CHAPTER 22 Glenohumeral Arthritis and Its Management
The humeral head and the glenoid normally articulate through smooth, congruent, and well-lubricated joint surfaces. Glenohumeral arthritis results when these joint surfaces are damaged by congenital, metabolic, traumatic, degenerative, vascular, septic, or nonseptic inflammatory factors. These conditions are common, especially in older populations, in whom the prevalence is approximately 20%.1–3
MECHANICS OF ARTHRITIS AND ARTHROPLASTY
Motion
Normal Capsular Laxity
In normal shoulders, ample capsular laxity allows full range of rotation at the glenohumeral joint. The glenohumeral capsule is normally lax through most of the functional range of shoulder motion.4,5 As the joint approaches the limit of its range, the tension in the capsule and its ligaments increases sharply, thereby serving to check the range of rotation (Fig. 22-1). In many conditions that require shoulder arthroplasty, the capsule and ligaments are contracted, thus limiting the range of rotation (Fig. 22-2). Shoulder arthroplasty can further tighten the capsule if the degenerated and collapsed humeral head is replaced by a relatively larger prosthesis and if a glenoid component is added to the surface of the glenoid bone. The prosthetic components might therefore consume more volume than the degenerated surfaces that they replace (Fig. 22-3) and consequently stuff the joint. Unless sufficient capsular release (Figs. 22-4 and 22-5) has been performed to accommodate this additional volume, the joint may be overstuffed. In this situation, joint motion is further limited (Fig. 22-6), and more torque (muscle force) is required to move the arm (Fig. 22-7).
Harryman and associates determined that all motions, including flexion, external and internal rotation, and maximal elevation, are diminished when the joint is overstuffed.6 Furthermore, this overstuffing causes obligate translation of the humeral head on the glenoid; for example, forced posterior translation occurs when external rotation is attempted against a tight anterior capsule (Figs. 22-8 and 22-9). Thus, if normal capsular laxity is lacking, unwanted translation and eccentric glenoid loading can result. Blevins and colleagues studied the effect of humeral head size on translation and rotation at the glenohumeral joint and determined that selection of head component size should be based more on restoring effective joint volume than on replicating the native head diameter.7 Vaesel and coworkers demonstrated in a cadaveric model that humeral head mismatch resulted in differing displacements depending on whether the head was too small, leading to inferior displacement, or too large, leading to superior displacement. In addition, they found that a press-fit prosthesis required a volume 1.25 times the volume of the native head to restore the normal kinematics of the glenohumeral joint.8
In arthroplasty surgery, the contribution of the components to joint stuffing can be estimated by adding the incremental thickness of the glenoid to the difference between the amount of intra-articular humerus replaced and the amount of humerus resected. To be comparable, the measurement of the amount of humeral head resected and the measurement of the amount of intra-articular humeral prosthesis added must both be made from the cut surface of the humeral neck to the articular surface. In modular humeral heads, the amount of bone replaced must include the thickness of the collar and the exposed part of the Morse taper stem, in addition to the head itself (see Fig. 22-3).
The incremental thickness of the glenoid is related primarily to the thickness of the component, as well as the amount of reaming, the presence or absence of cement between the component and the bone, and the effect of bone grafts (Fig. 22-10). The thickness of currently available glenoid components varies from 3 mm to more than 15 mm. Thicker glenoid polyethylene might help manage contact stress and might have superior wear properties.9 Metal-backed glenoid components affect load transfer and offer opportunities for screw fixation and tissue ingrowth.10 However, both thicker polyethylene and metal backing increase joint stuffing, which becomes particularly problematic in shoulders that remain tight even after soft tissue release. Overstuffing can also predispose a reconstructed shoulder to instability.10 In a finite element model, the peak stress generated at the glenoid component was as high as 25 MPa. This is significantly higher than polyethylene yield strength, and therefore wear and cold flow is to be expected.11 In addition, metal-backed components promoted higher contact stress, leading to increased wear in comparison to all-poly-ethylene components.
The amount of stuffing from the humeral component is determined both by the geometry of the component and by the position in which it is placed. The size of the intra-articular aspect of the humeral component is related to its design, including its radius of curvature, the percentage of the sphere represented by its articular surface, and the distance between the base of its collar and the articular surface of the prosthesis (Figs. 22-11 and 22-12). The position of the component also has a major effect on the degree to which it stuffs the joint. A component inserted into varus will disproportionately stuff the joint when the arm is at the side; this outcome is more likely when the stem of the prosthesis does not fit the humeral canal snugly. A component inserted excessively high tightens the capsule as the arm is elevated (similar to a mechanical cam) and limits the range of elevation (Figs. 22-13 and 22-14).
