CHAPTER 51 Lumbar Total Disc Replacement
Lumbar spinal fusion is a time-tested, proven successful procedure for alleviating symptoms in patients with unremitting low back pain from various causes. Immobilizing an unstable motion segment, as in a degenerative or isthmic spondylolisthesis, is the most common and least controversial reason for performing a lumbar spinal fusion. Painful disc degeneration is a more controversial indication for spinal fusion because methods for identification of an intervertebral disc as the pain generator are imperfect. Multiple studies have shown successful pain relief of symptomatic lumbar degenerative disc disease with lumbar fusion.1–4 Fusion also creates an anatomic situation with potentially negative consequences for long-term lumbar function, however. By limiting motion of the affected motion segment, additional stress is created at adjacent motion segments, leading to an increased incidence of adjacent level degeneration.5–7 Often this degeneration becomes symptomatic and is a common cause of late failure after lumbar fusion.7–10 In addition, spinal fusion fixes the sagittal alignment at that motion segment, which can affect the adjacent segments over time and be responsible for adjacent segment degeneration.11 A third potential cause of adjacent segment degeneration is the potential iatrogenic injury of the adjacent level with surgery, especially injury to the cephalad facet joint with placement of pedicle screw instrumentation.
With these clinical and anatomic limitations on use, lumbar disc arthroplasty is appropriate only for a small percentage of overall lumbar fusion patients. Various studies have shown potential lumbar TDR candidates limited to 0% to 25% of fusion patients, depending on the specific demographics of the surgical practices being studied.12,13 Many TDR implants have been shown to retain flexion-extension motion at the operative motion segment over time.14–20 This chapter addresses the general concepts of lumbar total disc arthroplasty and focuses on the specifics of lumbar TDR implants that currently have U.S. Food and Drug Administration (FDA) approval or are completing FDA-sponsored multisite investigational device exemption (IDE) trials in preparation for FDA approval.
Historical Devices
The origins of disc replacement date back to the late 1950s, with the first early attempts at disc reconstruction being accomplished by the injection of acrylic bone cement within the nucleus after discectomy.21–23 Around the same time, Fernstrom24 began implanting stainless steel spheres into the intervertebral disc space through a posterior approach after laminotomy and discectomy. Low back pain was associated with progressive disc height loss, and so the goal of these early devices was to restore disc height and maintain spinal motion. Fernstrom24 published his results in 1966, showing fair clinical results at 2 years postoperatively. The long-term outcomes of this device showed a high failure rate, however, secondary to subsidence of the implant with loss of motion, foraminal narrowing, and pain.
In the 1980s, artificial discs were designed using metal endplates and an intervening elastomeric rubber or plastic core, creating metal-plastic and rubber articulations among unattached components. The elastomeric discs were developed by Stefee and Lee and colleagues.25–29 Only the Stefee disc (Acroflex, Acromed) was implanted clinically, but it was soon discontinued because of catastrophic failure of the rubber core.25 Articulating discs include the SB CHARITÉ and the Marnay disc (later renamed the Prodisc I, precursor to the current Prodisc-L). Both discs enjoyed clinical success and are discussed later in this chapter. Another older implant design that never progressed to clinical application included the use of hinged spring articulation in all metal components. The newer, “modern,” designs that are discussed in more detail in this chapter draw upon the continued Orthopaedic experience with large joint replacement with respect to articulating materials, component geometry, and component constraint to minimize the risk of mechanical failure of the arthroplasty device or late failure of the supporting structures of the motion segment.
Biomechanical Considerations
Most of the forces seen across the motion segment are transmitted through the disc space. Force distribution across the disc and facet joint is posture dependent, however. Ledet and colleagues30 performed real-time measurements of forces across the disc space using an interbody force transducer. In this study, forces across the intervertebral disc are highest when the center of gravity is shifted forward and during twisting motions. Forces across the intervertebral disc decrease when the center of gravity is shifted posteriorly. The corresponding postures show high forces across the disc space in sitting positions with the highest forces seen during sitting with the torso flexed and twisted. Standing and lying in the supine position resulted in progressively lower forces at the disc space. A fused segment has no effect on the force transmission to the adjacent level but increases the range of motion of the adjacent level.31 The body attempts to preserve the total range of motion of the lumbar spine by compensating through the adjacent levels.
