Chapter 31 Healing of Knee Ligaments and Menisci
Ligaments
Structure and Function
Anatomy and Biochemistry
Ligaments are fibrous bands that span two or more bones and are critical to the static stability of the knee joint.53 All ligaments are composed of densely organized, fibrous connective tissue consisting of mainly water and type I collagen (approximately 70% to 90% of dry weight).65 Water is attracted into the extracellular matrix of the ligament by negatively charged proteoglycans, including decorin sulfate, chondroitin sulfate, and keratin sulfate (less than 1% of dry weight). This accounts for the ligament’s rate-dependent response to mechanical load, or viscoelasticity.
Collagen is responsible for the ligaments characteristic form and tensile strength. Collagen molecules orient along the long axis of the ligament to form fibrils.65 Ligaments contain a bimodal distribution of collagen fibril diameters. There is a group of fibrils between 40 and 75 µm in diameter and a second group between 100 and 150 µm in diameter.44,53 The fibrils are grouped into fibers that range from 1 to 20 µm in diameter, which are then grouped into fascicles from 360 to 1500 µm in diameter. The collagen in ligaments contain stable and unstable collagen cross links and has a characteristic crimp pattern.
Other components of the extracellular matrix of ligaments include types III, IV, V, and VI collagen (3% to 90% of dry weight), elastic fibers, including elastin, fibrillin, and microfibrillar-associated glycoprotein (usually less than 5%), and a small percentage of noncollagenous proteins, including fibronectin, laminin, thrombospondin, and tenascin.53
Fibroblasts are the dominant cell type in ligaments, responsible for forming and maintaining the extracellular matrix. Other cell types present include endothelial cells, peripheral nerve cells, and tissue mast cells.53 Compared with tendons, ligaments are more metabolically active.45 Intrinsic ligament fibroblasts possess plump nuclei and have a higher DNA content compared with tenoblasts. The higher metabolic activity in ligaments may be to the result of a functional need for more rapid adaptation.
The normal ligament insertion site into bone is a highly specialized tissue that functions to transmit complex mechanical loads from soft tissue to bone.65 Ligaments, in general, have two distinct types of insertion sites: direct and indirect.110 The femoral insertion of the anterior cruciate ligament is an example of a direct insertion site. At a direct insertion site, the ligament often enters the bone directly at a right angle to the bony surface. This transition contains four distinct zones—ligament, unmineralized fibrocartilage, mineralized fibrocartilage, and bone (Fig. 31-1). Cartilage-specific collagens including types II, IX, X, and XI are found in the fibrocartilage of the insertion site, with collagen X playing a fundamental role in maintaining the interface between mineralized and unmineralized fibrocartilage.57,102 Proteoglycans are also abundant in the fibrocartilaginous region of the insertion site, probably functioning to decrease stress concentration at the insertion.
An example of an indirect insertion is the tibial insertion of the medial collateral ligament. At this insertion site, collagen fibers blend with the periosteum, which are then anchored to bone via Sharpey’s fibers. These Sharpey’s fibers are obliquely oriented to the long axis of the bone, securely anchoring the ligament into bone and conferring mechanical strength (Fig. 31-2).65
Biomechanics and Function
The biomechanical characterization of a healing ligament is based on two elements, functional testing and tensile testing. Functional testing determines the contribution of the ligament to knee kinematics as well as the in situ forces of the ligament in response to external loading conditions. Tensile testing assesses the structural properties of the bone-ligament-bone complex and the material properties of the ligament substance.147,149
There are four basic aspects of ligament mechanical functional testing. These include laxity, stiffness, strength, and viscoelasticity.53 Failure or overload of these functions may result in ligamentous injury and loss of critical joint-stabilizing properties of the ligament (Fig. 31-3). Healing of a ligament may be assessed using these parameters as outcome measures for success.
