Materials in Hip Surgery
Ultra-High-Molecular-Weight Polyethylene
Stephen Li
Key Points
Introduction
Ultra-high-molecular-weight polyethylene (UHMWPE) has been the bearing material of choice in total hip replacement for over 50 years. It is the goal of this chapter to provide the practicing surgeon with basic knowledge and science of UHMWPE and to discuss key issues of UHMWPE as they apply to clinical performance.
UHMWPE has been the bearing material of choice in total hip replacement since its first use by Sir John Charnley in 1962. In this chapter, we will review the history of UHMWPE in joint replacement, the current status of the technology, and possible future directions.
The History of Bearing Materials
Since the advent of total hip replacement, relatively few polymeric materials have been actually used in total joint replacement. Scales provided the early history of bearing materials in total hip replacements in 1967. One of the first attempts at joint replacement was undertaken in 1890 by Berliner Professor Themistocles Glück (1853-1942). Glück produced an ivory ball and socket joint that he fixed to bone with nickel-plated screws. During this same period, Sir Robert Jones (1855-1933) used a strip of gold foil to cover reconstructed femoral heads. One amazing report indicated that one patient retained effective motion at the joint.4 In 1936, brothers Robert (1901-1980) and Jean (1905-1995) Judet introduced polymethylmethacrylate as the first synthetic polymeric material used as replacement for the femoral head. However, these acrylic devices became loose quickly because of high wear rates.
The first total hip replacement is credited to Philip Wiles (1899-1966), who developed a stainless steel, metal-on-metal device in 1938. In 1940, Austin Moore (1899-1963) and Harold Bohlman (1873-1979) first implanted Vitallium (cobalt-chrome-molybdenum alloy; Dentsply Austenal, York, Pa) in a 46-year-old, 250-pound male patient. The metal implant was made from molds, which were based on radiographic measurements. The implant was approximately 12 inches long and was bolted to the external surface of the femur.
In the 1940s through the 1960s, the development of metal-on-metal hip replacements continued with the McKee-Farrar and Ring prostheses. However, these devices fell out of favor with the introduction of the Charnley device. However, the metal-on-metal devices went through a renaissance starting in the 1980s and, once again, are used extensively.
Charnley initially chose polytetrafluoroethylene (PTFE) as a bearing material based on its general chemical inertness and low coefficient of friction. The first Charnley design to use PTFE was what we now refer to as a surface replacement prosthesis. The femoral head was covered with a “cup” of PTFE, and the acetabulum was lined with another layer of PTFE. This was a polymer-against-polymer total hip replacement. He next developed a procedure that replaced the femoral head and neck with a Moore femoral stem and a 42-mm ball to articulate against the PTFE cup. Last, he introduced the 22.22512,13-mm (″) acetabular head and the use of acrylic bone cement for fixation. The (PTFE) materials that Charnley used are widely and incorrectly referred to as Teflon, the most familiar name in PTFE materials. Although it appears that Teflon may have been used for a short period, in private correspondence, Charnley identified the actual materials specifically as Fluon G1 and Fluon G2, products of Imperial Chemical Industries.14 Clinical failures with Fluon PTFE acetabular cups generally occurred within 1 to 2 years and were attributed to the low creep and abrasive wear resistance of PTFE resins. Charnley found that the wear of PTFE against a stainless steel head provided 7 to 10 mm of wear in less than 3 years. Charnley’s results were first reported in 1972.
Credit is provided to Harry Craven, an engineer who worked with Charnley, who tested a material termed high-molecular-weight polyethylene (UHMWPE) that was given to him by a plastic gear salesman. This selection was remarkable given that the first hips using this material were implanted in 1962, and it has been the material of choice ever since.1 As will be discussed, UHMWPE had just been discovered a few years before, and it had only recently become commercially available for industrial applications.
UHMWPE
In the early literature, UHMWPE is often incorrectly called high-density polyethylene (HDPE). To make matters more confusing, HDPE was actually used in a few rare instances. Significant property and performance differences between UHMWPE and HDPE have been observed. HDPE would be a poor bearing material for joint replacement, because it has less wear resistance and lower resistance to fracture and fatigue.17 With a few known exceptions, which will be discussed later, very few implants were actually made of HDPE.
