Highly Cross-Linked Polyethylene Bearings
J. Benjamin Jackson III., John L. Masonis and Thomas Fehring
Key Points
Background
The success of total hip arthroplasty (THA) has been well documented. Since its inception in the 1960s, the major obstacles to success in THA have been component fixation and articular bearing wear. With documented improvements in durable component fixation over the past four decades, wear remains the most troublesome issue in THA. Materials utilized for the acetabular articular bearing in THA have included Teflon, polyurethanes, metal alloys, ceramics, and polyethylenes. Over the past four decades, modifications to the structure and production of polyethylene have shown both positive and negative effects. Polyethylene remains the most widely utilized acetabular bearing surface in the world. Our knowledge of its structure and performance will strongly influence the future of total hip arthroplasty.
Polyethylene Manufacturing
Commercial production of ultra-high-molecular-weight polyethylene (UHMWPE) began in the early 1950s. The largest current manufacturer of UHMWPE resin is Ticona (Florence, Ky). All Ticona resins are labeled “GUR.” Current medical/orthopedic grade resins include GUR 1020, 1120, 1050, and 1150. The numeric label following GUR explains the details of the resin. The first digit (1) indicates that the resin is for orthopedic usage. The second digit represents the presence (1) or absence (0) of calcium stearate. The third digit indicates the molecular weight of the resin. The final digit is used for manufacturing corporate coding (1). Although other resins (1900 series produced by Hercules Powder, Wilmington, Del) have been utilized for orthopedic purposes and clinically studied, they are no longer in production. The remainder of this chapter will focus on the current GUR resins in clinical usage (GUR 1020 and GUR 1050).1
Polyethylene is composed of repeating ethylene monomers, each of which contains two carbon atoms. Polyethylene molecules contain a crystalline region and an amorphous region. The polyethylene chains can be described as low or high density based on their molecular weight and chain length. Polyethylene begins as a resin or powder and is fabricated into bulk form for orthopedic implant designs. Resins are developed into bulk material by direct compression molding or are machined from ram-extruded bars or large molded sheets.
The mechanical properties of any given polyethylene implant are affected by the resin, the molecular weight, and preparation of the material. The temperature, pressure, and cooling rate during the manufacture of polyethylene will influence its ultimate properties. Final component preparation is completed by direct compression molding (the polyethylene is melted and then is solidified in its desired shape) or by machining the material into its desired shape.
Sterilization
Sterilization of polyethylene has become an area of expanded interest. The three current methods of sterilization include ethylene oxide gas, gas plasma, and radiation. A combination of these techniques is utilized in the production of highly cross-linked polyethylene (HCLPE). Ethylene oxide sterilization is completed in an enclosed chamber because of the toxicity of the gas. Following diffusion of ethylene oxide into polyethylene and adequate time for clearance, the polyethylene is biologically sterile. The mechanical properties of polyethylene are unchanged following ethylene oxide sterilization. Gamma radiation sterilization of polyethylene has been utilized for many years. The radiation dosage has varied by manufacturer, but a minimum dose of 2.5 rads is required for adequate sterilization. Radiation sterilization does affect the mechanical properties of polyethylene. Although initially believed to be benign, radiation sterilization can have both positive and negative effects on polyethylene mechanical properties and wear characteristics.
Cross-Linking
Cross-linking of polyethylene involves the formation of a complex structure containing multiple polyethylene molecules. Initially, polyethylene cross-linking was an unintentional by-product of radiation sterilization. However, further research found that cross-linked polyethylene is a more mechanically stable material that is more resistant to wear. Manufacturers now use gamma or electron beam radiation to cross-link polyethylene. When polyethylene is irradiated, the polyethylene chains are broken and the molecules are able to re-form with increased cross-linking to adjacent polyethylene molecules, or they become free radicals that are capable of bonding to other molecules present in the material. If oxygen is present at the time of irradiation, the free radical polyethylene molecule can bond to oxygen, thus incorporating oxygen into the polymer structure. Oxygen free radical deposition has been shown to negatively affect the long-term mechanical properties of polyethylene through oxidation.2
Once oxidation was recognized as a major contributor to polyethylene wear, multiple strategies were employed to prevent oxygen bonding to free radicals. These strategies include gamma radiation in an oxygen-depleted environment (e.g., nitrogen vacuum) and the addition of other molecules (e.g., vitamin E) to “scavenge” free radicals and prevent oxygen bonding. Although cross-linking improves the wear characteristics of polyethylene, there is a downside to gamma radiation—it impairs the mechanical strength of polyethylene. The tensile strength and fatigue strength of polyethylene are inversely proportional to the amount of radiation applied to the material. Therefore, the dosage of gamma radiation and cross-linking must be regulated to maximize wear resistance while minimizing the risk of material fatigue failure (fracture). Table 71-1 summarizes the current production techniques used in first- and second-generation HCLPE.
Table 71-1
Highly Cross-Linked Polyethylene Production Methods
Clinical Results
Radiographic Analysis of Polyethylene Wear Rates
Numerous authors have attempted to systematically evaluate the wear rates of first- and second-generation HCLPE components. These studies have used radiostereometric analysis and computer-assisted edge detection techniques (Martell method)4 for analysis of wear, creep, and penetration rates. Both short- and, more recently, intermediate-term clinical studies have been reported with a maximum follow-up of 7.6 years (Table 71-2).
Table 71-2
Clinical Wear Studies of HCLPE
CPE, Conventional polyethylene; HCLPE, highly cross-linked polyethylene; RCT, randomized controlled trial; RSA, radiostereometric analysis.