The motion segment does not act as a pure hinge in that the axis of rotation changes slightly with flexion and extension.32,33 Each lumbar vertebral level has a different location of the instantaneous axis of rotation for a given movement. For the L1-5 disc spaces, the average instantaneous axis of rotation is slightly posterior to midline and just below the inferior endplate on a mid-sagittal image. At L5-S1, the instantaneous axis of rotation is within the disc space.34 Range of motion and type of motion vary by level. Flexion-extension ranges from 12.7 to 19.6 degrees with the highest motion occurring at the L5-S1 segment (Table 51–1).32 At the L4-5 level, the motion is mostly translational, whereas the motion is rotational at the L5-S1 segment. Most studies comparing disc arthroplasty with the normal motion segment focus on flexion and extension. The motion of each vertebral segment is not only multidimensional, but also rotation and translation are coupled to varying degrees by level.
TABLE 51–1 Flexion-Extension of Lumbar Spine
L1-2 | 12.7 degrees ± 2.47 |
L2-3 | 16.6 degrees ± 2.43 |
L3-4 | 16.7 degrees ± 2.36 |
L4-5 | 18.3 degrees ± 2.67 |
L5-S1 | 19.6 degrees ± 4.71 |
Implant Design Considerations
Constrained versus Unconstrained
The concept of constraint in TDR has evolved over time and is not the same as in other articulating joint replacements of the appendicular skeleton. Kostuik35 described constraint in terms of the implant’s potential to limit the natural range of motion of the vertebral motion segment for each of the various rotational and translational motions. An implant unconstrained for a certain motion would provide no limitation to that motion well beyond the normal physiologic range. Examples are the CHARITÉ, Prodisc-L, and Maverick discs, which all are ball-and-socket articulations that provide no inherent limitation of axial rotation. These implants do have differing constraint, however, for the other motions of the lumbar motion segment.
Generally, the effect of increasing constraint is to increase the overall stability of the motion segment. A consequence of increasing constraint may be to decrease range of motion and change the stresses seen at the surrounding structures. Increasing constraint has been shown to increase the forces seen at the endplates.34,36,37 Increased stress at the endplate can have an effect on loosening of the component over time, as is seen in large joint arthroplasty. The effect of increasing constraint on the facet joints is unclear. Finite element models have shown increasing stress on the facet joints with increasing constraint.36,38 In vitro analyses have shown increased facet joint stresses with decreased constraint.39 Although it is logical to think that decreased constraint would increase the stress at the surrounding structures, the inconsistency in the data suggest that other factors may also play a role. Increased stress at the facet joints may lead to degenerative changes and pain over time. Implant design must balance between stability of the implant and restoring normal biomechanics.
Bearing Surfaces
Although many potential bearing surfaces are available, the most commonly used are cobalt-chromium alloy on ultrahigh molecular weight polyethylene (UHMWPE) and cobalt-chromium alloy on cobalt-chromium alloy. Both bearing surfaces are well established in orthopaedics for their biocompatibility and good wear resistance. Both bearing surfaces are known, however, to have long-term failures. Experience with large joint arthroplasty has shown that wear particles can lead to osteolysis, subsidence, migration, and need for revision surgery.40–42 Nonetheless, the effect of wear particles within a joint have a different local effect compared with the epidural space.43 To date, significant problems with osteolysis owing to polyethylene debris have not been seen clinically with the metal-polyethylene articulating implants with more than 20 years of use. This may be due to the lack of true synovium surrounding the disc arthroplasty, which has been implicated in the osteolysis seen associated with loosening of hip and knee implants.
Wear patterns observed in retrieval analysis of cobalt-chromium alloy with UHMWPE disc arthroplasty designs show the same failure mechanisms that are seen in hip and knee arthroplasty.44,45 Cobalt-chromium alloy with cobalt-chromium alloy articulations also include the risk of systemic release of cobalt and chromium ions. Although the blood serum ion concentrations are similar to total hip arthroplasty, the long-term effect is unknown.46,47 Industrial exposure to heavy metals such as hexavalent chromium is known to increase systemic inflammation and carcinoma.48 The types of ions that are released from an implant are different and may not have the same effect. Sarcoma adjacent to metal implants is reported in the literature, but it is very rare.49 Metal hypersensitivity is a potential cause for persistent pain with this bearing surface.
