5
Instability and Laxity
One of the most common shoulder conditions is instability of the glenohumeral joint. Shoulder instability continues to be a significant problem for athletic and active individuals, but it can be seen in any age group due to trauma. The concepts of instability continue to evolve, and the physical examination changes with these concepts. In clinical practice, however, shoulder instability presents one of the most difficult diagnostic areas as a result of a variety of factors. First, our knowledge of the anatomy and the biomechanics of shoulder function as it relates to stability continues to increase and change. Second, the examinations for instability are good for diagnosing some types of instability but not others. For example, the anterior apprehension test for anterior shoulder instability is fairly accurate for the patient with traumatic anterior instability, but its usefulness is less clear in the throwing athlete who does not have true subluxations or dislocations. The overhead athlete who has pain in the shoulder and who may have some form of shoulder instability continues to be a diagnostic dilemma.
This chapter summarizes the existing examination findings for instability and interprets their meaning, clarifies the difference between laxity and instability, and discusses the examination of the shoulder in light of evolving concepts of instability.
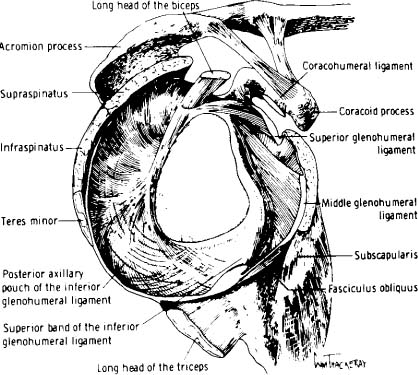
FIGURE 5-1 Ligament anatomy of the shoulder when viewed from the side (sagittal view). (Adapted with permission from Turkel SJ, Panio MW, Marshall JL, et al. Stabilizing mechanisms preventing anterior dislocation of the glenohumeral joint. 1981;63:1209.)
History of the Concepts of Instability
Instability at one time was a seemingly straightforward concept for the clinician. If the humeral head came out of the socket and stayed there, then the patient was thought to have a shoulder dislocation. If the humeral head went part of the way out but then went back into the joint, it was considered a subluxation. Over the past few years the normal anatomy has become better appreciated, and the complexity of shoulder instability has become apparent (Figs. 5-1, 5-2, 5-3).
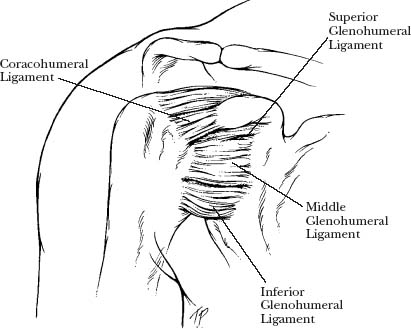
FIGURE 5-2 Ligament anatomy of the shoulder from the front (coronal view).
FIGURE 5-3 There are numerous variations of the superior labrum and middle glenohumeral ligaments. (Adapted with permission from Rao AG, KT, Chronopoulos E, McFarland EG. Anatomical variants in the anteroposterior aspect of the glenoid labrum: a statistical analysis of seventy-three cases. J Bone Joint Surg Am 2003;85(4):653–659.)
Initially, it was unclear which structures in the shoulder were damaged when the shoulder became unstable from traumatic causes. Perthes and Bankart1,2 both defined the pathology as a disruption of the labrum attachments to the glenoid, and subsequent studies demonstrated that there often is some form of capsular failure associated with labrum detachments (Fig. 5-4).
Rowe and Zarins3 in 1981 widened the concept of instability when they described the “dead arm” syndrome. They evaluated 60 shoulders where the patients complained of sudden, sharp, and paralyzing pain with the arm in an abducted and externally rotated position. This pain and heaviness of the arm could be caused by any activity in which the arm was used over shoulder level, including overhead work, tennis, swimming, and baseball. After the episode of pain the arm could be weak or “dead” for hours or days. Some of Rowe and Zarins’ patients had experienced a blow with the arm in abduction and external rotation, such as that seen in football and basketball players. In their cohort of patients, 26 patients with 27 shoulders injured could recall a sensation that the shoulder had distinctly come out of the socket. The other 32 patients with 33 shoulders injured did not feel a subluxation and complained only of the symptoms mentioned above. At the time of surgery, 32 shoulders (64%) had Bankart lesions, and the remainder had only capsular laxity.
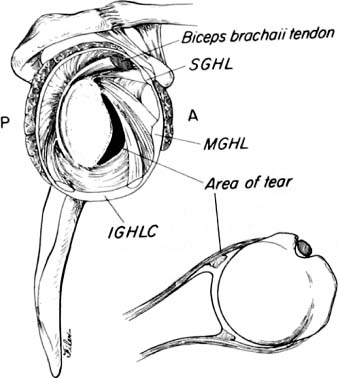
FIGURE 5-4 A Bankart lesion is detachment of the anteroinferior labrum from the glenoid rim. (Adapted with permission from Warner JP, Caborn DNM. Overview of shoulder instability. Crit Rev Phys Rehabil Med 1992;4:145–198.)
Consequently, Rowe and Zarins3 can be given credit for the concept of occult instability, where the patient did not feel a subluxation or dislocation of the humeral head out of the socket, but whose symptoms were due to some form of instability of the shoulder. These patients were unaware that they were actually having subluxations or near subluxations of the joint.
Rowe and Zarins were the first to suggest that the treatment of this condition was to perform only a capsular shift in cases where there was no Bankart lesion. Interestingly, 20 (54%) of their patients were felt to have a large rotator cuff interval, which they repaired with sutures. Of the patients who had their dominant arm operated upon, 64% were able to return to overhead work or sports with no limitation. Of the eight patients whose shoulders were judged to be damaged from forceful baseball pitching, four (50%) returned to their previous level of throwing, and four did not.
This concept was further expanded and popularized in the 1980s by Dr. Frank Jobe and colleagues,4 who postulated that the etiology of pain in overhead athletes was rotator cuff tendinitis due to instability. Whereas the throwing or overhead athlete felt only pain or a dead arm, the symptoms were produced by repetitive injury to the anterior restraining structures of the shoulder, specifically the anteroinferior glenohumeral ligament. Jobe et al theorized that the shoulder of throwing athletes and those athletes involved in overhead sports sustained significant stress to the ligaments when the arm was in an abducted and externally rotated position. This repetitive microtrauma to the ligaments resulted in their elongation. As the ligaments stretched, the rotator cuff impinged on the anterolateral acromion and produced pain (Fig. 5-5).
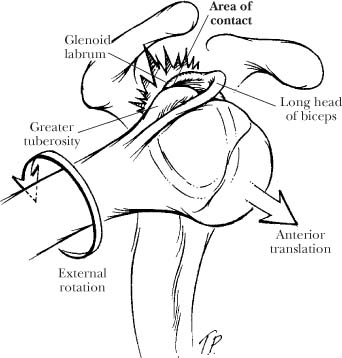
FIGURE 5-5 Hyperangulation of the humerus was postulated by Dr. Frank Jobe to lead to labrum and rotator cuff pathologies.
Upon examination of the patient, Jobe et al noticed that the shoulders felt loose and that placing the arm in a position of abduction and external rotation reproduced the pain. If nonoperative treatments failed, they recommended that an anterior capsular shift be performed to tighten the anteroinferior ligament complex. Jobe et al5 reported that this capsular shift procedure resulted in 68% of athletes capable of returning to throwing at their previous level for at least 1 year.
As a result of this work, shoulder pain in athletes was felt by many subsequent clinicians to be due to this form of occult instability, where the athlete did not feel overt subluxations or dislocation. In this syndrome, the only symptoms reported by patients included pain and the inability to perform their sport to a maximum level. The surgical treatment involves tightening the shoulder capsule that contains the ligaments, by performing a capsular shift either on the glenoid side5 or on the humeral side6,7 of the ligament attachments.
