CHAPTER 26 The Biceps Tendon
In 1983 it was not uncommon to see patients who had undergone isolated biceps tenodesis when in reality their symptoms emanated from rotator cuff disease and impingement syndrome. It is quixotic to think, however, that the biceps escapes the degenerative process or is unable to produce symptoms either on its own or in conjunction with other pathologic entities of the shoulder.
The long head of the biceps brachii is the proverbial stepchild of the shoulder. Kessell and Watson1 described the tendon as “somewhat of a maverick, easy to inculpate but difficult to condemn.” Lippman2 likened the long head of the biceps to the appendix: “An unimportant vestigial structure unless something goes wrong with it.” At various times in history, surgeons have tenodesed, translocated, pulled through drill holes in the humeral head, débrided with an arthroscope, and tenotomized this tendon. Still others have worshipped at the altar of the biceps, keeping it sacrosanct, contending that it must be there for a reason, even if it is unclear what exactly that reason is or ever was.
HISTORICAL REVIEW
The history of our understanding of the long head of the biceps is long and full of controversy. It has been at times indicted as the source of all shoulder pain; at other times it has been seen as an incidental structure of no real consequence. Hippocrates3 was the first to call attention to the pathologic displacement of muscles and tendons in dislocations. Accurate depictions of the anatomy of the biceps region and intertubercular groove appeared in the 1400s (Fig. 26-1A).
The first reported case of a long head of the biceps brachii tendon dislocation was in 1694 by William Cowper.4 He presented a case of a woman who was wringing clothes when she suddenly felt something displace in her shoulder. When Cowper examined her 3 days after the injury, he noted a depression of the deltoid, rigidity in the lower biceps, and an inability to extend the forearm. According to Cowper’s report, the tendon was reduced by manipulation, and with reduction the patient immediately recovered use of the arm. This sudden disabling episode followed by a miraculous recovery is seldom seen in our practice. Cowper had his supporters, and his theory was accepted by Boerhaave and Bromfield.5 Cowper’s observations came under suspicion, however, because of his plagiarism of the Dutch anatomist Godfried Bidloo.6 Before Cowper’s description, many biceps injuries were undoubtedly a result of direct trauma (see Fig. 26-1B).
In 1803, Monteggia7 reported a second case of dislocation but one in which the mechanism was habitual. Subsequently, numerous additional clinical reports appeared in the literature.8–12 Soden13 reported one such in 1841 and was actually able to confirm the diagnosis at autopsy. Hueter,14 in 1864, very nicely documented the signs and symptoms of lesions of the LHBT.
However, even during this relatively early period of our knowledge there was controversy. Jarjavey15 believed that most of the symptoms were attributable to subacromial bursitis and did not believe in the existence of simple luxation as a clinical phenomenon. Some authors5,16 believed that the biceps lesion was in fact secondary to arthritis or some other concomitant pathology. Callender17 mentioned one case of recurring dislocation, which he attributed to fibrous tissue within the groove. Duplay18–20 described periarthrite scapulo-humerale, a syndrome that included tendinitis of the biceps. McClellan21 believed the LHBT to be an important depressor of the humeral head. He wrote:
Bera,22 in 1910, believed that osteitis reduced the height of the lesser tuberosity and thus led to biceps instability. In the 1920s, valuable contributions were made by Meyer.23–30 He discussed his observations based on 59 spontaneous dislocations and 20 complete ruptures of the LHBT. He was the first to describe the supratubercular ridge (Fig. 26-2), degenerative changes on the undersurface of the acromion, the acromioclavicular joint, and the coracoacromial ligament. Meyer thought that attrition, particularly after use of the extremity in abduction and external rotation, led to gradual destruction of the capsule proximal to and in the region of the lesser tuberosity. Dislocation resulted as a consequence of capsular weakness in this region.
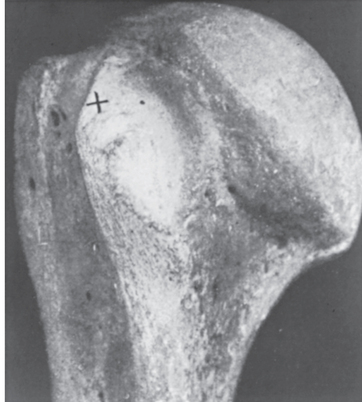
FIGURE 26-2 Original photograph of the supratubercular ridge taken by Meyer.