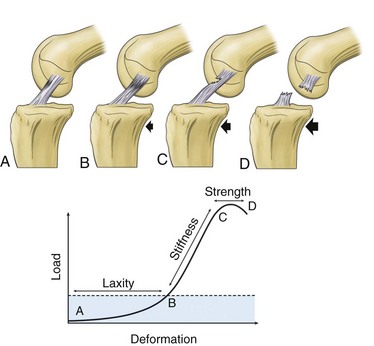
Figure 31-3 Schematic and graphic descriptions of laxity, stiffness, and strength.
(From Frank CB: Ligament healing: current knowledge and clinical applications. J Am Acad Orthop Surg 4:74–83, 1996.)
Laxity refers to the displacement of bones to which a ligament is attached from an anatomic position to a position in which the ligament takes up load. It is a function of both joint position and direction of load.53 Structurally, the laxity of a ligament is partly a function of number of fibers recruited by a specific movement and the orientation of these fibers to resist displacement.
Stiffness is the amount of load required to displace the bones to which a ligament is attached. The more load required, the stiffer the ligament-joint complex. Stiffness is also a function of fiber recruitment, with stiffer ligament having more fiber recruitment. In injured or damaged ligaments, either fibers are not recruited or those recruited are not as stiff as normal ligament fibers.54
Strength refers to the maximum tensile load that a bone-ligament-bone complex can withstand before it fails. Failure load is a function of both the number of fibers that tighten within a ligament, and the quality of those fibers. The direction of applied force during ligament testing also influences the structural and mechanical properties. Applying the force in the direction of the ligament will recruit a greater proportion of fiber bundles and thus result in higher forces.27 Therefore, load direction is a critical determinant of ligament strength.
Viscoelasticity refers to the ability of tissues to respond to repeat loading by altering length or load over time. Variations can account for as much as 10% changes in ligament length and up to 60% to 70% of changes in ligament loads under physiologic conditions. Creep is a viscoelastic property in which there is an increase in ligament length that occurs over time when the ligament is subjected to a constant load (Fig. 31-4). Another property, load or stress relaxation, occurs when less load is required over time to hold a ligament at a specific deformation.
Tensile testing provides information on the strength and quality of healing tissue and allows comparisons with intact ligaments. Two sets of information can be obtained from uniaxial tensile testing. The load-elongation curve demonstrates the structural properties of the bone-ligament-bone complex, and the stress-strain curve demonstrates the material properties of the ligament substance148 (Figs. 31-5 and 31-6). Both curves demonstrate characteristic toe, linear, and failure regions. In the initial low-stiffness toe region, elongation of a ligament occurs because of straightening of the crimp pattern.27 With further load, there is increasing recruitment of ligament fiber bundles. The slope of the linear region reflects the stiffness of the ligament or ligament complex. Structural properties that are evaluated with the load-elongation curve include linear stiffness, ultimate load, and energy absorbed at failure. The stress-strain curve provides information on modulus of elasticity, tensile strength, ultimate strain, and strain energy density.
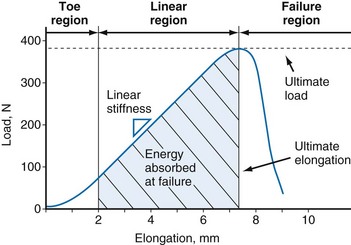
Figure 31-5 Typical load-elongation curve of the bone-MCL-bone complex.
(From Woo SL, Vogrin TM, Abramowitch SD: Healing and repair of ligament injuries in the knee. J Am Acad Orthop Surg 8:364–372, 2000.)
Biology of Ligament Injury and Healing
Ligament healing involves the restoration of the structural integrity of the tissue following an injury. The ability of an injured ligament to heal is affected by a number of variables, including the site and severity of the injury, presence of multiligamentous injury, various intrinsic factors (e.g., age, circulation status), type of treatment initiated, and degree of immobilization and rehabilitation after injury.148 Knowledge of the basic science of ligament injury and healing is instrumental in making informed treatment decisions in a clinical setting.