Other surgical techniques evolved to tighten the ligaments, including arthroscopic suturing and thermal capsular shrinkage techniques.8–10 The arthroscopic methods use sutures that are placed in the capsule arthroscopically to remove any redundancy in the capsule; this procedure is referred to as an arthroscopic capsular placation.11–13 In the thermal capsular shrinkage procedures, devices are used that generate heat to shrink the ligaments. This thermal injury to the tissue initiates a healing response that may tighten the ligaments. The thermal techniques were originally described as an initial treatment for capsular laxity, but due to high recurrence rates, they are increasingly recommended as an adjunct to other methods to tighten the capsule.14–16
The concepts of what causes pain in the shoulder of the overhead athlete continue to evolve. Walch et al17 in 1992 reported on internal impingement of the rotator cuff to the posterior and superior glenoid. When performing arthroscopy on the shoulders of 17 patients, Walch and colleagues could demonstrate this contact intra-articularly with the arm in abduction and external rotation. These concepts are discussed in more detail in Chapter 4. Because none of their patients seemed to be unstable upon examination, Walch et al suggested that this contact of the rotator cuff to the posterior and superior glenoid was physiological. For patients who failed nonoperative treatment, they recommended a humeral osteotomy, an operation they have since abandoned for this entity.18
With the advent of shoulder arthroscopy in the early 1980s, important discoveries were made about intra-articular pathology that was associated with shoulder pain and instability. In 1985, Andrews et al19 reported on superior labrum lesions in overhead athletes; they found that the stress of throwing was somehow the etiology. Subsequently, Snyder et al20 described four variations of superior labrum anterior to posterior (SLAP) lesions, and it became appreciated that these superior labrum detachments were found often in the overhead athlete.
F. Jobe et al4 noted that overhead athletes at the time of arthroscopy had SLAP lesions, partial rotator cuff tears, posterior humeral head chondromalacia, and rarely Bankart lesions. They later noticed that internal impingement as described by Walch et al17 could be seen arthroscopically in the shoulders of throwing athletes with pain. Jobe et al suggested that this contact of the rotator cuff to the posterior and superior labrum caused partial thickness cuff tears, labrum tears, or both (Fig. 5-6). These concepts are discussed in more detail in Chapter 6.
Pathology seen in the shoulder of the overhead athlete may include partial rotator cuff tears, SLAP lesions, and chondromalacia on the humeral head.
Another theory of why throwing athletes have pain in the shoulder is that they have a form of superior instability. Morgan et al21 and Burkhart et al22 suggested that the superior labrum attachments are gradually stretched by the overhead motion, and eventually a SLAP lesion results. Morgan et al divided type II SLAP lesions into three types based on their morphology, and they suggested that each of the three types had a different mechanism and pain pattern.
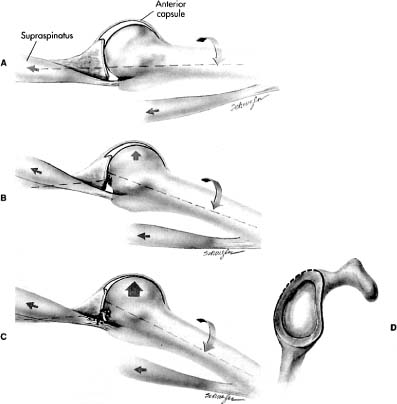
FIGURE 5-6 Internal impingement of the greater tuberosity and the rotator cuff to the posterior and superior glenoid has been postulated to cause labrum and rotator cuff pathologies. (Adapted with permission from Voluntary glenohumeral instability. In: Jobe FW, ed. Operative Techniques in Upper Extremity Sports Injuries. Baltimore: Mosby; 1996: 285–294.)
Furthermore, Morgan et al found that the SLAP lesion created a superior instability pattern that caused the athlete’s pain, and they reported that fixing the SLAP lesion could return the athlete to throwing in a large percentage of cases. At the time of surgery after the SLAP lesion had been repaired, if the shoulder was judged to still be loose in an anterior and inferior direction, they recommended tightening the shoulder with a capsular shift either arthroscopically with sutures or with thermal techniques. Using this approach, they reported that 100% of overhead athletes who had a SLAP repair with or without a capsular procedure returned to throwing. Of these 53 throwing athletes, 84% had returned to throwing with no limitations, and the remaining 16% reported a mild decreased velocity and control.22
Another philosophy regarding the reasons for shoulder pain in the overhead athlete has been developed by Nobuhara.23 With the publication in 2001 of his textbook on the shoulder, his concept of shoulder pain in overhead athletes has become accessible outside Japan.
Nobuhara contends that there are both functional and organic reasons for a throwing athlete to develop shoulder pain. However, he says that in the overhead athlete who presents with shoulder pain, the “concepts of subluxation, instability and loosening, or the terminology such as instability, translation, laxity and abnormal motion are confusing, and the addition of new information adds to the confusion.”
Nobuhara postulates that repetitive stress to the shoulder with throwing results in an injury to the rotator cuff interval in the anterior shoulder. This lesion consists of too much laxity in the rotator cuff interval, which changes the shoulder kinematics. With repetitive throwing, this rotator cuff interval lesion can result in anterior shoulder pain and a sense in the athlete that the shoulder is “unstable.” According to Nobuhara, when the interval becomes affected, the motion of the humeral head on the glenoid becomes altered so that with elevation there is anterior and inferior shoulder looseness with the arm in internal rotation.
Nobuhara also has noted the frequency of posterior shoulder pain in overhead athletes and concludes that the initial cause of the pain is fatigue of the rotator cuff muscles.23 He notes that the posterior capsule and infraspinatus tendon can become partially torn over time; he refers to this as an infraspinatus lesion. This lesion is typically an incomplete avulsion of portions of the infraspinatus tendon.
In Nobuhara’s cohort of 823 overhead athletes who presented to his clinic for evaluation, 388 (47%) had a rotator cuff interval lesion, 92 (11%) had an infraspinatus lesion, and 343 had both. When an athlete had both a rotator cuff lesion and an infraspinatus lesion, Nobuhara referred to this as anteroposterior instability in the throwing athlete, or an APIT lesion. A majority of the athletes could be treated effectively with rehabilitation, cortisone injection into the cuff interval, or distension of the subscapularis bursa with arthrography. Only 5.5% of the athletes required surgery, which in Nobuhara’s hands consisted of an open repair of the rotator cuff interval, resection of the coracoacromial ligament, and open repair of the infraspinatus tendon lesion. In this group of throwing athletes who required surgery, only two (0.2%) did not return to their previous level of throwing. Nobuhara recognized that treatment of the throwing athlete needed contributions from the clinician, the trainer, the coach, and the athlete.
Why “Occult” Instability is a Challenge
The concept of occult instability challenges the clinician when performing an examination of the shoulder of a patient who has pain with activity or who is overtly unstable. There are several reasons why the concept of “covert” or hidden instability confuses the interpretation of the examinations of the patient with “overt” instability. First, many studies have used laxity testing of the shoulder to define instability. Laxity testing is not easy to perform, and the definition of what is normal and abnormal is controversial. This is particularly troublesome when attempting to determine if someone is unstable in more than one direction, because the use of laxity testing tends to overestimate the number of patients who are unstable.24
Second, it is generally not desirable to demonstrate instability upon examination in the office because it is uncomfortable for most patients. As a result, the diagnosis often must be made based on patient history and radiographic studies. Third, there are many signs for instability upon physical examination that have never been adequately assessed using statistical methods. These tests claim to be capable of diagnosing occult instability based on the reproduction of pain, but most have not been validated for either increased laxity or overt instability.