(From Gilcreest EL: Dislocation and elongation of the long head of the biceps brachii. Analysis of six cases. Ann Surg 104:118-138, 1936.)
According to Schrager,31 F. Pasteur32 recognized all facets of biceps tendinitis and described it fully, elevating its status as a distinct clinical entity. In 1934, the diagnosis of biceps tendinitis was questioned by Codman,33 who wrote, “Personally, I believe that the sheath of the biceps is less apt to be involved than are other structures. I have never proved its involvement in a single case. I think that the substance of the tendon of the supraspinatus is most often involved.” In the 1940s, Lippmann,2,34 Tarsy,35 and Hitchcock and Bechtol36 all believed that biceps tendinitis was an important cause of shoulder pain, and each independently described tenodesis procedures. In 1950, DePalma37 described degeneration of the biceps tendon with aging and reported on both operative and nonoperative care. Based on gross and microscopic examination of 78 cases, he believed bicipital groove tenosynovitis was the most common cause of a painful and stiff shoulder.
In 1972, Neer38 described the anterior impingement syndrome in which anterior acromial spurring with thickening and fibrosis of the coracoacromial ligament causes impingement wear on the rotator cuff and biceps tendon. He pointed out a close association between rupture of the biceps tendon and rotator cuff tears.
Although the pendulum had swung away from primary biceps tendinitis and isolated biceps tendon instability since the 1970s, it appears to be swinging back. Several authors have published results of the diagnosis and treatment of isolated biceps tendinitis and dislocation. Post39 presented a series of patients with primary bicipital tenosynovitis, and O’Donoghue40 has reported on surgical techniques for treating the subluxating biceps tendon in athletes.
Since the late 1980s and early 1990s, the increased use of MRI and arthroscopy has provided valuable information about the LHBT and its lesions. In 1985, Andrews41 described tears in the superior labrum of the glenohumeral joint at the attachment of the biceps tendon. In 1990, Snyder42 first coined the term SLAP lesion (superior labrum anterior and posterior lesion) and described a classification system for it.
In the early 1990s, Walch43,44 classified various subluxations and dislocations of the biceps tendon. Since then, he has described the biceps pulley and pulley lesions (Fig. 26-3A and B). Pulley lesions involve the rotator interval and cuff and therefore affect the stabilizers of the LHBT. These hidden lesions,45 as described by Walch, are not always visible on arthroscopic examination, and open exploration of the rotator interval is sometimes required to identify them. Study of these lesions has directly incriminated the superior glenohumeral and coracohumeral ligaments and the subscapularis and supraspinatus in allowing biceps subluxation and dislocation. Bennett46 has shown that good visualization of the rotator interval, including the coracohumeral and superior glenohumeral ligaments, is possible with the arthroscope.
ANATOMY
The long head of the biceps brachii originates, in most cases, at the supraglenoid tubercle and glenoid labrum in the superior-most portion of the glenoid (Fig. 26-4). Some authors47–49 have described absence of the intra-articular portion of the long head of the biceps or an extra-articular structure. The tendon itself is approximately 9 cm long. At its origin, the tendon presents a variable insertion type. It may be bifurcated or trifurcated or it can have a single insertion point.50
In a study by Habermeyer and associates,51 the biceps was found to originate off of the supraglenoid tubercle in 20% of specimens studied. In 48% of the specimens the origin was off the superior-posterior aspect of the labrum, and in 28% of the specimens the origin was from both the tubercle and the labrum.
Classically, the anterior-to-posterior distribution of the biceps attachment has been classified into four types as described by Vangsness (Fig. 26-5A to D). The attachment is primarily posterior most of the time, with fewer specimens showing contributions from the anterior labrum.52–54 Tuoheti and colleagues questioned whether the anterior labrum contributes at all to the biceps origin.55 They looked macroscopically as well as microscopically at the attachment site of the LHBT in a series of 101 cadaveric specimens. In this study they found a distribution of 27% all posterior, 55.4% posterior dominant, 16.8% equal anterior and posterior, and 0% all anterior when the attachment of the biceps was assessed macroscopically. However, when these same specimens were assessed microscopically, the attachment site of the LHBT was seen to be predominantly posterior in all cases. This was the case even in specimens that had macroscopically appeared to have equal contributions from anterior and posterior. The authors noted that the macroscopic assessment was often influenced by the variable attachment of the inferior glenohumeral ligament to the labrum. When the inferior glenohumeral ligament attachment was close to that of the long head of the biceps, the macroscopic impression given was of a posterior-dominant or an equal anterior-posterior insertion. Microscopic examination, however, revealed that the attachment, even in these cases, was predominantly posterior.