Age-Related Changes in Ligament and Ligament Degeneration
The structural and mechanical properties of ligaments change with skeletal maturation.6,122 In the setting of open physes, ligaments often fail by bone avulsion, whereas ligaments fail in the midsubstance in the skeletally mature. Structural and mechanical properties of ligaments are also positively correlated with age; specimens from younger donors demonstrate superior properties compared with specimens from older donors.138 Water content, as well as rate of collagen synthesis, decreases with age in both the medial collateral ligament (MCL) and anterior collateral ligament (ACL) in a rabbit model.
The gross and microscopic changes of ligament degeneration can occur in acute and chronic settings. Following acute, full-thickness ligament disruption, an initial inflammatory response occurs whether the ligament is intra-articular or extra-articular. As an example, the torn ends of an acutely ruptured ACL rapidly involute. These changes are related to an increase in collagenase activity, apparently in response to local cell damage.4 Chronic degeneration of a ligament is uncommon, but when it occurs several histologic changes are involved, including collagen fragmentation and mucoid degeneration.120
Healing of Extra-articular Ligaments
Extra-articular healing proceeds in three phases: (1) inflammation; (2) cellular and matrix proliferation; and (3) remodeling.48,146 During phase I, a fibrin clot forms at the injury site. Potent vasodilators are released, including histamine and serotonin. There is infiltration of inflammatory cells and phagocytosis of necrotic debris by macrophages, followed by proliferation of capillary endothelial buds and fibroblast proliferation near the end of phase I. These fibroblasts begin early matrix synthesis. This early matrix is disorganized.83
Matrix and cellular proliferation are characterized by a proliferation and migration of fibroblasts to the site of injury.83 Proliferating cells are derived from intrinsic and extrinsic populations. Collagen and glycosaminoglycan (GAG) synthesis is upregulated. Type III collagen, formed in the initial healing period, is removed and replaced by type I collagen. The mechanical strength of the repair tissue begins to increase as new matrix is deposited.
There is a relative decrease in cellularity and vascularity during the remodeling phase of healing. There is an increase in collagen density and a gradual decrease in the content of collagen type III. Collagen fibrils gradually become organized along the axis of the ligament. Collagen cross linking and post-translational modifications occur during this phase and result in an increased tensile strength. Collagen fibril diameters and collagen cross links remain abnormal for at least 1 year after ligamentous injury.84,98 Alpha smooth muscle actin containing fibroblasts, or myofibroblasts, may be responsible for tensioning the ligament during the remodeling phase of healing.48
There is increased matrix synthesis and cellularity seen throughout the entire ligament (not just at the injury site) during the healing phase. Near the end of the remodeling phase, the uninjured ligament returns to normal cellularity and matrix organization, whereas scar tissue persists at the injury site.67,87 A few early studies optimistically noted apparent ligament regeneration, but subsequent studies have shown that although normal ligament strength may recover in some instances, this is not because of formation of true ligament tissue.54,147 Although the strength and stiffness of the collateral ligaments have been restored to 40% to 90% of normal values in animal studies, only about 30% to 70% of the material strength has returned. This scar tissue has inferior material properties compared with normal ligament matrix. It is weaker and creeps more than normal ligament and is also associated with an increased concentration of minor collagens (types III, V, and VI), decreased collagen cross links, and an increased amount of glycosaminoglycans (Table 31-1).74
Table 31-1 Differences Between Normal Ligaments and Scars
Normal (Uninjured) Ligaments | Ligament Scars |
---|---|
Collagen aligned | Collagen disorganized |
Collagen densely packed | Defects between collagen fibers |
Large collagen fibrils | Small collagen fibrils |
Mature fiber cross links | Immature cross links |
Primarily collagen type I (<10% type III) | More collagen type III |
Small proteoglycans | Some large proteoglycans |
Other components minor | Excesses of other components |
Low cell density | Increased cell density |
Low vascularity | Increased vascularity |
From Frank CB: Ligament healing: current knowledge and clinical applications. J Am Acad Orthop Surg 4:74–83, 1996.