Cadaver studies12 indicate that less than 10 mm of overstuffing can reduce normal capsular laxity by as much as 50% (Fig. 22-15). An overstuffed shoulder is predisposed to obligate translation when rotated to positions in which the capsule becomes tight (Table 22-1). Overstuffing can be avoided by ensuring adequate capsular laxity at the time of surgery: The arm should allow 40 degrees of external rotation at the side after the anterior structures have been approximated (Fig. 22-16), the humeral head should translate approximately 50% of the glenoid width on the posterior drawer test (Fig. 22-17), and the abducted arm should allow 60 degrees of internal rotation (Fig. 22-18). These are the 40, 50, 60 guidelines.
TABLE 22-1 Range of Angular Motion Before the Onset of Obligate Translation*
Angular Motion | Anatomic Shoulder | Joint Overstuffed 9mm |
---|---|---|
Elevation in the +90-degree scapular plane | 60 degrees | 30 degrees |
External rotation of the arm elevated 50 degrees | 60 degrees | 32 degrees |
* Values represent the maximal elevation achieved with no more than 2 mm of obligate translation.
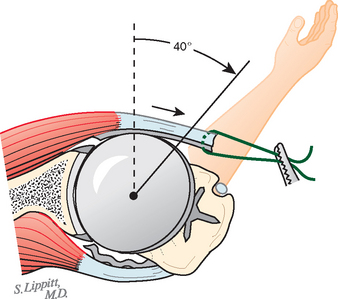
FIGURE 22-16 The 40, 50, 60 rule: External rotation. Forty degrees of external rotation with the subscapularis approximated.
(From Matsen FA III, Lippitt SB: Shoulder Surgery: Principles and Procedures. Philadelphia: WB Saunders, 2004, p 478.)
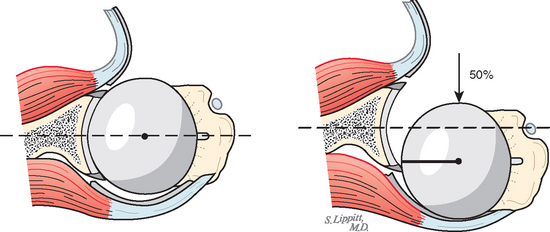
FIGURE 22-17 40, 50, 60 rule: Posterior drawer. Fifty percent posterior subluxation on the posterior drawer test.
(From Matsen FA III, Lippitt SB: Shoulder Surgery: Principles and Procedures. Philadelphia: WB Saunders, 2004, p 479.)
Humeral Articular Surface
A substantial and properly located humeral articular surface area allows a large unimpeded rotational range. Humeral articular surfaces that comprise a reduced portion of the sphere (Figs. 22-19 and 22-20; see Fig. 22-11) diminish the amount of range of motion with full surface contact at the glenohumeral joint and predispose to abutment of the rim of the glenoid against the tuberosities or anatomic neck of the humerus (Figs. 22-21 and 22-22).13 The normal extent of the humeral joint surface can be restored with proper positioning of the appropriate prosthesis at the time of joint replacement. Williams and coworkers investigated the effect of humeral head malposition after total shoulder arthroplasty on joint translation and range of motion. An offset of 8 mm or more in any direction resulted in a significant decrease in passive range of motion, whereas inferior malposition of greater than 4 mm led to increased subacromial contact. The authors determined that anatomic reconstruction of the humeral head—humeral shaft offset to within 4 mm is desirable for restoring normal motion and articular kinematics.14
Glenoid Articular Surface
The glenoid articular surface encompasses a relatively small portion of the sphere when compared with the articular surface of the humerus. If the prosthetic glenoid joint surface area is large in comparison to that of the humerus, abutment of the prosthesis against the humeral neck or tuberosities can restrict joint motion (Fig. 22-23).
Concentricity of the Coracoacromial and Glenohumeral Spheres
The centers of the articulation between the humeral head and glenoid on the one hand and the proximal humeral convexity and the coracoacromial arch on the other need to be concentric (Figs. 22-24 and 22-25).
Absence of Blocking Osteophytes
Osteophytes predispose to contact with the glenoid and can impair motion (Fig. 22-26). Blocking osteophytes must be completely resected at the time of joint reconstruction (Fig. 22-27).