The polymeric bearing material of choice remains UHWMPE. Fabricated sheet and bar forms of UHMWPE were first introduced during the K-fair in Dusseldorf in 1955 under the name of RCH (Ruhrchemie) 1000. The original polymer resin (particles) from which these forms were made were named GUR (granular UHMWPE Ruhrchemie) resins. The material was invented at the site of Ruhrchemie in Oberhausen, Germany, where the first pilot plant was built in 1955, followed by the first commercial scale production plant in 1960.
UHMWPE is synthesized from the polymerization of ethylene. This is done via Ziegler-Natta catalysis, which allows the polymer to form linear chains. The Ziegler-Natta catalyst is made from TiCl4 and an aluminum alkyl compound; hence the small amounts of Ti, Cl, and Al that are always found in elemental analysis of UHMWPE. The reaction is typically conducted at low polymerization pressures and temperatures, typically between 4 and 6 Bar pressures and between 66° C and 80° C. These mild conditions maximize the molecular weight (long chains) of the polymer and minimize branching. With some grades, a very fine calcium stearate powder can be added to serve as an oxidant that minimizes yellowing of the material during subsequent fabrication processes.
UHMW Resins
Since 2002, the main supplier of the UHMWPE materials used in orthopedics is Ticona (Auburn Hills, NJ), a business unit of Celanese. However, the history of suppliers and grades of UHMWPE is somewhat confusing. Before approximately 2002, there were two major suppliers of UHMWPE for medical implants, and each supplier also provided several grades of GUR resins.
As stated previously, Ruhrchemie first introduced RCH1000 in the 1950s. The first products fabricated from RCH1000 were made from GUR 412 resin. When the material was sold for medical applications, it was called RCH1000C and was made from GUR 112 resin. Although the physical properties of RCH1000 and RCH1000C were much the same, RCH1000C had lower levels of extraneous contaminants, and the final shaped material was ultrasonically checked to ensure that there were no unsintered areas. As the market for medical applications grew, fabricated forms of UHMWPE for medical applications were named Chirulen, and powder sold for medical applications of RCH1000C was renamed Chirulen P. In 1988, Ruhrchemie merged with the Hoechst/Celanese Corporation, which included operations in the United States in Bishop, Texas. In 1999, Celanese and another wholly owned company by Hoechst—Ticona—were separated from the Hoechst/Celanese Corporation. Ticona is now part of the Celanese Corporation and manufactures GUR UHMWPE for use in medical applications. This sequence of business changes had direct influence on the grades of materials sold to the medical community.
In the early 1990s, Hoechst/Celanese developed a four-number naming system for GUR resins (e.g., 4150 GUR) based on the manufacturing location, the molecular weight, and the presence of additives:
• The second digit indicated the presence (1) or absence (0) of calcium stearate.
• The third digit indicated a molecular weight of 2 million (2) or 5 million (5).
• The fourth digit was always 0, and its meaning is not generally known.
For example, the designation “GUR 4120” indicates that the resin was manufactured in the United States (4) with the addition of calcium stearate (1), and it has a molecular weight of approximately 2 million. In the literature, the last “0” is often omitted in material descriptions; thus GUR 4120 resin is termed “GUR 412.” At this time, sheets sold for medical applications in Europe were termed “Chirulen P.” In the 1990s, the United States produced resins for medical applications that had “HP” added to their GUR name to indicate high purity. Thus, GUR 4150 sold for medical applications was designated “GUR 4150HP.” However, in 1998, the names of all the resins were consolidated. All medical resins now started with the number 1. The other three numbers still have their original meaning. The four available resins are now designated 1150, 1050, 1120, and 1020. However, because of market-driven purchasing, 1050 and 1020, the calcium stearate–free grades, are the major resins sold for medical applications.
1900 Resins
The other UHMWPE resin used in joint replacement was originally sold under the name “Hifax 1900,” then “Himont 1900,” and now, simply, “1900 resin.” No 1900 resins have been sold to U.S. manufacturers since 2002. However, both Zimmer Orthopedics and Biomet made large purchases of 1900 just before it became unavailable for use in orthopedics, which allowed both companies to continue for several years the manufacture of implants traditionally made from 1900.
Similar to the GUR resins, several grades of 1900 were sold. They were designated “Hifax 1900,” “Hifax 1900H,” “Hifax 1900L,” and “Hifax 1900CM.” The differences in these grades were seen in their average molecular weight.