Anterior Collateral Ligament Injury and Healing Response
In adults, most ACL ruptures occur in the midsubstance of the ligament. Murray and co-workers found that following rupture, the human anterior cruciate ligament undergoes four histological phases: inflammation, epiligamentous regeneration, proliferation, and remodeling.96 Postinjury, the initial hemarthrosis results in inflammation that creates a hostile environment for ligament healing. The torn ends of the ACL do not touch, and there is no fibrin clot that forms between the ruptured ends in order to act as a scaffold for subsequent repair.97 Matrix metalloproteinases (MMPs) and other degradative enzymes, including fibrinolysates that degrade fibrin clot, are normally present in the synovial fluid but are upregulated following injury. These molecules degrade the remnants of the ACL and make healing even more difficult. They also observed the formation of an alpha-smooth muscle actin-expressing synovial cell layer on the surface of the ruptured ends of the ligament and postulate that it is this layer that may inhibit a healing response following anterior cruciate ligament injury and direct repair. For these reasons, the ACL rarely heals following rupture. Therefore, ACL reconstruction is the preferred surgery for patients with symptomatic knee instability.
Biologic Differences Between Anterior Collateral Ligament and Medial Collateral Ligament Healing
In direct contrast to the findings in ACL injuries, both clinical experience and animal studies of MCL injuries have indicated a relatively good, but seldom perfect, healing response.54,78,79 Numerous studies have examined the mechanisms accounting for the different healing potentials of the ACL and MCL.5 Multiple factors account for the differences, including ligament ultrastructure, local environment, and cellular properties. There are differences in fibril diameter, fibril diameter distributions, and subfascicular area fractions. Medial collateral ligament fibril diameters are larger and the subfascicular area fraction is higher in the MCL, indicating more densely packed fibrils.6 Cellular metabolism may also play a role in healing potential. MCL fibroblasts proliferate more rapidly than ACL fibroblasts in vitro and they have differential response to growth factors. There are also differences in cellular response to various chemotactic agents, such as cytokines. ACL and MCL cells demonstrate differential mitogenic, chemotactic, and matrix synthetic responses to mechanical load. There is increased expression of specific integrins on the cell surface of MCL fibroblasts in the healing MCL as compared with minimal integrin expression in the healing ACL cells. Messenger RNA for procollagen expression is also higher in the healing MCL compared with the healing ACL.
Biomechanical factors may also play a role in the ability to heal. The ACL contributes to knee stability in multiple directions whereas the MCL primarily restrains valgus rotation. Therefore, a ruptured MCL receives some protection from other structures, such as the ACL and capsule, and may not be subjected to the same forces that could impede ACL healing.148 The ACL may not be able to accommodate the multidirectional demands so as to allow healing.149 Knowledge of the in vivo ligamentous loads would help determine the optimal load and amount of strain to optimize ligament healing.
Combined Ligamentous Injuries
The prognosis for combined ACL-MCL injuries is generally worse than for single-ligament injury, regardless of selected treatment modality.56,76,148 Although the clinical treatment of these combined injuries remains controversial, evidence from recent animal models may assist in clinical decision making with regard to optimization of ligament healing potential.
Rabbit and canine models have studied the effects of ACL deficiency on the healing of the injured MCL. In these models, knees with untreated combined injury demonstrated increased valgus laxity and significant reduction in the tissue quality of the healed MCL.149 In contrast, another rabbit study demonstrated that reconstruction of the ACL combined with nonoperative full weight bearing and mobilization of the MCL leads to successful MCL healing.150 Studies of ACL reconstruction combined with MCL repair have demonstrated improved structural properties and functional testing of the MCL in the short term, with no biochemical or biomechanical difference versus nonoperative MCL treatment after 52 weeks.