Unrestricted Humeroscapular Motion Interface
Normally, 4 to 5 cm of excursion takes place at the upper aspect of the interface between the coracoid muscles and the subscapularis (Figs. 22-28 and 22-29). Bursal hypertrophy, adhesions, or spot welds between the proximal end of the humerus and the cuff on one hand and the deltoid and coracoacromial arch on the other can limit motion, even if the intra-articular aspect of the arthroplasty is perfectly balanced (Fig. 22-30). Lysis of humeroscapular spot welds is an important early step in arthroplasty of the shoulder.
Stability
Anatomically Oriented and Sufficiently Extensive Humeral Articular Surface Area
The orientation of the humeral articular surface can be described in terms of the humeral head centerline, or a line passing through the center of the humeral joint surface and the center of the anatomic neck. This line usually makes a valgus angle of about 130 degrees with the humeral shaft. The humeral head centerline generally makes a retroversion angle of about 30 degrees with the axis of elbow flexion.15–23 Studies point out that mean humeral retroversion varies widely from 7 to 50 degrees.24–26 Badet and coworkers demonstrated that humeral retroversion is decreased by as much as 8 degrees in shoulders with osteoarthritis and that humeral head subluxation is present in roughly 35% of cases.27 Hernigou and associates pointed out the importance of clearly defining the reference system when measuring humeral version.28 In one study, these authors determined that the mean angular orientation of the humeral articular surface varies by as much as 11 degrees when measured by the epicondylar axis versus a line perpendicular to the forearm axis.29
The extent of the humeral articular surface area is another critical determinant of stability. In an arthritic glenohumeral joint, stability can be compromised by a reduced amount of available humeral articular surface. Similarly, a prosthetic surface area that represents only a small part of the total sphere can predispose to instability in the same way that a Hill—Sachs defect does in traumatic instability by offering less contact area for joint surface contact (see Fig. 22-12).
Anatomically Oriented Glenoid
The glenoid centerline, the line perpendicular to the center of the glenoid fossa, is usually within 15 degrees of the plane of the scapula (Figs. 22-31 and 22-32). In an arthritic glenohumeral joint, stability may be compromised by abnormal glenoid shape and orientation (Figs. 22-33 and 22-34). Friedman and colleagues,30 Mullaji and associates,31 and Badet and coworkers27 have used computed tomography (CT) to document that arthritic involvement can alter glenoid version. Walch and colleagues reviewed the serial CT scans of 113 osteoarthritic shoulders to study the effect of the position of the humeral head on glenoid morphology.32
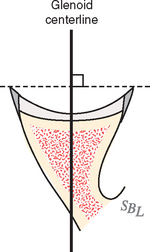
FIGURE 22-31 The glenoid centerline is perpendicular to the center of the glenoid fossa.
(From Matsen FA III, Lippitt SB: Shoulder Surgery: Principles and Procedures. Philadelphia: WB Saunders, 2004, p 481.)
Three main glenoid types were identified:
Type C (9%) was defined by glenoid retroversion of more than 25 degrees regardless of erosion.
In these cases, glenoid retroversion did not correlate with posterior wear but was primarily dysplastic in origin. The orientation of the glenoid prosthesis should be normalized as part of the arthroplasty procedure (Figs. 22-35 to 22-37).
Iannotti and colleagues found that retroversion of the glenoid component can lead to posterior instability in two ways. First, the retroverted glenoid disrupts the normal joint reactive forces and creates an off-axis moment leading to a posteriorly translated joint reactive force. Second, increased retroversion leads to a loss of the effective posterior wall height, leading to decreased constraint of the glenohumeral joint. Importantly, this cannot be corrected with humeral head position.33
Glenoid Concavity With Sufficient Intrinsic Stability
The arc of the glenoid determines the maximal angles that the net humeral joint reaction force can make with the glenoid centerline before dislocation occurs (Fig. 22-38).
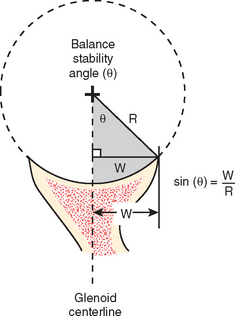
FIGURE 22-38 Calculating the balance stability angle. The predicted balance stability angle (BSA) in a specified direction for a spherical glenoid is given by the arc sine of the ratio of the glenoid width (W) in the direction of interest (the distance from the glenoid center to the reamed edge) and the radius of curvature for the glenoid. BSA = arc sin W/R, where R is the radius of the spherical glenoid. Because for small angles (e.g., <30 degrees), the sine and the tangent are about equal (see Chapter 16) and the tangent is approximately equal to the angle expressed in radians (∼57.3 degrees), we come up with the convenient general rule that the BSA ≅ 57.3 × W/R. For a normally directed glenoid concavity, the desired stability angle is at least 20 degrees. So if the glenoid width from the center to the edge is W, the radius should be less than three times the width (20 = 57.3 × W/R or R = 3 × W), or the diameter should be less than six times the width from the center to the edge. Thus a glenoid with a width of 10 mm would have the desired intrinsic stability if it had a diameter of less than 60 mm, whereas a glenoid with a width of 7 mm would need to have a diameter of less than 42 mm.