Fourth, the concept of occult instability is based on the premise that pain in an overhead athlete indicates that the ligamentous restraints have failed, but in those patients there is currently no objective way to measure that instability. The clinician is left treating a symptom complex that has few signs upon physical examination, radiographic studies that are inconclusive, and no method to measure the results of treatment except the absence of symptoms. Finally, the results of surgical procedures for occult instability often seem to be less predictable than surgeons and physicians would like (Table 5-1).
Although excellent results have been reported for the treatment of pain and instability in overhead athletes, it often seems unpredictable as to who will get better and who will not with treatment. There are few objective measures that can be used to make an accurate diagnosis or to follow the results of surgery, and the treating physician is left to rely on symptoms experienced by the patient.
The exact etiology of shoulder pain in the overhead athlete is unknown, and because multiple theories exist as to the cause, the shoulder examination remains uncertain.
These issues demonstrate that the exact etiology of the pain in the overhead athlete is not definitely known. Is the pain due to the partial rotator cuff tear? What about athletes with no cuff tear? Do SLAP lesions produce pain, or do they cause pain by allowing increased tension in the rotator cuff? Does the rotator cuff interval fail and create a pseudolaxity? Does the inferior glenohumeral ligament complex fail first and produce SLAP lesions or the reverse? If the ligaments have failed, how do we measure it?
This chapter will assist the practitioner who evaluates patients with either overt or covert instability of the shoulder by demonstrating where the evidence is good for the accuracy of the examination and where it is not. The goal is to help the practitioner know when the examination is reliable and when it is not. Although the impulse is to want a test that will answer all of these questions, the reality is that it currently does not exist. As a result, the practitioner must put all the parts of the puzzle together to make a diagnosis and form a plan of treatment. Some forms of shoulder instability are easier to diagnose than others, and further study will help us differentiate them.
Laxity versus Instability
One of the most important concepts to be understood for any joint is distinguishing between laxity and instability. All joints are constrained in certain directions by how the bones interdigitate and by the ligaments that attach bone to bone. If there were no ligaments, two bones next to each other would have six degrees of freedom to move upon each other; they could translate three directions, they could distract, and they could rotate clockwise or counterclockwise (Fig. 5-7).
The amount two bones can slide or rotate upon each other depends on the position of the ligaments between the bones, their architecture, and their length. This amount of movement is the laxity of the joint and is the normal motion that is necessary for its function. Upon examination the laxity can be tested by moving the bones in relationship to each other, and the amount of displacement can be estimated and in some cases measured. For example, the anteroposterior translation of the knee can be fairly accurately measured with an arthrometer, such as the KT-1000 (MedMetric Corp, San Diego, Calif). Increased laxity as recorded on a KT-1000 can mean that the anterior cruciate ligament is torn if the laxity is increased a certain amount over the other side. Unfortunately, there is currently no KT-1000 for the shoulder, although investigators are developing this type of device.25
Biomechanics of Shoulder Stability
There are excellent reviews of the factors that provide stability to the shoulder joint.26–31 The factors that contribute to shoulder stability include the bony anatomy of the glenoid and proximal humerus, the negative intra-articular pressure, the ligaments, the rotator cuff tendons, and the compressive force of the muscles. The labrum serves to deepen the socket and provides stability as a “chock block” to humeral head translation (Fig. 5-8).27 The labrum also provides stability by serving as the anchor of the glenohumeral ligaments to the glenoid rim.
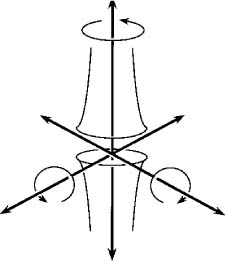
FIGURE 5-7 In any joint there are six degrees of freedom that are restrained by ligaments or by the constraints of the bones making up the articulation.
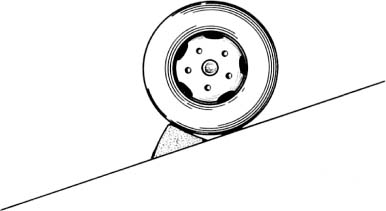
FIGURE 5-8 The glenoid labrum functions as a “chock block” to prevent the humeral head from sliding off the socket. (Adapted with permission from Warner JJP. The gross anatomy of the joint surfaces, ligaments, labrum and capusule. In: Matsen FA, Fu FH, Hawkins RJ, eds. The Shoulder: A Balance of Mobility and Stability. Rosemont, IL: American Academy of Orthopaedic Surgeons; 1993:7–27.)
The bony configurations of the proximal humerus and the glenoid contribute to stability of the shoulder joint. The humeral head is much larger than the glenoid, and the typical analogy is that the shoulder joint is like a beach ball on a dinner plate or a golf ball on a tee. Because the humeral head diameter is nearly twice that of the glenoid in the transverse plane, the humeral head requires other mechanisms to keep the head within the confines of the glenoid. The humeral head is typically retroverted, whereas the glenoid is in neutral or slightly retroverted.32–34 There is a wide variation of the normal range of humeral retroversion reported in the literature. Likewise, there is a wide range of normal for glenoid retroversion.35,36
Clinically, glenoid retroversion or humeral head retroversion does not seem to contribute to instability except in a few circumstances. In patients with glenoid dysplasia, where there is significant loss of posterior glenoid bone, the patients may develop arthritic changes and occasionally posterior instability of the shoulder. Patients who have joint arthroplasties, where the humeral head is placed in an abnormal extreme of anteversion or retroversion, may develop instability. However, we agree with Pagnani et al,27 who comment that bony alignment alone is rarely the cause of instability in most patients.
The integrity of the glenoid surface has been shown to contribute to instability, particularly if the bone of the glenoid rim is fractured or eroded away. In patients with traumatic instability, the bony rim can fracture away and is known as a “bony Bankart” lesion. Biomechanical studies have shown that if the amount of bone loss approaches 20% of the glenoid length, the shoulder can become unstable. Anteroinferior osseous defects of the glenoid were simulated by Itoi et al 37,38 by removing 12.5%, 25%, 37.5%, and 50% of the glenoid length (Fig. 5-9). Notable defects in stability during abduction while in external rotation and in internal rotation were evident at even the lowest amount of bone resection.37,38 Loss of bone from the anterior and inferior glenoid has been proposed as a major factor in the failure of some arthroscopic anterior stabilizations.39
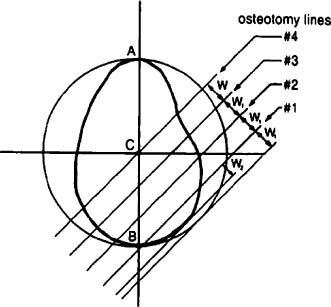
FIGURE 5-9 Osseous defects of the glenoid may contribute to shoulder instability, especially once they reach 20% of the surface. (Adapted with permission from Itoi E, et al. The effect of a glenoid defect on anteroinferior stability of the shoulder after Bankart repair: a cadaveric study. J Bone Joint Surg Am 2000;82(1):35–46.)
The shoulder joint, like most joints, is a closed system that has negative intra-articular pressure. This is believed to be due to the adhesive nature of the synovial fluid, which tends to hold the bones together. This factor typically is not clinically important because a vented shoulder joint is, fortunately, rare in shoulder injuries. However, this factor is important in biomechanical studies where the joint is vented to perform the study. Venting a shoulder joint in a biomechanical study drastically reduces the amount of force necessary to translate the joint.40
Another factor that provides stability to the shoulder joint is called the concavity compression, which is compression of the humeral head into the glenoid. This is provided in part by the bony geometry, by the labrum, and by the muscles that will compress the humeral head into the socket (Figs. 5-10, 5-11). When the arm is moved, the concavity compression serves to keep the humeral head relatively centered in the socket. Because the shoulder does not demonstrate perfect ball-and-socket mechanics when the arm is moved, the humeral head will undergo some translation on the socket with elevation or rotation of the arm. These translations of the center of the humeral head upon the glenoid are said to be coupled to humeral or arm motion in elevation or rotation. These motions are controlled by the interaction of the labrum, ligaments, and rotator cuff.