Implant Stabilization
Material and implant design considerations are factors in implant stability. Early stability is generally provided for by implant design, including roughened surface coatings, spikes, keels, or screw fixation.50 Late stability is obtained through additional direct bony attachment to the implant via ongrowth or ingrowth into the surface coating. In addition, implants designed to maximize endplate surface area contact and promote force transfer to the peripheral aspect of the endplate, where the bone is strongest, minimize the risk of implant subsidence. Biologic surfaces have become the standard for noncemented acetabular fixation in total hip arthroplasty. Attempts at biologic coatings for total knee arthroplasty have failed secondary to loosening of the components.51 This high failure rate is likely due to micromotion at the bone-implant interface. Similar problems must be monitored for in the spine.
Clinical Indications for Lumbar Total Disc Replacement
In the United States, lumbar disc replacement is currently approved for the treatment of single-level lumbar degenerative disc disease in skeletally mature individuals with no more than grade I spondylolisthesis having failed at least 6 months of nonoperative treatment. Currently available implants are approved only for use from L3 to S1 (Prodisc L3-S1, CHARITÉ L4-S1). The contraindications for TDR are extensive (Table 51–2). A relative contraindication is significant facet joint degeneration, although this is difficult to grade.52 There are many reports of multilevel disc arthroplasty, including level 1 evidence in prospectively randomized clinical trials, but this is currently considered off-label use in the United States based on strict FDA-approved criteria.39,53
TABLE 51–2 Contraindications to Lumbar Total Disc Arthroplasty
Osteopenia or osteoporosis defined as dual-energy x-ray absorptiometry scan bone density measured T-score ≤−1.0 |
Preoperative evaluation for total disc arthroplasty should include plain radiographs with flexion and extension films, magnetic resonance imaging (MRI), computed tomography (CT) scan, and dual-energy x-ray absorptiometry (DEXA) scan in patients at risk for osteopenia.39 Discography is considered by many authors to be an important diagnostic criterion for lumbar TDR but was not a requirement for many of the FDA-sponsored clinical trials. In addition, more recent reports not only have questioned the efficacy of discography, but also have implicated it in accelerating the development of disc degeneration.54–56
Surgical Approach and Discectomy
Implant designs of most lumbar TDR devices require a direct anterior exposure of the intervertebral space; this is true of both prostheses currently available for use in the United States. This exposure can be obtained through either a transperitoneal or a retroperitoneal approach. Currently, a retroperitoneal approach is preferred because it minimizes the risk of postoperative ileus, bowel obstruction secondary to adhesions, and retrograde ejaculation (in men).19 In most cases, the approach is performed with a vascular or general cosurgeon. The exposure must be wide enough to expose the entire anterior surface of the disc space, requiring greater mobilization of the great vessels than is necessary for fusion. The final view of the disc must be directly anterior. Other prostheses in development or available for use outside the United States (e.g., Oblique-Maverick) use an anterolateral or direct lateral approach to the disc space, minimizing or eliminating the need for mobilization of the great vessels.
After exposure of the disc space, the disc is removed in entirety, including the anterior anulus and anterior longitudinal ligament, the entire nucleus pulposus, and the posterior anulus. To restore posterior disc height in cases with significant disc collapse, the posterior longitudinal ligament must be either released or resected. The outer lateral anulus is preserved. The cartilaginous endplate is removed taking care to preserve the subchondral bone. To recreate the proper center of rotation for the lumbar motion segment, most of the devices are placed abutting the posterior edge of the vertebral body. Proper posterior placement also helps to decrease the forces seen at the facet joints.57 Position of the implant is confirmed using intraoperative anteroposterior and lateral fluoroscopy throughout the procedure.