The cross-sectional characteristics of the long head of the biceps change during its course from the supraglenoid tubercle down to the musculotendinous junction.56 The shape of the proximal tendon differs from that of the middle and distal portions of the tendon. The proximal part of the tendon is flatter and becomes more circular as it enters the bicipital groove.
McGough and associates tested the tensile properties of the LHBT in normal specimens.57,58 They measured the cross-sectional area of the biceps tendon in three areas: proximal, middle, and distal. The tendon was found to be 22.7 ± 9.3 mm2, 22.7 ± 3.5 mm2, and 10.8 ± 2.8 mm2, respectively. There was no statistically significant difference among the three regions. The ultimate tensile strength, ultimate strain, and strain energy density were measured for all specimens and revealed 32.5 ± 5.3 MPa, 10.1% ± 2.7%, and 1.9 ± 0.4 MPa, respectively. The modulus of elasticity was calculated to be 421 ± 212 MPa. The mode of failure of the tendons was complete rupture within the midpoint of the tendon substance in all cases.
The angle formed by a line from the bottom of the groove to a central point on the humeral head is constant and corresponds to the retrotorsion angle measured from the epicondyles (Fig. 26-6).59 This angle can be referenced as a guide when placing a humeral head prosthesis. The bicipital tendon, though intra-articular, is extrasynovial. The synovial sheath reflects on itself to form a visceral sheath that encases the biceps tendon (Fig. 26-7). The sheath is open, communicates directly with the glenohumeral joint, and ends in a blind pouch at the level of the bicipital groove.
The LHBT can be divided into two zones. The first is the traction zone, in which the tendon of the biceps closely resembles a normal tendon. The second is the sliding zone, which is the fibrocartilaginous portion of the tendon that is in contact with the bony groove. The density of intratendinous vessels in the traction zone is comparable to the vascularization of other tendons. In the sliding zone, vascularization of the biceps tendon is markedly decreased. No vessels are present in the part of the tendon on which the humerus slides. A difference in blood supply has been reported between the sliding and intra-articular portions.60 This area has also been shown to be composed of fibrocartilage. The portion of the long head of the biceps inside the bicipital groove possesses a mesotendon that arises from the posterolateral portion of its groove.51 Vascularization appears to play a minor role in the pathogenesis of biceps tendon rupture.
Distally, the long head of the biceps and the short head of the biceps come together to form a common tendon before inserting onto the radial tubercle. A third muscle belly has been described in some specimens. Mercer61 and Gilcreest62 measured the tensile strength of the distal biceps tendon and found it to range from 150 to 200 lb. The blood supply of the muscle belly of the long head of the biceps is via the brachial artery.51 The nerve supply to this muscle is via the musculocutaneous nerve arising from C5–C7.
Rotator Interval
The rotator interval is an integral part of the cuff and capsule and is distinguishable only by sharp dissection.63 The most important retaining structure in this area is the portion of the shoulder capsule thickened by the coracohumeral ligament and the edges of the subscapularis and supraspinatus tendons; this part of the capsule bridges the tuberosities in the uppermost portion of the sulcus (see Fig. 26-3A). This portion of the capsule is the first and foremost obstacle to medial dislocation of the tendon. Meyer23,28 found in his series that in cases of dislocation of the long head of the biceps, this portion of the capsule was always stretched or torn. Codman,33 commenting on Meyer’s work, was of the opinion that “displacement of the tendon is a result of rupture at that portion of the musculotendinous cuff, which is inserted into the inner edge of the intratubercular notch.” This opinion is supported by the findings of Sakurai and colleagues,64 who observed the transverse humeral ligament to be intact in 25 specimens that nonetheless had medial displacement of the biceps. The rotator interval contains two structures that are important in stabilizing the biceps tendon within the groove: the coracohumeral ligament and the superior glenohumeral ligament.