Intrinsic Factors
The healing response of ligaments is affected by a variety of endocrine or metabolic abnormalities, systemically or locally. Systemic factors include diabetes mellitus and its effect on the circulatory system, insulin deficiency, and alteration of collagen synthesis and cross linking, and the effect of various changes in the endocrine system on ultimate load of the repaired ligament, and the rates of collagen and glycosaminoglycan synthesis or degradation.135 Local factors, such as poor circulation or infection, impede the proliferation of cells and prolong the inflammatory phase of healing.148
Treatment of Ligamentous Injuries
Immobilization versus Controlled Motion and Exercise
Rehabilitation of an injured ligament often depends on whether there is joint instability associated with the injury. Stable joints may be treated with immobilization for varying time periods, followed by exercise protocols to gain motion and strength. Unstable joints may additionally be treated with specific bracing techniques or surgical intervention. The effects of proper techniques in rehabilitation on patient outcome have been studied in several areas. Injured ligaments have abnormal proprioception and training regimens may improve this. Supervised rehabilitation of postural and balance training may reduce the number of re-injuries in the ankle and play a role in injury prevention.77 Joint position sense (JPS) in ACL-deficient knees has been described as impaired. Although knee stability can improve with exercise therapy, there may be no improvement in JPS.31 The role of JPS in the stability of ACL-deficient knees remains unclear.62
Postoperative rehabilitation contributes greatly to the success of ACL reconstruction. Early joint motion is beneficial for reducing pain, improving articular cartilage nutrition, and minimizing scar formation that limits joint motion. Functional sports agility programs during the early rehabilitation period after ACL reconstruction are well tolerated and beneficial to overall outcome.124
Aggressive rehabilitation programs that involve contraction of the dominant quadriceps muscles have now become popular. Closed kinetic chain exercises (foot fixed against a resistance) are the mainstay of rehabilitation of ACL-insufficient or ACL-reconstructed knees. Some authors have suggested that open- and closed-chain exercises can be modified to minimize the risk of applying excessive strain on the ACL graft and of excessive patellofemoral joint stress.44,51 However, open kinetic chain exercises (foot not fixed against a resistance) may result in increased anterior-posterior knee laxity compared with the normal knee.89 The relationship between rehabilitation exercises and the healing response of an ACL graft is still not completely understood.
Bracing
Functional knee braces provide a protective strain-shielding effect on the normal ACL when anterior shear loads and internal rotation torques are applied to the knee in non–weight-bearing and weight-bearing conditions.19 However, this protective effect is at loads that are less than those seen in normal walking. Future studies should strive to determine the actual loads transmitted across the knee and ACL graft strain during various rehabilitation exercises and relate these to the healing response of the knee and graft.18
Electrical Stimulation
Electrical stimulation has been shown to enhance the repair of biologic tissues such as bone and tendon. The use of direct current yielded improvements in maximum rupture force, energy absorbed, stiffness, and laxity in the rat MCL.87
Intra-articular Ligament Reconstruction
Tendon Graft Biology
When surgical intervention is indicated for the treatment of a damaged ligament, a tendon graft is often used for the reconstruction. The tendon is placed in a new biologic and mechanical role. There is a gradual biologic transformation of tissues that are transplanted to an intra-articular environment, such as in ACL reconstruction. This process has been termed ligamentization5 (Fig. 31-7). This gradual biologic transformation of the transplant begins with graft avascular necrosis. The intrinsic graft cells do not survive transplantation to the new environment. Cell necrosis occurs over the first 3 weeks. This is followed by cellular repopulation, which occurs prior to revascularization of the ligament. Repopulating cells appear to be derived from synovium and obtain their nutrition by synovial diffusion. Other possible sources of repopulating cells include mesenchymal stem cells, marrow cells, blood cells, and cells from the residual ACL stump.4,96 It is also possible that there is a surviving subset of cells in the graft, although this is much less likely. The eventual phenotype of the repopulating cells is not known. It is not known whether these cells assume the phenotype of normal ACL cells. These cells remain metabolically active for a prolonged period of time during the healing process of intra-articular ligament grafts.