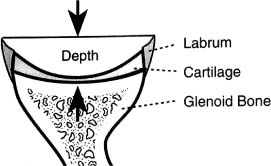
FIGURE 5-10 Concavity compression may be due in part to the bone configuration. (Adapted with permission from Matsen FI, Lippitt S, Sidles J, Harryman DI. Practical Evaluation and Management of the Shoulder. Philadelphia: WB Saunders; 1994.)
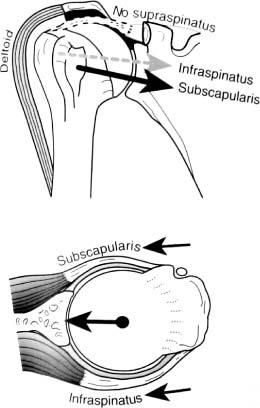
FIGURE 5-11 Concavity compression also may be due to the musculature that compresses the humeral head into the socket. (Adapted with permission from Matsen FI, Lippitt S, Sidles J, Harryman DI. Practical Evaluation and Management of the Shoulder. Philadelphia: WB Saunders; 1994.)
The exact contribution of the labrum to the concavity compression depends on the arm position and the amount of compressive load.41 According to Matsen et al,28 the labrum effectively deepens the socket, and it creates a bumper to resist translation (Fig. 5-8). The labrum doubles the anteroposterior depth of the glenoid.42 Matsen et al28 calculated that this mechanism provides up to 60% of the force resisting translation of the humerus on the glenoid. Lippitt et al43 demonstrated that excision of the glenoid labrum reduces the stability of the glenohumeral joint through the concavity compression mechanism by ~20%. Thus, in a shoulder with a labral lesion, the concavity compression mechanism may be compromised, so that there are increased translations of the humeral head on the socket with shoulder motion.
In the normal shoulder, Matsen et al28 suggested that the majority of compression forces are provided by the rotator cuff muscles (subscapularis, supraspinatus, infraspinatus, teres minor), and this compression of the humeral head into the glenoid provides stability to the joint. Though controversial, some have suggested that the biceps tendon may provide some stability to the glenohumeral joint with the arm in certain positions. As a result, it has been suggested that patients with instability of the shoulder through the concavity compression mechanism can increase the security of the glenohumeral joints by strengthening the rotator cuff muscles. Clinically, the concavity compression mechanism can be compromised in patients with shoulder muscle weakness, torn rotator cuff tendons, labral tears, or glenoid defects.28
The next major restraints to shoulder motion are the capsular ligaments (Table 5-2). Biomechanical studies have indicated that the major restraint to anterior translation of the humeral head upon the glenoid with the arm at the side is the anterior capsule.26 With the arm in an abducted and externally rotated position, the anterior band of the inferior glenohumeral ligament is the major restraint to instability of the joint. The second major restraint to translation in this position is the anterior glenoid labrum. Once the anterior structures fail, the next structures to undergo deformation include the posterior capsule. This injury to the opposite side of the primary direction of instability is referred to as the “circle concept” of shoulder stability (Fig. 5-12).44
The major restraint to posterior excursion is the posterior capsule when the arm is at the side or when the arm is in front of the body. In one biomechanical study, for a posterior dislocation to occur with the arm in this position, the anterosuperior capsule also had to be incised from the 12 to the 3 o’clock position.45–48 Furthermore, Harryman et al49 demonstrated with the arm in an adducted position that the rotator cuff interval (anterosuperior capsule) was the primary restraint of the inferior and posterior translation of the humeral head. With the arm in a position of adduction, flexion, and internal rotation, the glenohumeral joint did not dislocate posteriorly. In the adducted arm in the external rotation position, the middle glenohumeral ligament has the primary role in preventing inferior subluxation of the humeral head.50
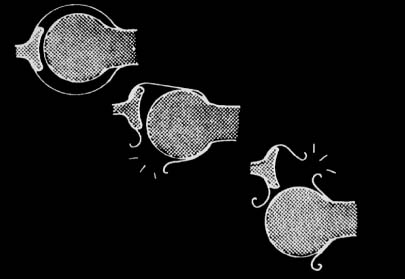
FIGURE 5-12 The circle concept of shoulder instability suggests that for severe excursion of the humeral head to occur, the ligaments opposite the side of the direction of translation will also eventually fail. (Adapted with permission from Pagnini MJ, Warren RF. Stabilizer of the glenohumeral joint. J Shoulder Elbow Surg 1994;3:173–190.)
The major restraints to inferior translation have been found to be dependent on shoulder position. Warner et al50 demonstrated that the primary capsuloligamentous constraint to inferior humeral subluxation in the abducted position is the posterior band of the inferior glenohumeral ligament. At 45 degrees of abduction, the anterior band of the inferior glenohumeral ligaments becomes the primary restraint to inferior translation in either a neutral or an internally rotated position; when the arm is in an externally rotated position, the posterior band is the primary restraint of the inferior glenohumeral ligament. At 90 degrees of abduction, the posterior band of the glenohumeral ligament is the primary restraint to inferior translation in external rotation, and the anterior band is the primary restraint to inferior translation in internal rotation.50
The major structures that prevent superior subluxation and instability of the humeral head depend on which direction is being considered. Some authors suggest that there is a difference between straight superior subluxation and anterosuperior subluxation.21,51–53 The major restraint to superior migration of the humeral head is the supraspinatus tendon. When the supraspinatus tendon is torn, the next restraints to superior migration become the acromion and the acromioclavicular (AC) joint. Some patients with massive rotator cuff tears can have migration of the humeral head superiorly to the point of causing erosion of the AC joint.54–56
The major restraints to anterior and superior subluxation include the coracoacromial ligament, the rotator cuff interval tissue, and the anterior edge of the acromion.57–59 Although the coracoacromial ligament has been implicated as a culprit in the etiology of rotator cuff disease, there is increasing evidence that it serves an important function as a restraint to the humeral head as the arm is elevated. This function is increased when the rotator cuff tendons are torn and no longer keep the humeral head centered in the glenoid. This anterior and superior subluxation of the humeral head has become recognized as a common cause of disability in patients with massive rotator cuff tears, after failed rotator cuff repairs, and after hemiarthroplasty for proximal humerus fractures.
The “Zero” or “Loose Packed” Position
The resting position of the shoulder has been described as the position in which the shoulder capsule is the most lax. It is supposed that this position would be where the greatest translations of the shoulder can occur due to small concavity-compressive forces.60 This has also been called the “loose packed” position. This position has been recommended for assessing joint laxity and for evaluating restrictions that may cause pain.60,61
This position should not be confused with the position of the arm at rest when the arm is at the side. Based on observation and experience, the zero or loose packed position has been reported to be in the plane of the scapula and around 55 to 70 degrees of abduction of the arm (i.e., the humerus in relation to the thorax)60,61 (Fig. 5-13). There have been several studies that have evaluated shoulder joint laxity based on arm position. Debski et al,62 using robotic manipulators in a cadaveric shoulder model, found that anteroposterior translation of the shoulder was greatest with the arm in neutral rotation and elevated between 30 and 60 degrees in relation to the thorax.
Hsu et al60 studied translations in a cadaver model using an instrumented testing machine. They found that the position for the greatest excursion of the humerus on the glenoid was at 40 degrees of glenohumeral abduction, which corresponds to a position of around 55 to 70 degrees of arm abduction if one were to adjust the model for scapulothoracic motion. They found that there was no one position for all specimens where there is the most laxity, but rather the loosest position overall for all specimens seemed to be around 45% of maximum arm abduction. Like other researchers they found that the joint became more constrained (i.e., had less laxity) above or below that level.