The coracohumeral ligament has a broad, thin origin on the coracoid. This origin is along the lateral border of the coracoid, and as the ligament passes laterally, it divides into two main bands. One band inserts onto the anterior edge of the supraspinatus tendon and the greater tuberosity. The other band inserts onto the superior border of the subscapularis, the transverse humeral ligament, and the lesser tuberosity (Figs. 26-8 and 26-9). The coracohumeral ligament has extensions that envelop the cuff tendons and blend into the superficial and deep layers of the supraspinatus and subscapularis tendons and the articular capsule. These extensions reinforce the capsule in the rotator interval at the border of the tendinous cuff.65 The coracohumeral ligament is superficial to the shoulder capsule and overlies the biceps tendon.
The superior glenohumeral ligament is the second structure stabilizing the biceps in the rotator interval. It arises from the labrum adjacent to the supraglenoid tubercle, inserts onto the superior lateral portion of the lesser tuberosity, and blends into the medial aspect of the coracohumeral ligament. It crosses the floor of the rotator interval.66 Along with the coracohumeral ligament, the superior glenohumeral ligament forms a reflection pulley for the biceps tendon. This pulley is in direct contact with the insertion of the subscapularis tendon. All these structures blend together to form a sleeve above the entrance to the bicipital groove that is analogous to the flexor tendon pulleys of the hand.43 This sleeve prevents the medial dislocation of the long biceps tendon. Though the superior glenohumeral ligament was previously considered insignificant, it is now considered an important stabilizer for the biceps tendon.
Cole and coauthors67 described the anatomy of the rotator interval in both adults and fetuses. They found two distinct types. Type I intervals have a contiguous layer of capsule in the region of the superior and middle glenohumeral ligaments. On the other hand, type II intervals have a defect in the capsule between the superior and middle glenohumeral ligaments. The type II interval occurred more commonly (28 of 37 specimens). This study suggests that interval defects may be a congenital phenomenon. In Werner and colleagues’ study68 of the rotator interval, the superior glenohumeral ligament was found to make a U-shaped sling to stabilize the biceps tendon in the groove. The fasciculus obliquus also plays a significant role in investing the biceps tendon, as was revealed on microscopic examination. The subscapularis was not found to be involved in this suspensory sling. This suspensory mechanism protects the biceps against anterior shearing stresses. The authors concluded that the superior glenohumeral ligament is the most important stabilizing structure for the biceps tendon and that injury to this sling might lead to anterior bicipital instability.
The Groove
The supraspinatus and subscapularis tendons fuse to form a sheath that surrounds the biceps tendon at the proximal end of the groove. Fibers from the subscapularis tendon pass below the biceps tendon and join with fibers from the supraspinatus to form the floor of the sheath (Fig. 26-10). The floor of the sheath is formed by the superior portion of the subscapularis and supraspinatus tendons. A slip from the supraspinatus forms the roof of the sheath along with the superior glenohumeral and coracohumeral ligaments. The deep portion of the sheath runs adjacent to the bone and forms a fibrocartilaginous lining in the groove that extends approximately 7 mm distal to the entrance of the groove.69
The transverse humeral ligament’s role in stabilizing the biceps in its sulcus has been disputed by several authors.23,64,70 Traditionally, the LHBT was thought to be maintained within the sulcus by the action of the transverse ligament (Fig. 26-11). Meyer23 found that the transverse ligament was in fact either too weak or often entirely absent. The very existence of the transverse ligament as a separate discrete structure has been disputed by Gleason and coauthors, who showed continuation of fibers from the supraspinatus and subscapularis tendons, even in the distal portion of the groove.71 Paavolainen70 and coworkers were unable to dislocate the biceps even after sectioning the intertubercular transverse ligament, as long as the rotator cuff was intact.72
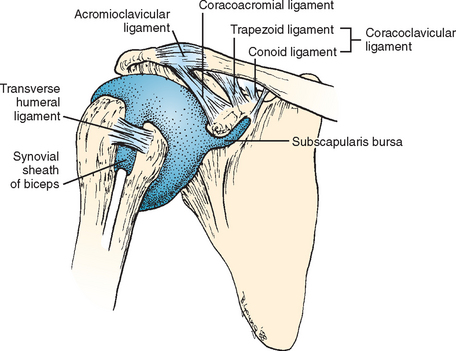
FIGURE 26-11 The ligaments around the shoulder. Meyer23 found the transverse ligament to be too weak or entirely absent.