Specific Devices
CHARITÉ Artificial Disc
The CHARITÉ Artificial Disc (DePuy Spine Inc., Raynham, MA) is the first device to complete the U.S. FDA approval process and has the largest and the longest clinical experience. The device has a biconvex UHMWPE spacer that acts as a mobile core (Fig. 51–1). The two metallic endplates are made of a cobalt-chromium alloy, and each concave endplate articulates independently with the core. There are ventral and dorsal teeth on the endplates to aid in positioning and maintenance of final position, and the latest version of the device has a titanium and a hydroxyapatite coating.21
The FDA IDE study gave level 1 evidence showing the CHARITÉ disc replacement as equivalent to anterior interbody fusion with a BAK cage (Zimmer Spine, Minneapolis MN) filled with iliac crest autograft.14,15 In the study, 305 patients were randomly assigned to receive either the CHARITÉ lumbar disc replacement or anterior lumbar interbody fusion (ALIF) with a pair of cylindric threaded BAK cages and iliac crest autograft. Study levels included only L4-5 or L5-S1. For the CHARITÉ group, the Oswestry Disability Index (ODI) improved by 24.8 points from 50.6 preoperatively to 25.8 at 24 months. In the BAK fusion group, ODI improved by 22 points from 52.1 to 30.1. The visual analog score (VAS) improved from 72 points in the CHARITÉ group to 30.6 points at 24 months. For the BAK fusion group, the VAS improved from 71.8 to 36.3 points. These results were maintained at the 5-year follow-up.58 In this study, FDA success at 24 months was defined by success in all of four major criteria: (1) a 25% improvement in the ODI, (2) no device failure, (3) no major complication, and (4) and no neurologic deterioration. In the FDA IDE study, 57% of the CHARITÉ group and 46% of the fusion group met all four criteria.
Longer term retrospective level 3 clinical data are also available for TDR using the CHARITÉ device.59,60 Lemaire and colleagues59 reviewed 100 patients with a minimum 10-year follow-up. Of patients, 90% had a good to excellent outcome with 63% returning to heavy labor jobs and 91.6% overall returning to work. Mean flexion-extension was 10.3 degrees with 5.4 degrees of lateral bending. Five patients (5%) required arthrodesis secondary to either implant failure or facet arthrosis. There were two neurologic injuries (2%), one L5 nerve injury and one sexual dysfunction, both of which recovered. There were two cases of spontaneous fusion (2%), and two cases of adjacent segment degeneration (2%). David60 reviewed 106 patients implanted over a 6-year period with a minimum 10-year follow-up. Of patients, 82% had good to excellent outcomes; 89% returned to work with 77% returning to heavy labor. Eight patients (7.5%) required a posterior instrumented fusion. There were five cases (4.6%) of postoperative facet arthrosis, three cases (2.8%) of subsidence, three cases (2.8%) of adjacent level disease, and two cases (1.9%) of core subluxation.
Ross and colleagues61 found poorer quality long-term results using the CHARITÉ device. Kaplan-Meier analysis was performed on 226 CHARITÉ implants. Radiologic failure was defined as a broken wire, subsidence, or lack of movement. At 8 years, there was a 90% survival rate. This rate declined dramatically to 35% at 13 years. At final follow-up, ODI improved by 14 points on average, 1 point below the benchmark for clinical improvement. At final follow-up, 69% of patients believed that they were better or much better than preoperatively; 20% believed that they were worse than preoperatively.
Cunningham and colleagues62 showed that the CHARITÉ disc is effective in maintaining lumbar motion. They reviewed radiographs from the initial IDE study and assessed motion at the disc arthroplasty as a percentage of the total lumbar spine motion. These numbers were compared with cadaveric normal discs. At 2 years, the disc replacement maintained normal segmental lumbar motion and preserved the overall lumbar motion. Putzier and colleagues63 reviewed 63 CHARITÉ discs at 17-year follow-up. At 17 years, 60% of the implanted discs had spontaneously ankylosed, and only 17% were deemed functional. In the patients in whom the disc replacement was still functional, there was no evidence of adjacent segment degeneration, suggesting that a functional implant is effective in preventing adjacent segment degeneration. Clinically, the patients with spontaneous ankylosis did better than patients with functional implants. One suggested cause of the clinical failure is hypermobility leading to early facet joint degeneration at the arthroplasty level. Hypermobility from removal of the anterior longitudinal ligament has been shown in vitro.64 In contrast, Huang and colleagues65 assessed 51 TDRs at 8.5 years. Range of motion greater than 5 degrees corresponded to improved clinical outcome.