(From Matsen FA III, Lippitt SB: Shoulder Surgery: Principles and Procedures. Philadelphia: WB Saunders, 2004, p 499.)
In an arthritic joint, the effective glenoid arc can be diminished by wear or inflammation; for example, posterior wear is typical of glenohumeral osteoarthritis (see Fig. 22-34) and capsulorrhaphy arthropathy, whereas central erosion of the glenoid is typical of rheumatoid arthritis (Fig. 22-39) and superior erosion is typical of cuff tear arthropathy (Fig. 22-40). One of the goals of arthroplasty is to restore optimal bony support for the glenoid component (Fig. 22-41).
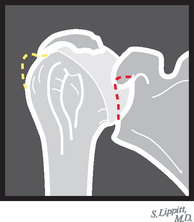
FIGURE 22-40 Cuff tear arthropathy. Superior medial erosion (dotted red line) typical of cuff tear arthropathy.
(From Matsen FA III, Lippitt SB: Shoulder Surgery: Principles and Procedures. Philadelphia: WB Saunders, 2004, p 426.)
The effect of posterior glenoid erosion can also be seen from the glenoidograms (Figs. 22-42 and 22-43; see Fig. 22-33). It is evident that burring the biconcave glenoid alone cannot re-create the normal glenoid shape and orientation (Fig. 22-44).
Control of the Net Humeral Joint Reaction Force
The direction of the net humeral joint reaction force is controlled actively by elements of the rotator cuff and other shoulder muscles (Fig. 22-45). Neural control of the magnitude of the different muscle forces provides the mechanism by which the direction of the net humeral joint reaction force is modulated. For example, by increasing the force of contraction of a muscle whose direction of force is parallel to the glenoid centerline, the body can change the direction of the net humeral joint reaction force to an orientation that is in closer alignment with the glenoid fossa. Apreleva and associates demonstrated that the magnitude and direction of the net humeral joint reaction force varies according to the relative ratio of forces applied by the rotator cuff and deltoid muscles.34
In glenohumeral arthritis, control of the net humeral joint reaction force may be compromised by tendon rupture, tuberosity detachment, and deconditioning. The most striking example is in cuff tear arthropathy, where the normally stabilizing cuff muscle forces are compromised (Figs. 22-46 and 22-47).
If the net humeral joint reaction force is not centered in the glenoid fossa after glenohumeral arthroplasty, eccentric loading can produce a rocking horse loosening of the glenoid component (Fig. 22-48). A slight degree of mismatch of the glenoid and humeral diameters of curvature allows minor amounts of force malalignment before rim contact occurs. Severt and associates35 pointed out that a high degree of conformity between the glenoid and humeral joint surfaces increases the translational force and frictional torque applied to the glenoid component and, on this basis, advocated the use of less-conforming and less-constrained designs. Karduna and colleagues noted that prosthetically reconstructed shoulders developed higher force for a given translation as conformity increased and that more conforming joints resulted in higher peak tensile strain just before rim loading.36 Anglin and associates demonstrated that more-conforming designs led to an increase in distraction of the glenoid component from the underlying bone during simulated edge loading.37
Severe degrees of mismatch can have adverse effects on the glenohumeral contact area (Fig. 22-49) and on peak stresses in the polyethylene component (Fig. 22-50). Couteau and coworkers analyzed the mechanical effect of changes in articular conformity in shoulder arthroplasty and determined that decreases in the contact area of articulation can cause high stresses that may be transferred to the glenoid fixation.38 In a finite element model, Terriere and associates demonstrated a dramatic increase in contact pressure as conformity mismatch increased and reached critical levels above 10 mm of radial mismatch. This was amplified by an additional 10% to 20% when retroversion of the component was introduced into the model.39 Thus, there must be a compromise between the arguments in favor of more mismatch between the radius of curvature of the glenoid and that of the humerus (less translational loads on the glenoid component and less risk of rim loading with translation) and those in favor of greater conformity (more stability and less contact stress) (Fig. 22-51).