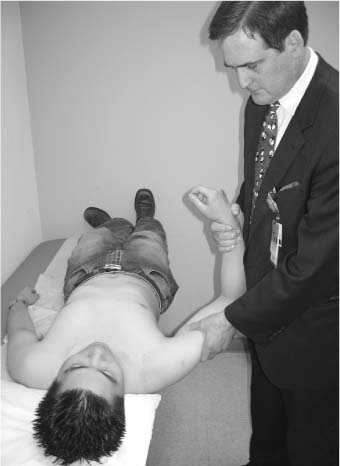
FIGURE 5-13 The position of the area where the ligaments are loosest, also known as the “loose-packed position,” is with the arm abducted 50 to 60 degrees with neutral rotation as shown here.
The importance of these findings is that the position where the arm is tested for laxity testing will determine how much translation is available upon examination. There is increasing evidence that the constraints to movement are dependent upon arm position and the amount of compressive force applied to the joint. These factors explain why two physicians may obtain different results when examining the same shoulder. If the movements are not tested with the arm in nearly the same position, it is likely that different results will be obtained.
The position of the arm when testing for laxity is important when comparing results by multiple examiners or when performing serial examinations.
Laxity Testing
The shoulder is one of the most mobile joints in the body, but it is important to be able to evaluate shoulder laxity because it can help when making the diagnosis of instability. When testing ligaments in the body to determine if they are torn, it is important to measure not only the laxity of the joint (how much excursion there is between the bones) but also the firmness of the end point of the test. If a ligament is intact, there typically is a “firm” end point with examinations that test that ligament; if it is torn, then the end point is “soft” because the ligament does not abruptly stop motion.
Unfortunately, in the shoulder it is difficult to evaluate the end point in any one direction because several parts tend to want to move at once during the examination. When trying to examine the shoulder for laxity, the scapula can move on the thorax, and the humerus can rotate at the glenohumeral joint. As a result, one cannot reliably use the presence or absence of an end point as a criterion for ligament disruption in the shoulder.
The inherent laxity of the shoulder has been measured in laboratory and clinical studies. It is important to understand how the studies are performed, because this influences the amount of laxity that can be expected in the shoulder. In the laboratory studies, the bones of the shoulder typically are placed in jigs, which hold them in place, or electromagnetic sensors are attached that will closely monitor the movement of the bones. In studies where the bones are attached to jigs, the excursion of the bones must necessarily be constrained in some direction or other.
One thing to consider when evaluating these biomechanical studies of shoulder ligament function is the effect of venting the joint on the bone movements. The shoulder capsule creates a suction effect when it is closed, and when it is vented this suction effect is lost, and the bone excursion can increase significantly.40 Venting the joint may produce results that do not accurately reflect the normal function and motion of the shoulder joint.
Another important variable when evaluating these studies is the amount of force used to create excursion or translation of the joint. This is important because a large force will cause greater excursion, and a lesser force will produce less excursion. The amount of force used can influence the amount the bones move in both laboratory studies and in clinical studies involving measurement of laxity using surface markers or indwelling pins placed in the bones. This variability in force used to measure translation can be a factor in clinical practice because one examiner may push harder on the joint than another. This may result in one examiner measuring a large translation of the bones and another examiner measuring less translation in the same subject. The position of the bones at the time of testing in these studies is also important because it may not duplicate the clinical situation.
The results of these laxity studies are summarized in Table 5-3. These numbers are important to consider when determining what is normal and what is abnormal movement of the humeral head on the glenoid. The variation in results can be explained by the variability in the experimental methods of each study.
Several studies have examined shoulder laxity in cadavers. Sauers et al68 found that, using a 200-N force in a cadaver model, the humeral head translation on the glenoid was anteriorly 11.8 mm ± 5.7 mm, posteriorly 8.6 mm ± 4.8 mm, and inferiorly 20.2 ± 7.7 mm. Reis et al66 measured in cadavers shoulder joint translations using electromagnetic three-dimensional goniometers and compared the results with translations measured using skin sensors. They did not report the amount of force used during the translations. They found an average anterior translation of 9.8 mm, with a range of 3.7 to 14.7 mm, and an average posterior translation of 9.6 mm, with a range of 3.7 to 17.2 mm.
Clinical studies of the laxity of the shoulder joint in living human subjects (as opposed to cadaveric studies) have been performed by several researchers. Harryman et al64 studied shoulder laxity by placing pins into the humerus and scapula of volunteers. They then moved the bones of the subjects manually with the subjects in seated and in supine positions, but they did not quantitate the amount of force used to create the translations. It is noteworthy that there was a wide range of laxities in all three directions of translation.
Two studies have evaluated the relationship of translations obtained upon physical examination to true translations of the bones as determined by rigid pin fixation into the bones. Reis et al66 studied cadavers with sensors attached to pins placed into the glenoid and the scapula, and they compared the translations with the results using sensors placed on the skin of the same specimens (Table 5-4). They found the cutaneous sensors had a strong agreement with the bone sensors for anterior and posterior translations (interclass correlation coefficients of 0.81 and 0.86, respectively). They did not quantitate the amount of force used to create translations, and the translations were performed with the specimens upright and supine.
Sauers et al69 performed a similar study using an instrumented laxity device that applied a 200 N load to the proximal humerus of cadavers. The translations were measured with skin sensors and with electrogoniometric devices attached by pins inserted into the bones. Sauers et al found minimal differences between the skin sensor measures and those obtained by the electromagnetic sensors. Correlation coefficients were found to be good for laxity in all directions (anterior = .71, p < .001; posterior = .69, p < .001; inferior = .68, p < .001). Minimal differences were observed between the cutaneous and bone-pinned measurement techniques for laxity. The authors concluded that the cutaneously applied sensors are accurate for determining shoulder laxities and stiffness of the joint.
Other studies have been done in living subjects to measure shoulder translations in millimeters. Tibone et al67 used skin sensors to study the shoulder joint translations of 43 female athletes who were either soccer players or swimmers. The subjects were tested supine, and the arm was held in a device that supported it in 90 degrees of elevation and neutral rotation. The translations were performed manually by the examiners; there was no control of the force used to move the arm on the scapula. The mean age of the athletes was 18.8 years, and both dominant and nondominant arms were tested.
The results were reported as total anteroposterior translations and not as anterior or posterior translations (Table 5-5). Sauers et al found that total anteroposterior translations in the swimmers’ dominant shoulders (average 12.4 mm, range 8.9–15.9 mm) and nondominant shoulders (average 13.8 mm, range 8.8–17.4 mm) were statistically larger than in the dominant (average 9.6, range 5.8–15.1 mm) and nondominant (10.7 mm, range 5.2–16.7 mm) shoulders of the soccer players. The side-to-side difference for the swimmers was 0.1 to 5.3 mm and for the soccer players, 0.1 to 4.3 mm.
Sauers et al68 used an instrumented device with a force transducer to produce translations in the shoulders of seated volunteers. They concluded that the amount of translation depended on how much force was applied to the shoulder. They also found that posterior laxity was found greater than anterior laxity, and that a wide spectrum of laxity was found in the shoulders of asymptomatic volunteers.
Other studies attempted to measure the head translation using radiographic techniques (Table 5-6).70–74 These studies vary according to the position of the arm tested, the type of radiographs taken, how the landmarks on the bones were measured, and how much force was used in the translations. The methodology used for measuring the radiographs influenced the results, so there is not a consistent measurement between studies.