The revascularization phase begins 6 to 8 weeks following graft transplantation.5 Vascular synovial tissue forms around the graft. New blood supply to the graft is derived from the fat pad, tibial remnant, and posterior synovial tissues. Growing capillary buds are seen histologically. Revascularization progresses from the periphery of the graft to the central portion of the graft.
Matrix synthesis occurs in the ligament graft following cellular repopulation and revascularization.5 Over time, the collagen and glycosaminoglycan content of the graft becomes similar to a normal ligament.23 Similarly, the collagen crimp pattern and reducible cross link profile becomes more like that of a normal intra-articular ligament as compared with the extra-articular tendon graft. However, there is a persistent abnormal unimodal distribution of small diameter collagen fibrils in the graft. There is variable return of the pyridinoline collagen cross links. Type III collagen also has been found to remain elevated for a variable period of time following graft transplantation. These persistent abnormalities in collagen cross links, collagen type, and collagen fibril diameter probably contribute to the inferior biomechanical properties of the graft.
The last phase in the graft incorporation process is the remodeling phase.5 There is a gradual decrease in cellularity and vascularity and an increase in matrix organization. This process occurs for a minimum of 1 year following graft transplantation.
Graft Attachment Site Biology
Surgical reconstruction of a ligament relies on the grafted tendon to attach to the bones previously connected by the torn ligament. Tendon to bone healing occurs by the formation of a fibrovascular interface tissue between the tendon and bone. In this way, the tendon becomes anchored to the bone. There is gradual reestablishment of collagen fiber continuity between the tendon and bone. Some animal studies have demonstrated direct collagen fiber continuity between the tendon and bone (Sharpey’s fibers), whereas other studies have demonstrated formation of a fibrocartilage interface between tendon and bone.110,111 The concomitant bone formation is generally an intramembranous process. The attachment strength gradually increases as collagen fiber continuity improves.
Bone plug healing, such as seen in bone-tendon-bone ACL reconstructions, involves incorporation of the bone plug into the bone tunnel over a 12-week period.5 The original insertion site of the patellar tendon may remain histologically normal. The comparative strength of the healed tendon to bone attachment versus the healed bone plug attachment is unknown.
Healing of a tendon graft in a bone tunnel, as is required in ACL reconstruction using semitendinosus tendon, begins with the formation of a fibrovascular interface tissue between the tendon and bone.110 There is progressive bone ingrowth into this interface tissue, with gradual reestablishment of an indirect type of insertion by intramembranous bone formation. The increase in strength of the healing tendon to bone attachment correlates well with the progressive bone ingrowth and maturation of the interface (Figs. 31-8 and 31-9).
Healing of tendon to the surface of bone also occurs by bone ingrowth into the fibrous interface tissue that forms between the tendon and bone.116 In a larger animal model (goats), an indirect insertion forms, whereas studies in lower animals have demonstrated formation of a direct insertion. Further study is required to determine the basic cellular mechanism of tendon to bone healing. Studies have demonstrated that bone morphogenetic protein-2, a potent osteoinductive agent, can augment tendon healing in a bone tunnel.111
Future Directions in Ligament Healing
Researchers are actively exploring methods to improve the quality of healing ligament tissue and to find novel ways to accelerate the healing process. Advances in the fields of molecular biology and biochemistry may have applications in the ligament healing process.148 These approaches include innovative biologic and bioengineering techniques of using growth factors, gene transfer therapy, cell therapy, scaffolding materials, and mechanical stimuli.22