The grades of UHMWPE that were being supplied or used by orthopedic companies in 1995 are listed in Table 5-1. By this time, names containing “RCH1000” and “Chirulen” were no longer used. In 2010, the only resins available to orthopedic surgeons are GUR 1050 and GUR 1020 from Ticona. In addition to these resin names, in some instances, manufacturers have provided separate trade names to identify their products. These names have been used in the literature to describe different implants for correlation with clinical and laboratory performance, and are described more fully in Table 5-2.
Table 5-1
Available UHMWPE Resins, 1995
UHMWPE, Ultra-high-molecular-weight polyethylene.
Table 5-2
Names for Non–Highly CrossLinked UHMWPE Resins and Products
UHMWPE, Ultra-high-molecular-weight polyethylene.
Characteristics and Properties of UHMWPE
Molecular Weight
A key material property for UHMWPE is its molecular weight, because this property distinguishes UHMWPE from the other forms of polyethylene and determines the properties and behavior of the polymer. The molecular weight of UHMWPE is generally determined experimentally by measuring the relative viscosity of solutions of the material at different concentrations. However, the higher the molecular weight of UHMWPE, the more difficult it is to dissolve in suitable solvents. For this reason, it is difficult to determine the molecular weight of any polymer when the value exceeds 1,000,000.
The specific method used to determine the molecular weight of UHMWPE is described in American Society for Testing and Materials (ASTM) Standard D-4020. In this method, a dilute solution of UHMWPE is made by dissolving a small amount of UHMWPE powder in decahydronapthalene.
The relative viscosity of the solution is determined with a capillary viscometer, which measures the rate of flow of the solution through a small orifice. The measured value of the relative viscosity is then used to estimate the average molecular weight of the polymer using the Mark-Houwink equation:
where η is the intrinsic viscosity, M is the average molecular weight, and K and a are constants that vary with the solvent used and the temperature at which the measurements are made.
Once the value for [η], is determined, the molecular weight can be estimated using the following equation:
Care must be taken in comparing molecular weight values from different sources, because it is possible that different equations were used, resulting in the possibility of different molecular weight values from the same viscosity measurements.
Physical Properties
The standards for physical properties are provided in ASTM DF-648, Standard Specification for Ultra-High-Molecular-Weight Polyethylene Powder and Fabricated Form for Surgical Implants. In this standard, requirements are given for the powdered resins provided for three types of UHMWPE, designated types 1, 2, and 3, where type 1 comprises GUR resins with molecular weight of approximately 2 million (1020), type 2 GUR resins with molecular weights of approximately 5 (e.g., 1150), and type 3 1900 resins. Requirements for the powder resin of these three types are presented in Table 5-3.
Table 5-3
ASTM F648 UHMWPE Specifications
UHMWPE, Ultra-high-molecular-weight polyethylene.
Fabrication Methods
As described previously, UHWMPE is synthesized as a powder, which is formed into solid shapes by one of three methods: extrusion and machining, sheet compression molding and machining, and direct compression molding.
Extrusion and Machining.
In this process, powder is continuously fed into a heated chamber. A ram pushes this powder into a heated cylindrical barrel, retracts, leaving the chamber empty, and waits for the next fixed amount of powder. The process is continuous, and each push of the ram advances the polyethylene through the heated barrel. In this manner, the powder is consolidated into a continuous cylindrical rod, which is then cut into 10-foot lengths for sale. Implants are machined from this cylindrical bar stock.
Sheet Compression Molding.
In this process, the UHMWPE powder is introduced into a large rectangular container, typically measuring 4′ × 8′. A platen large enough to cover the entire container is used to apply pressure to the heated container. In this manner, sheets are formed that are up to 8 inches thick and measure up to 8 feet in length and width. Implants can be machined from these molded sheets.
Direct Compression Molding.
Powder is placed into a mold in the shape of the final component and then is heated under pressure to achieve consolidation. After the mold has cooled, the net shape implant is removed and packaged. Devices formed in this fashion have no external machining lines and often exhibit a highly glossy surface finish. The properties of directly molded components are different from those of components produced by extrusion or compression molded sheet. This will be discussed in a subsequent section. The production advantages of direct compression molding are that it is possible to make very complicated geometries in a single step, the surface finish of the polyethylene is extremely smooth, and the process imparts beneficial properties to the UHMWPE. Disadvantages are that the process is relatively slow (e.g., expensive), and individual molds must be made for each product. Reports indicate that direct molded components have lower wear rates than their corresponding extruded bar/compression molded sheet and machining components. Bankston and associates reported a 50% reduction in the average clinical wear rate of directly molded components compared with machined components (.05 mm/yr vs .11 mm/yr, respectively). These same differences in wear rates were noted in hip simulation wear studies.