Osseous Anatomy
The bicipital groove is formed between the lesser tuberosity medially and the greater tuberosity laterally. Cone and colleagues73 have extensively studied the bicipital groove, measuring the width and depth of the intertubercular groove and the angle of the medial wall (Fig. 26-12). In assessing the width of the intertubercular sulcus, two separate measurements were obtained from the bicipital groove view. The first measurement was the top width, or the distance between the medial and lateral lips of the intertubercular sulcus. The second measurement was the middle width, or the distance between the walls of the sulcus but taken at a depth halfway into the sulcus. The difference between measurements was varied, but the ratio of the top width to the middle width was constant at 1.6. The average depth of the groove was found to be 4.3 mm (range, 4-6 mm), and the mean medial wall angle was 56 degrees (range, 40-70 degrees).
In a separate study, Ueberham and Le Floch-Prigent74 measured the intertubercular sulcus in dry bones. They found the total length of the groove to be 27.5 mm. On average, the proximal part of the intertubercular sulcus was 12.4 mm and the most distal part was 15.1 mm. They noted that the groove was deepest in the middle and shallower at both its proximal and distal ends. They measured the angle between the proximal segment of the groove and the distal segment and found it to be 142 degrees with a high degree of variability. A supratubercular ridge was present in 45% of specimens and was thought to force the biceps anteriorly, thereby increasing the risk of dislocation. This abnormality was found in isolation in 17% of specimens or in association with one other lesion in 18%.
Variability in the medial wall angle was confirmed in studies by Hitchcock and Bechtol,36 Habermeyer,51 and Cone.73 Hitchcock and Bechtol found that the medial wall angle was 90 degrees in 10% of specimens, 75 degrees in 35%, 60 degrees in 34%, 45 degrees in 13%, 30 degrees in 6%, and 15 degrees in 2% (Fig. 26-13). They correlated (as did Habermeyer) the medial wall angle with probability of subluxation of the LHBT. Cone, on the other hand, did not find any correlation between the incidence of subluxation and low medial wall angles.
Vettivel and associates75 found that the shape of the intertubercular sulcus correlated with hand dominance. In their study, the intertubercular sulcus was wider and had a more acute medial wall angle on the dominant extremity. They attributed this difference to greater stress passing through the tendon in the dominant extremity, especially during manual activities.
Comparative Anatomy
Hitchcock and Bechtol,36 using specimens from the Museum of Natural History in Chicago, outlined changes in the relationship of the scapula and bicipital groove from the quadruped to the erect biped (Fig. 26-14). They described a progressive anteroposterior (AP) flattening of the thorax resulting in an increased angle that the scapula forms with the thorax and a relative lateral displacement of the scapula. Humans have a relatively short forearm and lateral part of the scapula. Such anatomy necessitates greater medial rotation of the humerus to enable the hand to reach the midline. The AP flattening of the thorax and the short forearm were compensated for, but only incompletely, by torsion of the humerus. In the quadruped opossum, the biceps tendon takes a straight course through the bicipital groove and is an effective abductor of the forelimb in the forward plane. However, in humans, the tendon is lodged against the lesser tuberosity, where a supratubercular ridge or shallow groove can traumatize the tendon. Humans are unique among the primates in having marked variation in configuration of the bicipital groove.
Developmental Anatomy
The development of the glenohumeral joint is similar to that of other synovial joints in the human body. Accord ing to Gardner and Gray,76 it involves two basic processes. Initially, an inner zone forms between the two developing bones of the joint. This is followed by the creation of cavities by enzymatic action. The inner zone often comprises three layers: a chondrogenic layer on either side of a looser layer of cells. The joint capsule and many of the intra-articular structures, such as the synovial membrane, the ligaments, the labrum, and the biceps tendon, are formed from this inner zone of tissue. Giuliani and associates77 confirmed that the tendon of the long head of the biceps brachii arises in continuity with the anlage of the glenoid labrum. At approximately 7 weeks of gestation, the joint is well formed, the humeral head is spherical, and the tendons of the infraspinatus, subscapularis, and biceps, as well as the glenoid labrum, can be seen (Fig. 26-15).
Pathologic Anatomy
Some authors37,78–80 believe that tenosynovitis is the chief cause of pain in biceps tendinitis and that it leads to an altered gliding mechanism of the tendon sheath. They describe gradual pathologic changes in the area of the biceps tendon. Initially there can be capillary dilation, edema of the tendon with progressive cellular infiltration of the sheath and synovium. Filmy adhesions can then form between the tendon and the tendon sheath. In the chronic stage, fraying and narrowing of the biceps tendon occurs along with minimal to moderate synovial proliferation and fibrosis. Ultimately, the tendon is replaced by fibrous tissue, and dense fibrous adhesions become organized. The biceps tendon passes directly under the critical zone of the supraspinatus tendon.