Growth Factors
Growth factors such as epidermal growth factor (EGF), basic fibroblast growth factor (bFGF), acidic fibroblast growth factor (aFGF), platelet-derived growth factor BB (PDGF-BB), and transforming growth factor beta (TGF-β) are currently being evaluated for their effects on fibroblast proliferation, matrix synthesis, and cell migration. Animal models have shown that TGF-β is a good promoter of matrix synthesis, whereas PDGF-BB, EGF, and bFGF are positive mitogens on fibroblasts of the ACL and MCL.118,148 Investigators have demonstrated the possibility that growth factors can enhance the in vivo healing of injured ligaments.84,145 In one rabbit model, high doses of PDGF delivered with a fibrin sealant led to significant increases in the structural properties of the femur-MCL-tibia complex.75
Gene Transfer
Gene transfer technology has been developed to design and enhance delivery vehicles for growth factors. Controlling and regulating the expression of proteins within a host cell will enable researchers to administer treatments over a prolonged period of time.148 Although the technology for treating ligamentous injuries is still somewhat novel, preliminary studies have shown positive effects on collagen fibril diameter and distribution, as well as significant increases in mechanical properties.100
Cell Therapy
The concept of cell therapy is that implantation of genetically manipulated cells can enhance the repair of ligaments as those cells become constituents of the healing tissue.148 Both in vivo and in vitro studies have already demonstrated that mesenchymal stem cells are capable of differentiating into many cell types involved in ligamentous healing. A study by Watanabe and colleagues141 has demonstrated the ability of nucleated donor bone marrow cells to migrate to the area of MCL injury after transplantation. Cell therapy may lead to new methods of treatment for ligament injuries in the future.
Biologic Scaffolds
Biologic scaffolds offer the potential to accelerate ligament and tendon healing and regeneration. Particular interest has been on the porcine small intestine submucosa. Research has shown that this submucosa can be modified in vitro by seeding marrow stromal cells on the scaffold and by applying cyclic stretching to increase cell alignment. When applied in vivo, the tissue-engineered scaffold could potentially accelerate the healing process.22 Further research is needed in this area before these scaffolds gain widespread clinical applications.
Menisci
Structure and Function
The menisci of the knee are C-shaped wedges of fibrocartilage interposed between the condyles of the femur and tibia. They are actually extensions of the tibia that serve to deepen the articular surfaces of the tibial plateau to accommodate the condyles of the femur better. The peripheral border of each meniscus is thick and convex and attached to the joint capsule, whereas the inner border tapers to a thin, free edge.140 The proximal surfaces of the menisci are concave and in contact with the condyles of the femur; their distal surfaces are flat and rest on the tibial plateau (Fig. 31-10).
The medial meniscus is somewhat semicircular in form, approximately 3.5 cm in length and considerably wider posteriorly than anteriorly. The anterior horn of the medial meniscus is attached to the tibial plateau in the area of the anterior intercondylar fossa, anterior to the ACL (Fig. 31-11). The posterior fibers of the anterior horn merge with the transverse ligament, which connects the anterior horns of the medial and lateral menisci.140 The posterior horn of the medial meniscus is firmly attached to the posterior intercondylar fossa of the tibia between the attachments of the lateral meniscus and posterior cruciate ligament. The periphery of the medial meniscus is attached to the joint capsule throughout its length. The tibial portion of the capsular attachment is referred to as the coronary ligament. At its midpoint, the medial meniscus is more firmly attached to the femur and tibia through a condensation in the joint capsule known as the deep medial collateral ligament.