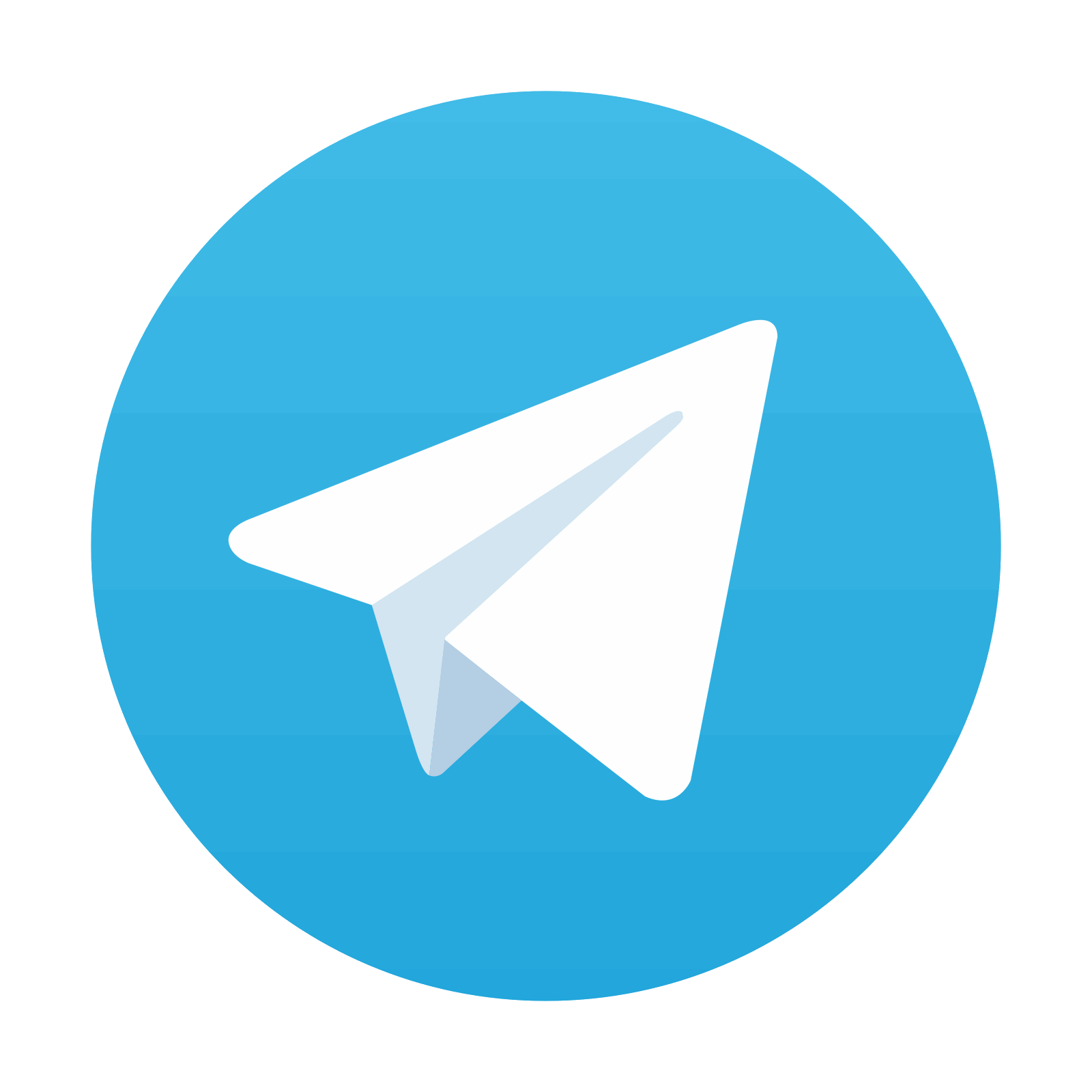
Stay updated, free articles. Join our Telegram channel

Full access? Get Clinical Tree
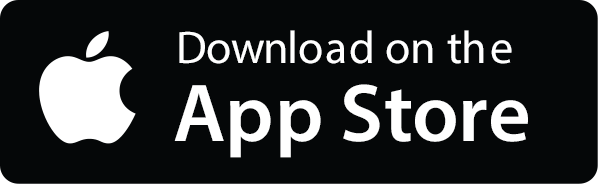
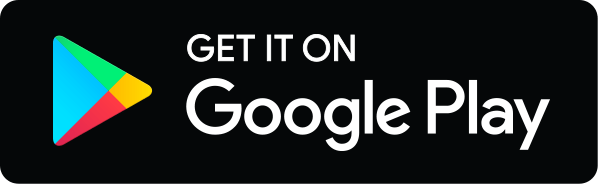