As a final processing step, some suppliers anneal their bars after ram extrusion to presumably remove any residual stresses. The conditions of the annealing are proprietary, but they involve heating the material, which complicates the thermal history of the bar.
Sterilization Methods and Oxidation
Since it became commercially available in the late 1960s, the dominant method used for sterilization of UHMWPE components has been gamma irradiation from a Co60 source. In addition to sterilizing the material, the gamma rays create free radicals within the UHMWPE that can react with other free radicals within the polymer, and with oxygen from the atmosphere. These reactions are generally described as oxidation reactions. As will be seen, over the past 10 years, the issue of oxidation has greatly influenced methods of sterilization and methods used to improve the performance of UHMWPE.
The mechanisms of oxidation have been discussed at length elsewhere and will only be summarized here. Exposure of UHMWPE to gamma rays or electron beams can cause rupture of the carbon-carbon or carbon-hydrogen bonds. In either case, two free radicals are formed for each bond that is broken. These free radicals generally are very reactive and will undergo one of the following reactions:
These different reaction pathways lead to chemical changes that can be measured in a variety of ways. Infrared spectroscopy can be used to detect the presence of chemical moieties such as carbonyl groups and double bonds, which appear after oxidation has occurred. In 1990, Fourier transform infrared (FTIR) was introduced as an updated form of the dispersive infrared method used by Eyerer. FTIR provided more sensitive than traditional infrared methods and allowed determination of the relative amounts of oxidative products as a function of depth from the surface of a sample.27 This method is now described in ASTM 1421. It is important to note that values of oxidation measured with FTIR can be highly variable, with differences between test laboratories exceeding 129% under certain conditions. This large variability indicates that care must be taken in comparing values of the oxidation index of UHMWPE samples tested in different laboratories or at different times.
Oxidation also leads to increases in density of the UHMWPE, hence the reported change in density of UHMWPE samples with shelf aging in air after gamma irradiation with a dose of 25 to 40 KGy. The change in density is a slow process, with change of approximately 0.003 g/mL/yr noted. Density is measured with the use of a density gradient column and a protocol provided in ASTM D-1505. It should be noted that quality defects such as subsurface white bands and nonconsolidated particles appear at density values >0.95 g/mL.
It has been known since the late 1970s from the study of retrieved implants that UHMWPE oxidizes after gamma sterilization, and that physical properties may be adversely affected. However, UHMWPE oxidation was not considered a major clinical factor in limiting the performance of total hip replacement at that time. It was not until the early 1990s that interest in oxidation was rekindled, as factors that could influence the generation of particulate debris were sought out. If postirradiation aging is severe enough, the quality of a polyethylene component can be adversely affected, as is evidenced by the presence of nonconsolidated particles or by polyethylene exhibiting subsurface white bands on cross-sectioning. Collier and coworkers reported that 20% of acetabular components implanted for 4 years or longer exhibited signs of fracture and fatigue due to oxidation. Further, it was demonstrated that, over time, the mechanical properties of gamma-sterilized UHWMPE components that were not implanted were also reduced through the effects of oxidation. These reports and others showed that oxidation of UHWMPE after gamma irradiation in air could reduce its strength and fracture resistance, and that this process began immediately after irradiation and continued for years. However, because the rate of this process was relatively slow, guidelines were adopted that recommended that UHMWPE components exposed to gamma irradiation in air should be implanted within 5 years of sterilization. By 1996, most manufacturers had modified the gamma sterilization process or had abandoned gamma sterilization in favor of alternative methods in an attempt to minimize the effects of postirradiation oxidation. Common methods that did not employ ionizing radiation included sterilization with ethylene oxide or gas plasma; however, because these methods did not provide any cross-linking, they did not lead to improvement in the wear properties of UHMWPE.
It now appears that in the vast majority cases, oxidation does not significantly or adversely influence the clinical wear rate of UHMWPE components. This view is based on the following considerations.