Six studies have documented radiographic wear rates with more than 6 years of follow-up6–10 (Table 71-3). Three of these studies used retrospective comparison designs that evaluated first-generation HCLPE versus conventional polyethylene (CPE). McCalden and associates10 evaluated the wear rate of Longevity versus Trilogy liners using Martell methods and documented a 50% relative wear rate reduction in the HCLPE cohort at a mean of 6.8 years. Rajadhyaksha and colleagues12 evaluated the wear rate of Crossfire (HCLPE) versus Nitrogen Vac (CPE), using Martell methods, and documented a 74% relative wear reduction in the HCLPE cohort at a mean of 6.1 years. Rohr and coworkers34 evaluated the wear rate of Crossfire (HCLPE) versus Exeter (CPE) using radiostereometric analysis and documented a 74% relative wear reduction in the HCLPE cohort at a mean of 6 years.
Table 71-3
Six-Year Clinical Study Results
CPE, Conventional polyethylene; HCLPE, highly cross-linked polyethylene; RCT, randomized controlled trial; RSA, radiostereometric analysis.
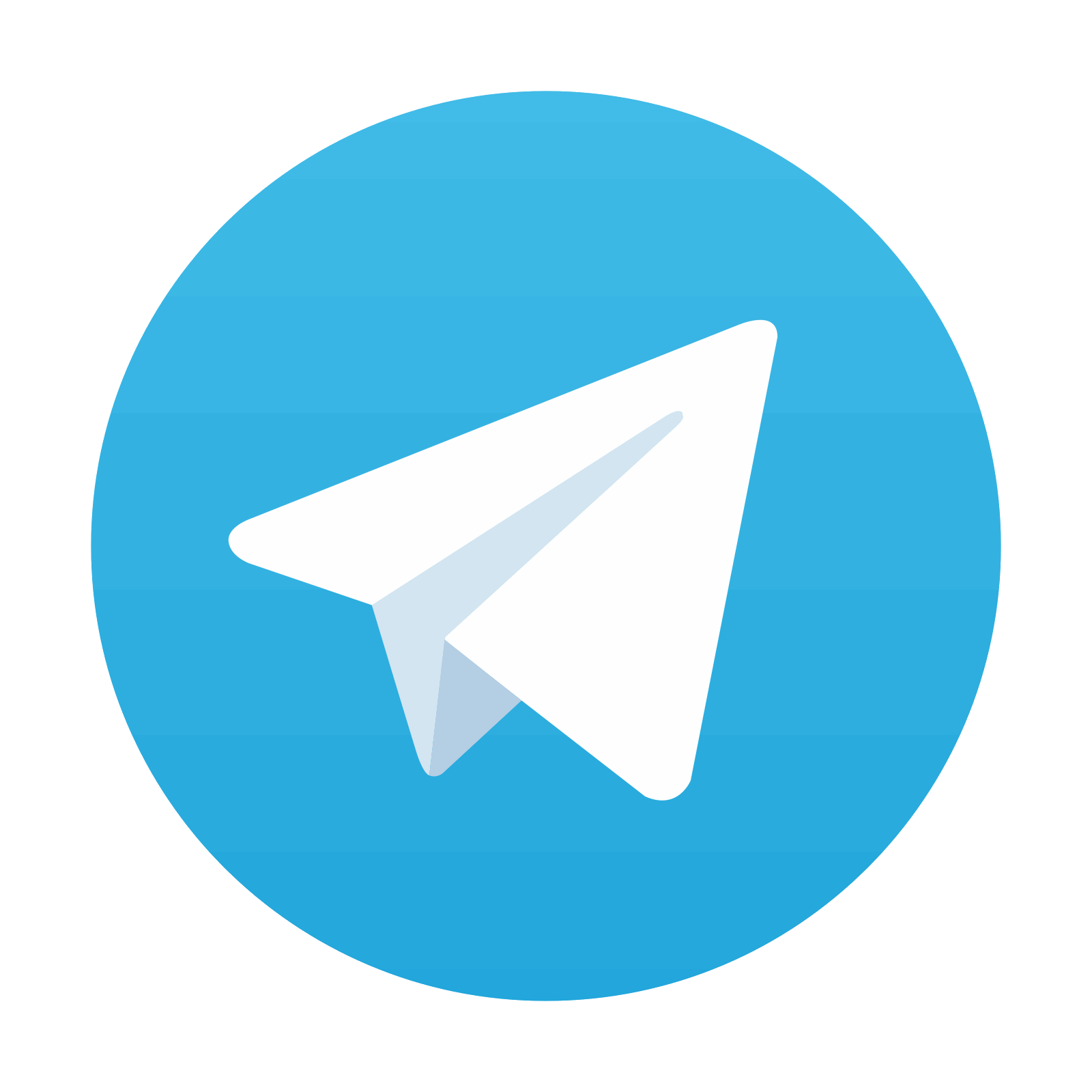
Stay updated, free articles. Join our Telegram channel

Full access? Get Clinical Tree
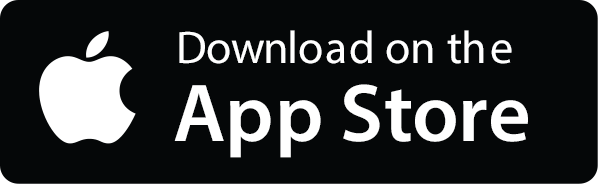
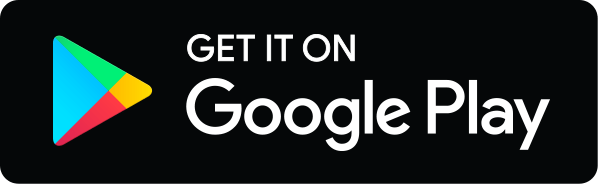