Claessens and Snoek81 described microscopic changes consistent with a relatively avascular state, including atrophic irregular collagen fiber, fissuring and shredding of tendon fibers, fibrinoid necrosis, and a productive inflammatory reaction with an increase in fibrocytes. Refior and Sowa found that the origin of the tendon and the portion of the tendon that exits the sulcus were predisposed to microscopic degeneration.82 They believed that these areas are at the highest risk for tendon rupture. Macroscopic changes noted in cadaver studies by DePalma37,78–80,83 and by Claessens and colleagues81,84 included tendinitis with shredding of the tendon by osteophytes, adhesions of the synovial sheaths and the tendon and its osteofibrous compartment, subluxation or dislocation of the tendon, and rupture of the tendon with retraction of the distal portion or adhesion of the distal portion to the sulcus.
Although it was commonly believed that the dislocated tendon always displaces medially and rides over the subscapularis tendon, Petersson85 found only one such case in his series. He found that in most cases, internal degeneration of the subscapularis in the region of the lesser tuberosity occurred, allowing the tendon to dislocate medially and under the subscapularis. Similar pathologic findings were described by DePalma.
Sakurai and coworkers64 studied three groups of patients with chronic shoulder pathology. Patients with an intact rotator cuff (group 1) did not have any flattening of the tendon in the intertubercular region. Half the patients with partial-thickness tearing or small rotator cuff tears (group 2) had flattening of the tendon in the intertubercular groove. Patients with massive rotator cuff tears (group 3) had tendon flattening in 66% of the specimens. Bicipital fraying was seen in 16% of these cases. Medial displacement was noted in 12% of group 2 and 38% of group 3. Rupture of the biceps tendon was seen in 16% of group 3 specimens. In their study, these authors measured bicipital height, which included the height of the soft tissues. They demonstrated a significant difference in height of the medial wall between patients with medial displacement of the biceps and those without instability. They were unable to show a difference in height of the medial wall among the three groups. The authors concluded that the LHBT could potentially compensate for deficient rotator cuff function. The result of this compensation is relative stenosis of the tendon in the groove. Other gliding disorders can also result from this mismatch between the enlarged tendon size and the noncompliant bony groove. This mismatch may be responsible for degeneration of the soft tissue along the medial wall of the sulcus, causing a pulley lesion and resulting instability.
The biceps tendon and its enveloping synovial sheath can be affected by inflammatory or infectious processes of the glenohumeral joint as a result of their anatomic location and course. Tumorous conditions affecting the synovium of the shoulder can also involve the sheath of the tendon.86 Therefore, tenosynovitis of the biceps accompanies septic arthritis of the shoulder. Similarly, rheumatic inflammation, osteoarthritis, hemodialysis arthropathy,31 and crystalline arthritis of the glenohumeral joint affect the biceps tendon. The clinical syndrome in such cases is dominated by the articular pathology. In the past, biceps rupture has been reported to occur in conjunction with tuberculosis and syphilis.
Osteochondromatosis has also been reported in the shoulder and bicipital sheath.87 This disorder causes pain and loose body formation in the shoulder. Detection of loose bodies in the bicipital sheath on radiography or MRI should arouse suspicion of this pathologic process. Treatment by synovectomy has been successful.
Osseous Pathoanatomy
The shape of the groove has often been implicated in the pathogenesis of biceps tendon ruptures.36,37,59,78–80,88 A shallow, flattened groove (Fig. 26-16) is commonly associated with subluxation or dislocation of the biceps tendon, and a narrow groove with a sharp medial wall and an osteophyte at the aperture is often associated with biceps tendinitis and rupture (Fig. 26-17). Spurs on the floor of the groove can erode the tendon. Although these groove abnormalities can contribute to bicipital tendon problems, it is more likely that some are changes in response to pathology of the soft tissues around the shoulder rather than the underlying cause. In all degenerative conditions about the shoulder, soft tissue changes precede bony changes. For instance, in rotator cuff disorders, fibrosis, bursitis, and tendinosis or ensethopathy precede the formation of spurring in the anterior acromion. Synovitis and cartilage degeneration precede spur formation in the acromioclavicular joint. It seems logical that changes in the bicipital groove and the entrance to the groove would follow changes in the tendon, capsule, ligaments, and synovium around it.