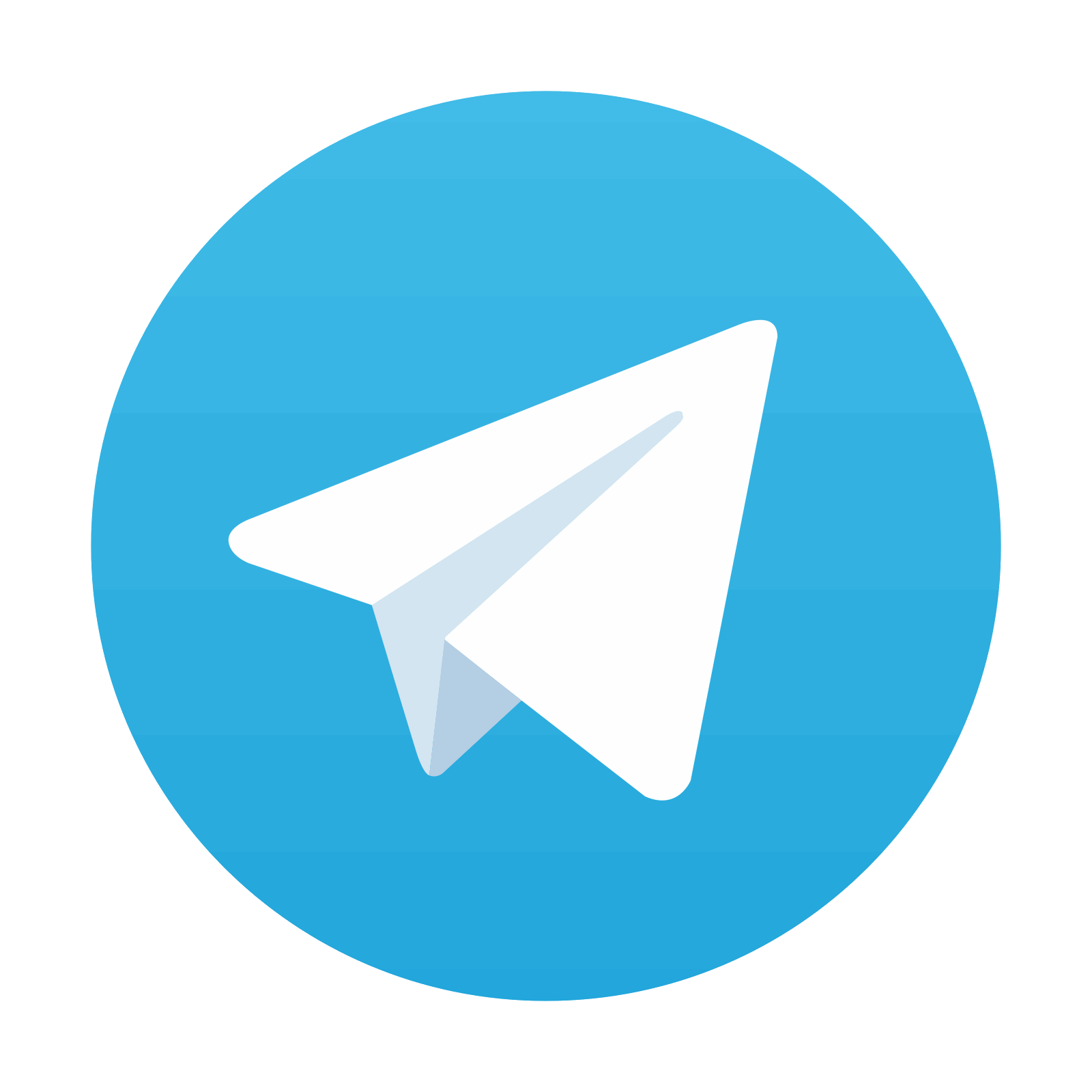
Stay updated, free articles. Join our Telegram channel

Full access? Get Clinical Tree
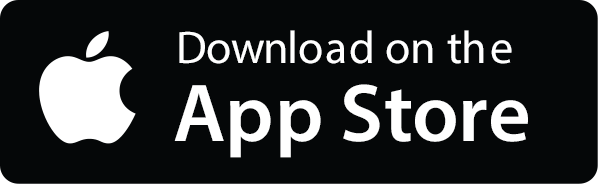
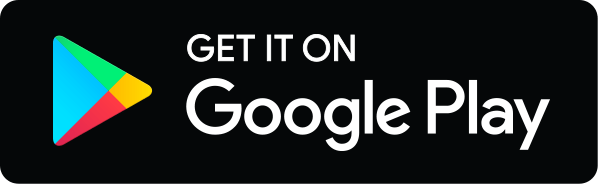