The rate of postirradiation aging of UHMWPE outside the body is very slow. Typically, components must be stored for longer than 4 years under ambient conditions for visible signs of degradation to appear, such as the development of unconsolidated polyethylene particles and subsurface bands of embrittled material as observed in sectioned components. Because most devices are implanted within 4 years of sterilization, the amount of oxidation is generally low. Although ex vivo oxidation of UHMWPE has been well studied, in vivo oxidation is a more controversial topic. The conclusions of literature reports range from claims that little or no oxidation occurs in vivo to the assertion that oxidation is actually faster in vivo than ex vivo.44 This subject has been difficult to elucidate because the oxidation state of retrieved implants is not known prior to implantation. Moreover, it has been reported that other factors such as mechanical loading, wear, and the polyethylene manufacturing process can significantly influence the rate of oxidation of UHMWPE in vivo.
Few reports convincingly correlate oxidation of UHMWPE to increased wear rate. Hip simulation studies of acetabular cups with postirradiation aging times ranging up to 10 years showed that significant oxidation of UHMWPE did not adversely affect the rate of wear.46 In a report on the analysis of wear and oxidation level of 100 retrieved Charnley acetabular cups, it was noted that there was no correlation (r2 < 0.1) between degree of oxidation of the polyethylene and radiographically or directly measured wear.48
In three reports on hip simulator tests, the wear of acetabular inserts was reduced by 30% to 46% after gamma irradiation (both in air and in an inert atmosphere) in comparison with rates after sterilization with ethylene oxide gas.50 This was due primarily to the beneficial effects of cross-linking on the mechanical properties of UHMWPE, which is a by-product of gamma ray exposure, in addition to increased wear resistance and embrittlement with aging.
Dramatic loss of fracture toughness and fatigue resistance of UHMWPE with oxidation has led to catastrophic failure of some designs of implants that had regions of exceedingly high stress concentration. A well-publicized example is the acetabular cup system (ACS) liner, which was recalled by DePuy Orthopaedics (Warsaw, Ind) in 1989 because of rim fractures. This component was designed with a metal-backed shell that provided support only for the polyethylene insert around the rim. The liners that fractured in clinical service had a wall thickness of only 2.5 mm, which, in combination with high levels of oxidation, led to rim fractures in a small percent of cases.
The current view is that high-energy irradiation is the preferred method of sterilization of UHMWPE. Adverse effects of postirradiation aging are limited to decreases in fracture and fatigue resistance, without reduced wear resistance. Postirradiation issues can be minimized by irradiating components in a low-oxygen environment (e.g., vacuum, nitrogen, argon). The use of nonirradiation methods such as ethylene oxide gas has provided products that will not oxidize as the result of irradiation but have higher wear rates caused by lack of cross-linking. The issue of oxidation, however, has greatly influenced the adoption of methods of treatment of UHMWPE with higher doses of radiation used to minimize wear. This will be discussed in detail later under the section, “Highly Cross-Linked UHMWPE.”
Modifications to Ultra-High-Molecular-Weight Polyethylene
Several notable efforts have been undertaken to improve the clinical performance of UHMWPE through material modifications. Four of these efforts will be reviewed here: carbon fiber reinforcement, increased crystallinity of UHMWPE, cross-linking of UHMWPE, and the addition of antioxidants.
Carbon-Reinforced
In the late 1970s, in an effort to reduce creep (cold flow) of UHMWPE and to decrease wear, Zimmer Inc. (Mendham, NJ) developed a material called Poly II, which was a reinforced composite of UHMWPE and carbon fibers. This composite was made by directly molding short carbon fibers and polyethylene powder into tibial inserts, patellar buttons, and acetabular components. The addition of carbon fibers led to increased compressive and flexural yield strength, tensile properties, and creep resistance, and to improved wear resistance. The magnitude of these changes in properties increased with the amount of fiber added.56 However, after these materials had been introduced for clinical use, they were found to have lower fatigue resistance than UHMWPE. Additionally, the materials suffered from manufacturing problems associated with incomplete molding. This led to poor adhesion of fibers to the polyethylene matrix, as evidenced by apparent fiber pull-out during in vivo use. Efforts to improve adhesion between fibers and matrix, such as changing the geometry of the fibers or the use of coatings, did lead to significant clinical improvement. Surface damage scores of retrieved Poly II components were also higher than those of unreinforced UHMWPE components. Its use was discontinued approximately 7 years after its introduction into the marketplace. It is interesting to note that no reports have described the long-term clinical performance of these devices.