Bony anomalies and variations have been proposed as a cause of subluxation and tendinitis of the biceps tendon. The supratubercular ridge has been described by Meyer23 as a ridge that extends forward and downward from the region of the articular cartilage to the upper and dorsal portions of the lesser tuberosity (Fig. 26-18). The incidence of this structure, according to Cilley,89 was 17.5% in 200 humeri examined. The ridge decreases the depth of the sulcus and diminishes the effectiveness of the tuberosity as a trochlea. Meyer believed that the ridge pushes the biceps tendon against the transverse ligament, thereby favoring dislocation.
In Hitchcock and Bechtol’s series,36 the supratubercular ridge was found to be markedly developed in 8% and moderately developed in 59%. They found a direct correlation between the presence of a supratubercular ridge and spurs on the lesser tuberosity (medial wall spurs). In their series, medial wall spurs were found in approximately 45% of patients with a supratubercular ridge. When no supratubercular ridge was present, only 3% of the humeri had spurs on the lesser tuberosity (see Fig. 26-18C). They concluded that spurs on the lesser tuberosity developed in response to the biceps tendon’s being pressed against the tuberosity by the supratubercular ridge.
A supratubercular ridge was found in approximately 50% of patients in a study by Cone and colleagues.73 They did not, however, find a correlation with it and the presence of bicipital groove spurs. It was their belief that the medial wall spur was related more to a traction enostosis or a reactive bone formation. In one specimen, they found that the transverse humeral ligament had completely ossified, converting the bicipital groove into a bony tunnel. They agreed with DePalma that the presence of bony spurs on the floor of the bicipital groove is related to chronic bicipital tenosynovitis (Fig. 26-19).
Pfahler and coworkers88 prospectively compared the ultrasound and radiographic findings of patients with chronic shoulder pain to those of normal control subjects. They found that 43% of those with radiographically evident degenerative changes of the bicipital groove also had sonographic evidence of biceps tendon inflammation. Conversely, ultrasound findings of biceps tendinitis correlated with a smaller total opening angle (<80 degrees) on radiographs 65% of the time. Patients with a flatter medial angle had signs of biceps tendinitis 47% of the time. In patients with bicipital rupture, 61% had a shallow medial angle. They concluded that 65% of patients in their series with anterior shoulder pain had “conspicuous anatomic findings of the bicipital groove.”
FUNCTION OF THE BICEPS TENDON
Basmajian and Latif90 characterized the action of the biceps brachii muscle as flexion of the elbow joint when the forearm is in the neutral or supinated position but contributing little when the forearm is in a pronated position. They also believed the biceps to be important in decelerating the rapidly moving arm, such as occurs during forceful overhand throwing. There is now general agreement that the biceps brachii is in fact a strong supinator of the forearm and only a weak flexor of the elbow. Debate continues, however, on the exact function of the biceps proximally at the shoulder. Most anatomy texts regard the biceps as a weak flexor of the shoulder.91 Studies on function of the biceps tendon can be divided into three broad categories: direct observation, electromyographic (EMG) studies, and biomechanical cadaver studies.
Direct Observation
Multiple investigators2,34,36,92 have pointed out that the biceps tendon does not slide in the groove. Rather, the humerus moves on the stationary biceps tendon during shoulder motion like a monorail car on a track. From full adduction to full elevation of the arm, the groove moves a distance of up to 2 to 5 cm along the tendon. Maximal excursion of the humeral head along the tendon is produced when the shoulder is in a position of maximal external rotation. Minimal excursion is seen when the shoulder is in a position of maximal internal rotation. To facilitate excursion along the tendon, the synovial pouch extends from the shoulder joint and lines the greater part of the intertubercular groove (see Fig. 26-7). Within this bursa, the tendon glides through its peritendineum.
The LHBT is sometimes divided into an intra-articular segment and an intra-tubercular segment. Lippman2 noted that with the arm in the position of full abduction, 1.3 cm of the LHBT lies within the shoulder joint. This changes, however, when the arm is adducted and externally rotated such that the length of tendon within the joint increases to 5 cm. He therefore questioned the utility of such a distinction given that what portion of the tendon is intra-capsular and what portion is within the groove depends on the position of the arm (Fig. 26-20).
