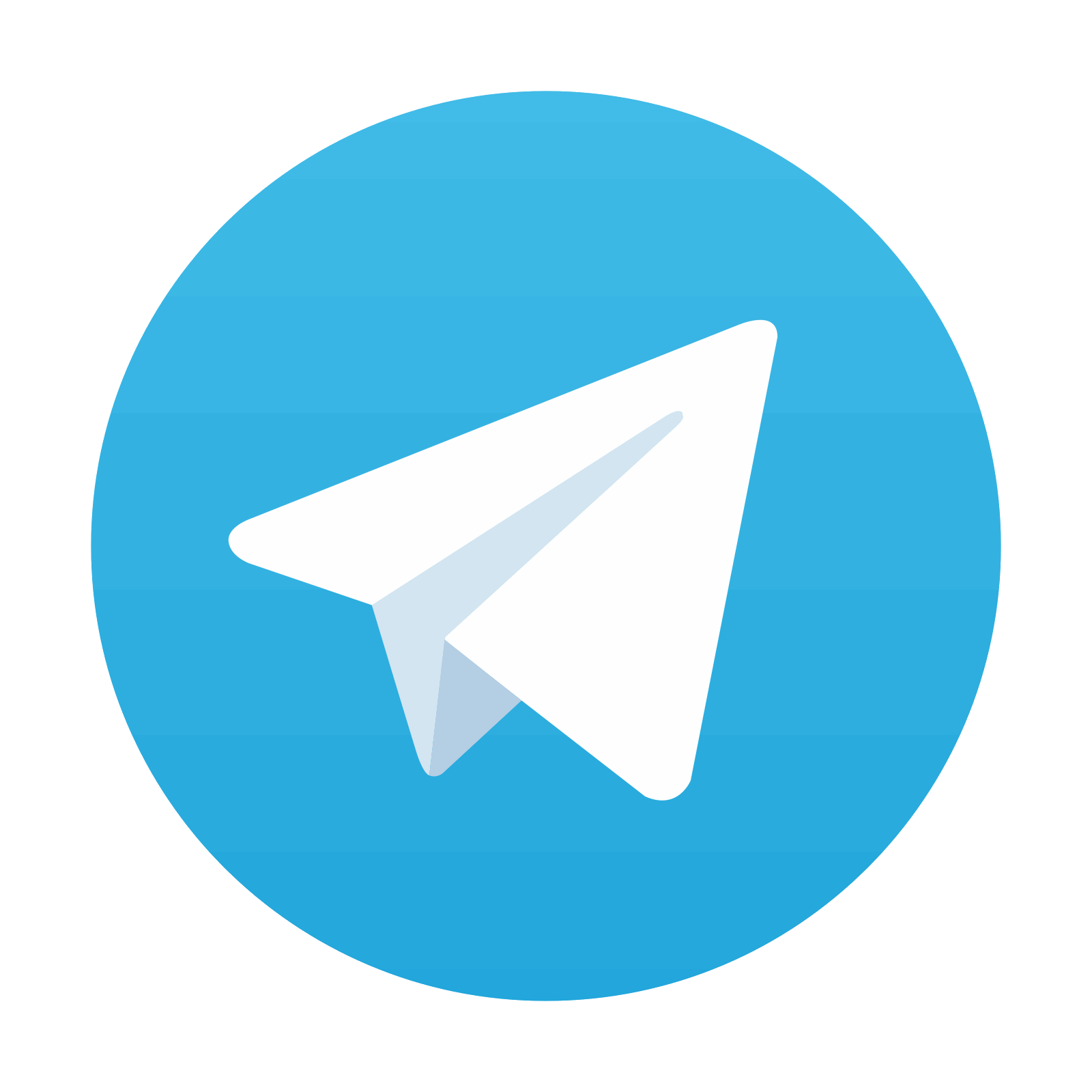
Stay updated, free articles. Join our Telegram channel

Full access? Get Clinical Tree
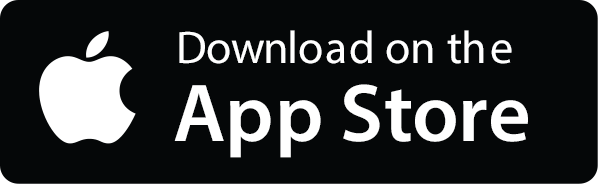
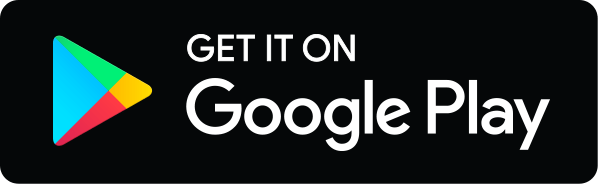