Quantitative Measures of Laxity from the Clinical Examination
In these biomechanical studies, laxity was measured accurately in millimeters, but the best way to measure laxity in the office or operating room remains controversial. There have been three different systems for measuring shoulder laxity in clinical practice.
Translation of the Humeral Head in Millimeters
The first system for measuring how much the humeral head moves on the socket is in millimeters; this system was recommended by Hawkins et al75 (Fig. 5-14). They recommended that the classification of humeral head translation be expressed in millimeters of translation over the glenoid face. In this schema, grade 0 equals no translation, grade I equals mild translation (<1 cm), grade II equals moderate translation (1–2 cm, or to the rim), and grade III equals severe translation (>2 cm, or over the rim).
Using millimeters to estimate shoulder laxity for anterior and posterior translations, to our knowledge, has never been validated as accurate. Similarly, no study has evaluated the inter- or intraobserver reproducibility of using millimeters as a measure for anterior or posterior laxity. As a result, we do not recommend this type of estimation of shoulder laxity in clinical practice.
The millimeter system has been used frequently for quantitating inferior translation of the humeral head with the sulcus sign. Commonly reported grading systems for inferior laxity using this sign include grade I (0.5–1 cm of translation), grade II (1–2.0 cm), and grade III (> 2.0 cm).76 However, there are some studies where the sulcus sign is graded as I (0.5–1.0 cm), II (1.0–1.5 cm), and III (> 1.5 cm).77,78 Hawkins et al75 recommended a classification of inferior translation with sulcus testing: grade 0 (no translation), grade I (mild translation, 0ߝ1 cm), grade II (moderate translation, 1–2 cm), and grade III (severe translation, > 2 cm).76,79,80
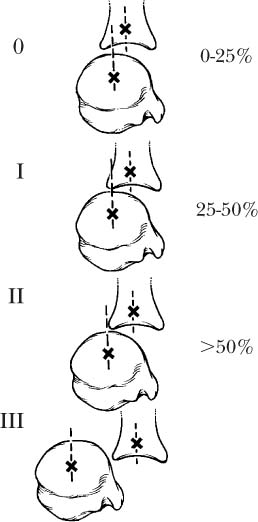
FIGURE 5-14 The Hawkins classification of humeral head translation suggests that the magnitude of translation can be reported by the examiner in millimeters.
None of these systems for measuring the sulcus sign has been validated using accurate methods of measuring translation, such as electrogoniometers with pins placed in bones. It should be noted that in Harryman et al’s study,64 the inferior excursion of the humeral head inferiorly was 11 mm ± 4 mm, with a range of 5 to 15 mm. They examined only normal male subjects between the ages of 25 and 45 years, so it is unclear what translations are possible in females or in subjects with instability. The only study to demonstrate inferior translations that approach 20 mm was conducted by Sauers et al69 upon cadavers. As a result, it is likely that most grade III sulcus signs that are measured in clinical practice are actually under 2 cm of translation.
There have been only two published studies that have evaluated the intraobserver reproducibility of the grading system of sulcus signs.78,81 In a study by Levy et al,81 four examiners evaluated 43 athletes for a sulcus sign, then repeated the test 3 months later. The examiners included physicians with different degrees of experience, including a resident, two orthopedic sports medicine fellows, and a sports medicine attending physician. The patients were examined supine. The researchers found that the intraexaminer reproducibility for a sulcus sign was 93% for the senior surgeon (kappa > 0.5), for the fellows 91% (kappa > 0.5), 74% (kappa = 0.5), and 86% for the resident (kappa > 0.5). They found that the interobserver agreement between the attending physician and the fellows was 43% and 39%, and 64% for the resident. When they combined grades 0 and 1, there was improvement of the reproducibility to 85%, but the kappa value remained low at > 0.5. Although the reproducibility appeared to be high within one examiner, the sulcus sign was not agreed upon by different examiners.
The sulcus sign is a measure of laxity and not instability, and it should not be graded but reported as positive or negative.
We performed a study78 of interobserver agreement for the sulcus sign using sports medicine fellows and an attending physician who examined 88 shoulders of patients who were under anesthesia. All the fellows had been in the fellowship for 6 months before participating in the study in order to gain experience and familiarity with the grading system. The examinations were performed blinded, so that one observer did not know the results of the other examiner. There was agreement on the degree of sulcus sign 70% (range 61–87%) of the time between the fellows and the attending surgeon (kappa 0.38, range 0.12–0.76). The most agreement between the fellows and the senior surgeon was when the sulcus was grade I (80% agreement) rather than grade II (65% agreement) or grade III (zero agreement). This study used the results of the attending physician as the gold standard because it was not possible to validate the exact amount of excursion in terms of millimeters. This study suggests that different examiners tend to disagree on inferior laxity as the amount of the laxity increases.
Only one study80 has evaluated the usefulness of the sulcus sign in patients with shoulder instability. Tzannes and Murrell80 reported on the sensitivity, specificity, and likelihood ratios (see appendix) of the sulcus sign for an unspecified group of patients with multidirectional shoulder instability (MDI). It should be noted that they did not define MDI in this study. They reported that a sulcus sign of under 1 cm had a sensitivity of 72% and a specificity of 85% for instability, but when the sulcus sign was over 2 cm, sensitivity went down to 28%, and specificity was 97%. These data demonstrated that using a strict criterion of 2 cm as a sign of inferior instability results in 72% of patients with MDI not being diagnosed with that condition. The authors did not validate the measures with radiographs or other techniques.
Translation of the Humeral Head in Percent Head Diameters
The second way to measure the amount of translation of the humeral head is to report the movement in terms of percent of the total head diameter.82,83 In this classification scheme, the humeral head is said to move to the rim (grade I), over the rim less than 50% of the diameter, or over the rim 50% of the head diameter. It has been suggested by some researchers that 25% is a subluxation and 50% a dislocation.84
There are several difficulties with this method of gauging translation of the humeral head. First, there has been no study that validates this method of measurement and that proves that the grades actually correspond to certain percentages of head diameter. Second, humeral head diameters vary widely, and the examiner cannot really estimate the head diameter exactly. Third, Harryman et al64 tried to validate the use of humeral head diameters as a measure of laxity. In their study of normal volunteers, they translated the shoulder and calculated the percent of humeral head translation by using normative data for humeral head diameters obtained from the literature. They found in their normal male subjects that the excursion anteriorly would have been only 35% of the humeral head and posteriorly only 35 to 39% of the humeral head. Inferior translation would have been 44% of the diameter of the humeral head on average. Harryman et al concluded that this form of measurement of translation was problematic and that the accuracy of this grading system using percent of humeral head diameters was questionable.
FIGURE 5-15 The Hawkins classification of instability is based upon what is felt by the examiner. (Adapted with permission from Matsen FI, Lippitt S, Sidles J, Harryman DI. Practical Evaluation and Management of the Shoulder. Philadelphia: WB Saunders; 1994.)
Translation of the Humeral Head Based on What is Felt
The third method of measuring translation of the humeral head is to describe what is felt by the examiner and not to rely on exact measures in millimeters or percentages. This classification was first described by Hawkins and Krisman85,86 and included four grades: 0 = normal translation, I = translation of the head to the rim, II = translation of the head over the rim, and III = locks out, where the humeral head stays out of the joint when the hands are removed (Fig. 5-15). This method of measuring translation was adopted by the American Shoulder and Elbow Society in 1994.87
A similar classification was suggested by Altcheck et al,88 but it included a comparison of the shoulder translation to the opposite shoulder. In their system, a grade I laxity indicated that there was increased translation compared with the other shoulder, but there was no subluxation or dislocation upon examination. Grades II and III laxity were the same as those reported by Hawkins and Krisman.85,86 This classification was encumbered by the need to accurately examine both shoulders, which is not always possible. This system also assumes that symmetry is normal, but subsequent studies have demonstrated that asymmetry of translation is frequently normal.77
We modified the Hawkins classification to discard the grade 0 designation because “normal” translation upon physical examination was not something that could be felt or quantitated by the observer. Upon examination the examiner either will feel the head go over the rim (grade II laxity) or will not (grade I) (Fig. 5-16). This modified Hawkins scale includes grade III as “locks out.”