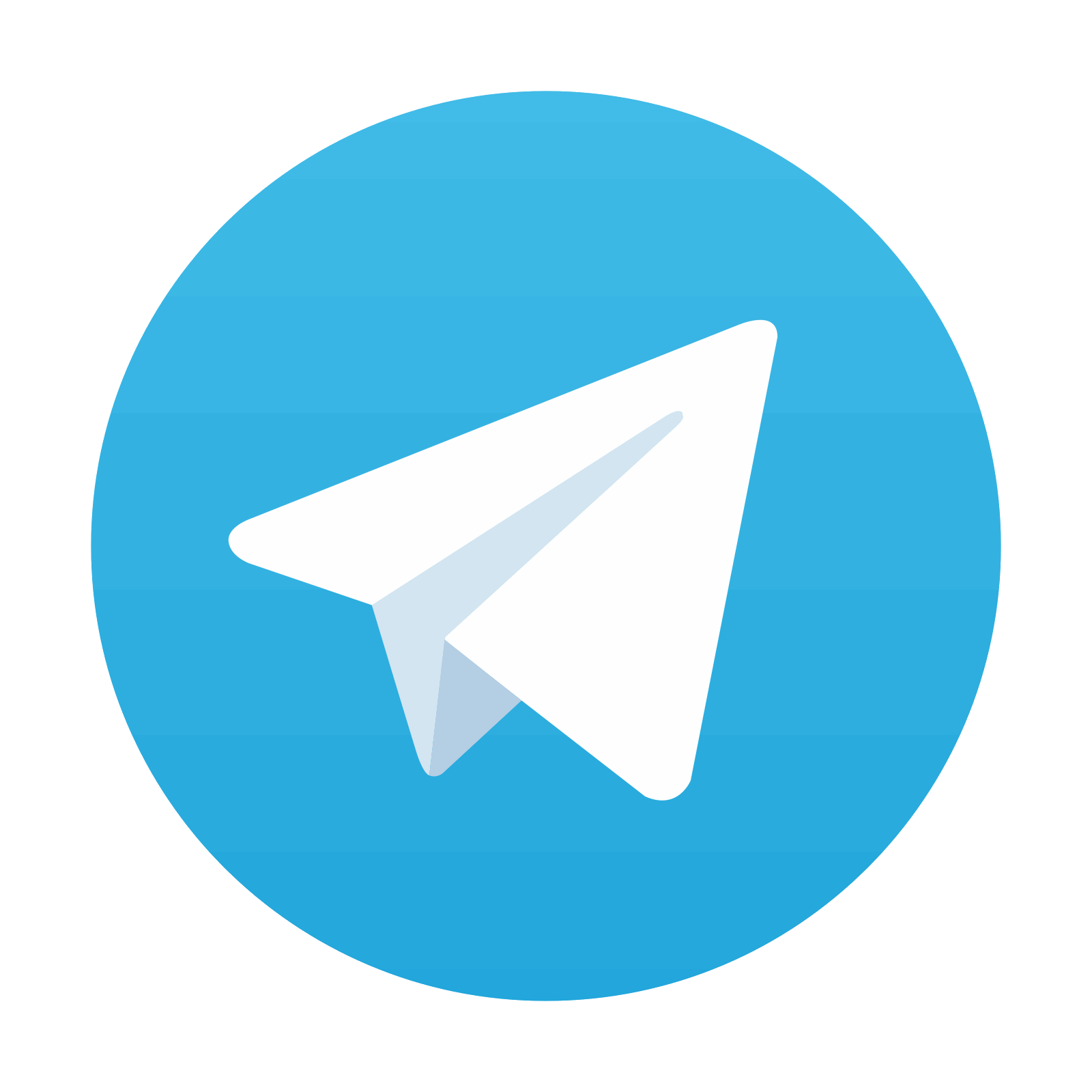
Stay updated, free articles. Join our Telegram channel

Full access? Get Clinical Tree
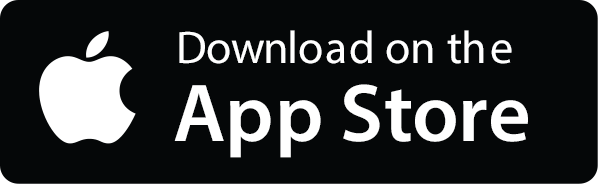
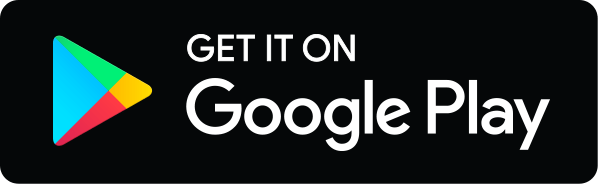