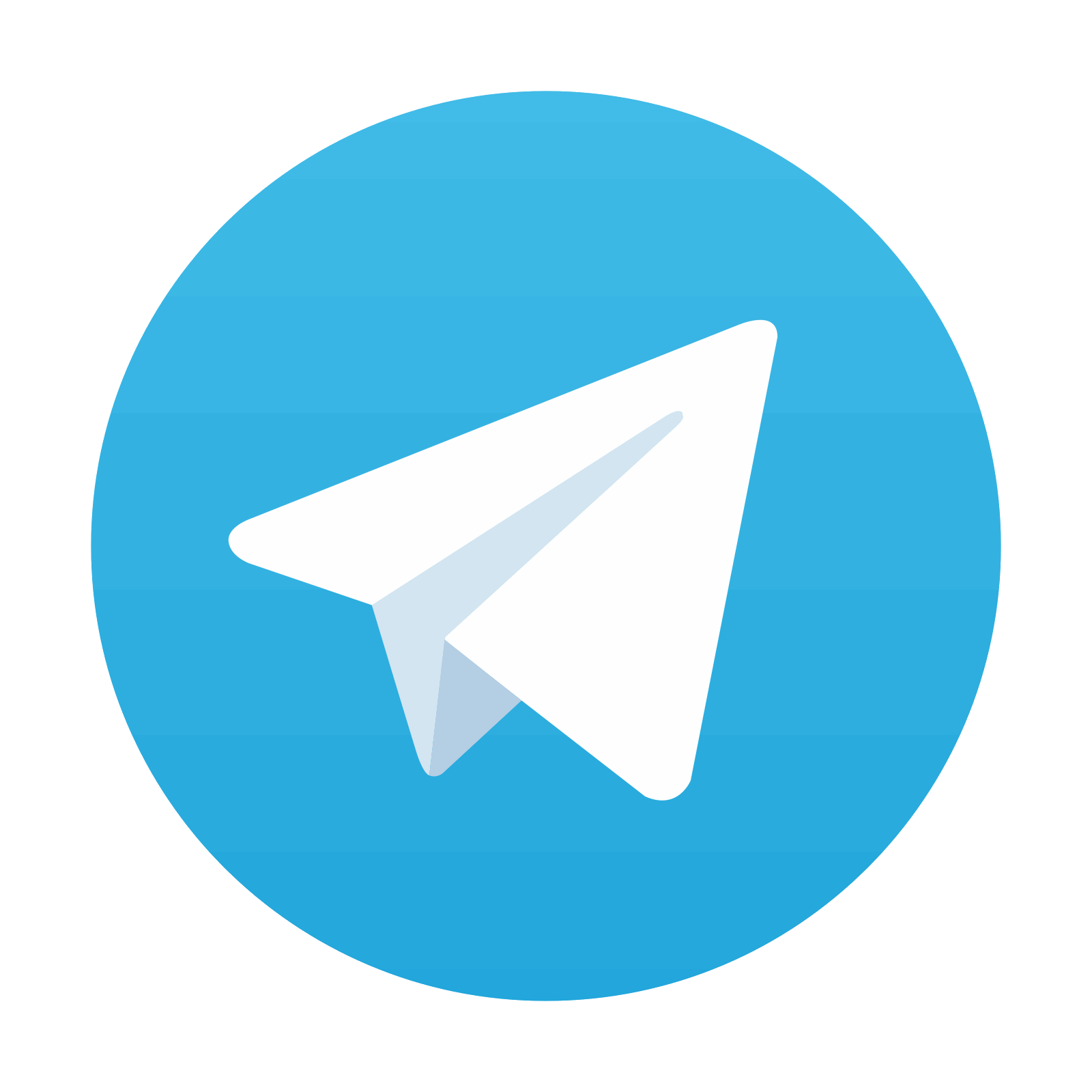
Stay updated, free articles. Join our Telegram channel

Full access? Get Clinical Tree
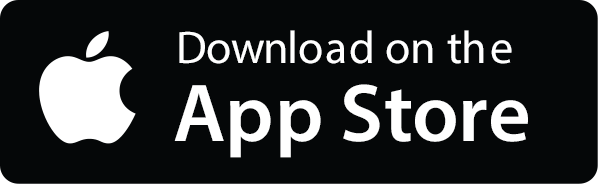
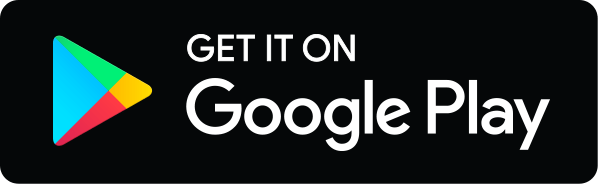