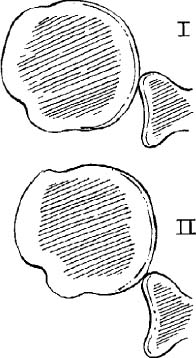
FIGURE 5-16 Studies have shown that combining translations 0 and 1 of the Hawkins classification increases the accuracy of the system. In a modified Hawkins scale, the examiner reports the translation as 1 (not over the rim), 2 (over the rim), or 3 (locks out). (Adapted with permission from McFarland EG, Campbell G, McDowell J. Posterior shoulder laxity in asymptomatic athletes. Am J Sports Med 1996;24(4):468–471.)
The validity of these classification systems has not been determined by biomechanical studies. None of the previously published studies using this measurement have demonstrated exactly how much translation is needed to subluxate the shoulder over the rim. Also, in some patients a click is felt when attempting to subluxate the shoulder, and it is sometimes difficult to know if that click is a subluxation or some other phenomenon, such as the labrum clicking. The intraobserver reproducibility of the Hawkins system, however, has been examined by McFarland et al24,78 and Levy et al.81 In the study by McFarland et al, one examiner evaluated 40 shoulders in 20 volunteers 4 weeks apart. The shoulder was evaluated using an anterior and posterior drawer as described by Gerber and Ganz.89 In this study we used the modified Hawkins scale, where there was no grade 0 but only grades I, II, and III. Twelve shoulders were eliminated when the subjects could not relax, so only 28 shoulders were included in the study. For anterior translation the reproducibility for one examiner was 100%, and for posterior translation it was 86%. It is possible that these numbers were so high because the examiner was an experienced shoulder surgeon with a consistent technique of making the measurement.
The second study to evaluate the intraobserver reproducibility of the Hawkins scale for anterior and posterior translations was performed by Levy et al81 on athletes at Duke University. The five examiners in this study included an attending orthopedic surgeon, two sports medicine fellows, and a resident who examined 43 athletes 2 months apart. They found that intraobserver reproducibility was only 46% (kappa < 0.5) for all examiners (range 44–50%). If grade 0 laxity was included with grade I laxity (modified Hawkins scale), then the intraobserver agreement increased to 73% (range 67–73%).
The interobserver reliability of this measure has been studied by the same groups. In the study by McFarland et al,24,78 a modified Hawkins scale was used, and the patients were all examined under anesthesia using an anterior and posterior drawer test as described by Gerber and Ganz.89 The four fellows agreed with the attending physician for anterior translations 69 to 89% of the time (kappa 0.41–0.73). For posterior translations, the fellows agreed with the attending physician 57 to 87% of the time (kappa 0.12–0.76). These kappa values are in the fair to poor range for interobserver agreement.
In their study, Levy et al81 found that overall interobserver agreement was 47% if the original rating scale of Hawkins and Bokor83 was utilized. For anterior translations, if grades 0 and 1 were equalized (a modified Hawkins scale), the overall interobserver agreement increased to 73%. Once the modified Hawkins scale was used, the fellows agreed with the attending physician 68 and 75% of the time for posterior translation, and the agreement between the attending physician and both fellows was 67% of all shoulders tested.
These studies suggest that this grading scale can be used to measure translations such that they can be communicated between observers, but these studies also suggest that comparing studies may still be difficult due to interobserver variability. It is our belief that this system has the most utility for clinical practice, but an instrumented device holds the most promise for the future.
A modified Hawkins scale is the most valid and reproducible method for reporting laxity of the shoulder in an anterior and posterior direction.
Examination Techniques for Measuring Anteroposterior Laxity
Anterior and Posterior Drawer
The anterior and posterior drawer signs for measuring laxity were described by Gerber and Ganz89 in 1984. This examination is performed with the patient supine and the examiner to the side. The subject must be relaxed, and it is recommended that the subject be placed so that the shoulder is not supported by the table. Gerber and Ganz recommended abducting the shoulder between 80 and 120 degrees.
The posterior drawer is performed by holding the patient’s wrist with one hand and placing the other hand over the patient’s shoulder so that the thumb is in the front and the fingers in the back (Fig. 5-17). Gerber and Ganz recommended holding the forearm with the elbow flexed, but we prefer to hold the arm at the wrist because the arm is easier to control (Fig. 5-18). The thumb should be placed directly over the humeral head and a posteriorly directed force applied on the humeral head. It is essential that the arm be forward flexed at the same time a posterior force is applied to allow the head to sublux posteriorly out the back of the shoulder. The fingers in the back can be used to palpate the head as it goes out the back. Pressure is then relieved from the thumb anteriorly so that there is no longer any force from the examiner keeping the shoulder subluxated out the back of the shoulder. It is then determined by the examiner if the head stays subluxated out the back of the shoulder joint with no force. If the humeral head stays subluxated out the back (i.e., “locked out”), then it is considered a grade III laxity. The head typically subluxes back into the joint on its own as the arm is extended, and the examiner can use the fingers posteriorly to feel the reduction (i.e., a grade II laxity).
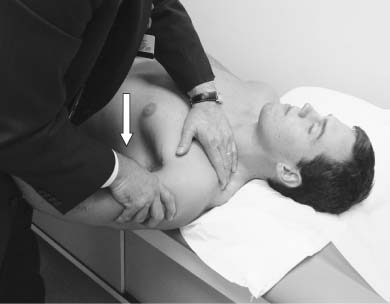
FIGURE 5-17 The posterior drawer test described by Gerber is performed with one hand stabilizing the scapula.
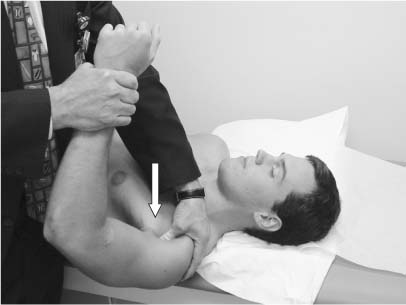
FIGURE 5-18 Posterior laxity testing of the shoulder can be performed without stabilizing the scapula.
We typically ask patients if the subluxation reproduces any of their symptoms. Crepitus with this maneuver is nonspecific and does not indicate either a labral tear or instability. It should be noted that in our studies of the posterior drawer that we perform the maneuver with the arm elevated ~45 to 60 degrees where the posterior capsule is the most lax.26 This position is also called the zero “unpacked” position because it is the point at which the joint has the most mobility90. This discrepancy in arm position between our studies and studies using Gerber and Ganz’s technique may explain the higher laxity grades reported in our studies compared with others.
More translation of the humerus on the scapula can be obtained with the arm abducted less than 90 degrees when performing laxity testing.
The anterior drawer is more difficult because the examiner has to control scapular rotation on the thorax. The arm is abducted 80 to 120 degrees, flexed 0 to 20 degrees, and held in 0 to 30 degrees of external rotation. Gerber and Ganz89 recommended that one hand stabilize the scapula, while the second hand holds the arm at the proximal humerus area (Fig. 5-19). The hand stabilizing the scapula holds the thumb on the coracoid and the fingers tightly on the scapular spine like a clamp. An anterior force is then directed on the humerus to create anterior translation.
We have modified the anterior drawer test to control rotation of the scapula (Fig. 5-20). The hands changed from the position used in the posterior drawer so that now one hand is on the wrist and one hand is on the proximal arm of the extremity. The arm is abducted ~40 degrees and slightly internally rotated, and a slight axial load is applied to prevent the scapula from rotating. This axial load also improves the ability to feel the humeral head subluxate over the glenoid rim. The examiner then applies an anterior force and rolls the humeral head onto the chest in one motion. It is important to try to translate the whole arm and not just the humeral head, because simply pushing the head anteriorly will tighten the anterior structures and prevent subluxation. Once the head is subluxed, pressure is relieved to see if the head remains out of the socket (grade III). If the humeral head subluxes back once pressure is released, then it is a grade II translation.
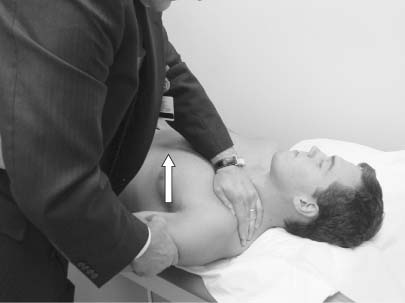
FIGURE 5-19 The anterior drawer test as described by Gerber is performed with one hand stabilizing the scapula.
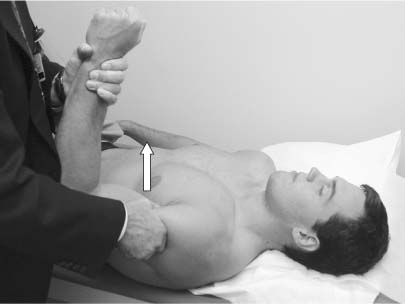
FIGURE 5-20 The anterior drawer test may obtain more excursion using the modified anterior drawer technique shown.
Applying a slight axial load to the humerus into the socket with an anterior drawer test makes it easier to feel the humeral head translate over the glenoid rim with laxity testing.
We are in agreement with Gerber and Ganz89 and Emery and Mullaji,91 who feel that shoulder laxity and translations can be appreciated better with the patient supine versus sitting. The subject is more apt to relax his or her muscles when supine than when sitting. Also, the position of the shoulder where there is the most laxity in the capsule is with the arm abducted around 45 degrees; this position is difficult to obtain with the patient sitting.
When performing the posterior drawer, if the patient has pain due to the hand placement on the proximal humerus, we recommend a modification whereby the proximal humerus is not grasped rigidly by the examiner. Instead, the arm can be held more distally, or the palm of the examining hand can be used to create a posterior force.
The validity of this grading system of translation has not been studied, but its reproducibility and reliability have been studied and are discussed above. Several studies using this test have demonstrated that the normal shoulder typically has a grade II laxity where the humeral head can be subluxated over the rim.24,78,92 In our study77 of high school and collegiate athletes who never had shoulder problems, 65% of the women and 50% of the men could be subluxated over the posterior rim with this test (i.e., were a type II laxity). This emphasized that it actually is more common in that age group to have a positive posterior drawer than it is to not have one. That is, a positive posterior drawer as we perform it suggests that the ability to subluxate the shoulder over the rim is normal.
It is normal to be able to subluxate the shoulder over the rim posteriorly in young athletic populations.
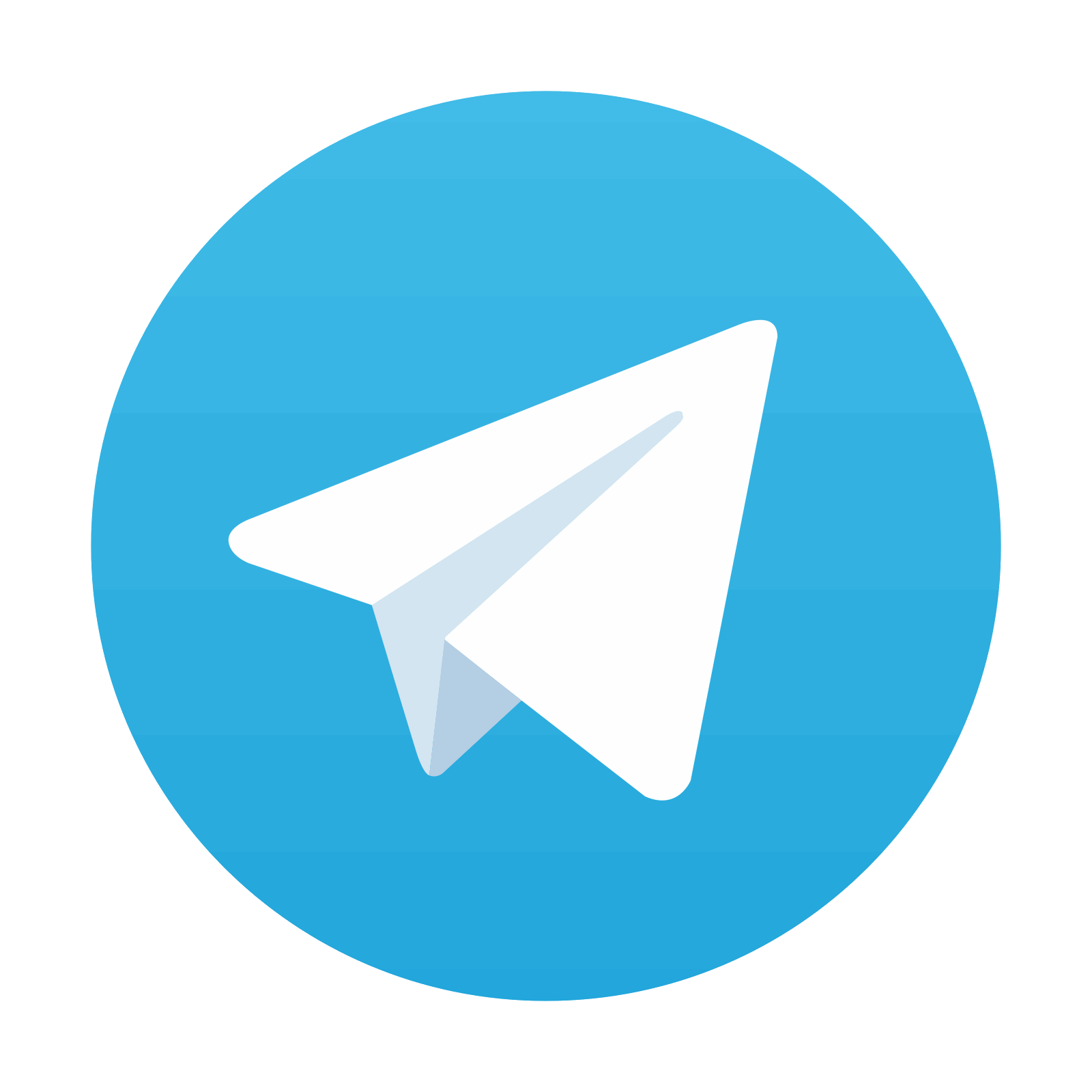
Stay updated, free articles. Join our Telegram channel

Full access? Get Clinical Tree
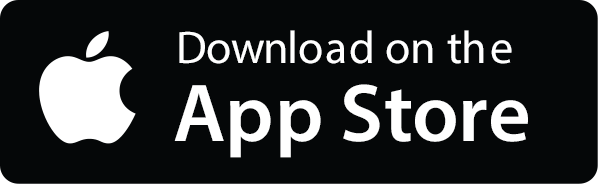
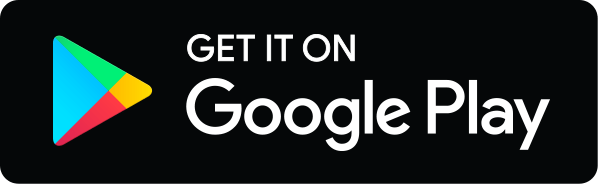