When the arm is in full external rotation, the tendon occupies the floor of the groove, and its more proximal portion presses on the humeral head. Because of this, it was originally believed that in external rotation the long head of the biceps acted as a head depressor at the shoulder to enhance abduction strength at the shoulder. Lucas93 used a force vector diagram to demonstrate how the long head of the biceps could act as a dynamic humeral head depressor. The biceps potentially can also act as a static humeral head depressor, preventing migration of the humeral head into the acromion with contraction of the deltoid (Fig. 26-21). Rowe94 believes that the function of the biceps tendon as a humeral head depressor increases in the context of a chronic rotator cuff tear. He points to the hypertrophy often seen in the tendon in these situations as evidence. Bush95 went as far as to transfer the LHBT laterally when repairing cuff defects and claimed that there was increased humeral head depression when this was done.
Andrews and colleagues41 arthroscopically observed the biceps tendon and the superior glenoid–labrum complex during electrical stimulation of the biceps. They noted superior lifting of the labrum and compression of the glenohumeral joint and concluded that in this respect the biceps is a “shunt muscle of the shoulder” and helps to stabilize the glenohumeral joint during throwing. They agree that its primary role during throwing is still to decelerate the elbow. It is this sudden deceleration that leads to tearing of the superior glenoid–labrum complex by the biceps tendon.
In a study by Itoi and colleagues,96 both the long and short heads of the biceps were shown to function as anterior stabilizers of the glenohumeral joint with the arm in abduction and external rotation. It was further demonstrated that with increasing instability from sectioning of the inferior glenohumeral ligament (as occurs with development of a Bankart lesion) both heads of the biceps have an increased stabilizing function to resist anterior displacement of the humeral head. They concluded that during rehabilitation of patients with chronic anterior instability, biceps strengthening should be included in the nonoperative treatment of these lesions.
Kumar and associates97 also studied the stabilizing role of the biceps tendon. They were able to show that severing the LHBT while both heads were tensed caused significant upward migration of the humeral head. In this manner, the long head of the biceps is important in stabilizing the humeral head in the glenoid during powerful elbow flexion and forearm supination. They warned against sacrifice of the intra-articular portion of the LHBT because of the danger of producing instability during forced elbow flexion and supination.
Other authors have published evidence for the role of the LHBT in glenohumeral stability.98,99 Pagnani99 studied cadaveric shoulders subjected to anterior, posterior, and inferior force at varying arm angles. The amount of translation that occurred with and without an applied force to the biceps was measured. The results showed decreased translation of the glenohumeral joint in all directions when a 55-N force was applied to the biceps. The effect was greatest at middle and lower elevations. Rotation of the shoulder joint also influenced translation; external rotation decreased posterior translation, whereas internal rotation decreased anterior translation. This applied force to the biceps also decreased superior and inferior translation of the glenohumeral joint. Pagnani concluded that the biceps helps to center the humeral head on the glenoid, thus stabilizing the fulcrum at which motion can occur.
Malicky and coworkers98 showed that the biceps provides a 30-N stabilizing force to the humeral head when the arm is in neutral rotation. This stabilizing force depended on the relative position of the groove, with the smallest stabilizing effect when the arm was in external rotation. In neutral rotation, the biceps can add concavity compression as well as a posteriorly directed force on the head against the glenoid. In external rotation, the biceps applies an anteriorly directed force on the humeral head and can actually destabilize the joint.
Warner and McMahon100 studied seven patients with isolated rupture of the LHBT. Rupture was documented either surgically or with MRI. They demonstrated an increased superior translation of the humeral head at all degrees of humeral abduction when compared with the contralateral (control) side. This increased superior translation was not present with the arm at 0 degrees of elevation. They concluded that isolated loss of the biceps could lead to impingement.
Electromyographic Evaluation
Basmajian and Latif90 were pioneers in evaluating the musculoskeletal system, including the shoulder, with integrated-function and dynamic-spectrum EMG. They reported that both heads of the biceps are active during shoulder flexion, and the long head is the more active of the two. Habermeyer51 found clear EMG activity in the biceps during abduction, greatest at 132 degrees of abduction. Interestingly, he found the muscle to be active even with the arm in neutral rotation; that is, the biceps is active in abduction even when the arm is not externally rotated. In flexion, Habermeyer found the most activity during the first 90 degrees of flexion. In flexion, the biceps was active in external but not internal rotation. The effectiveness of the long head of the biceps is greatest in external rotation when its tension is maximal. With the arm adducted and internally rotated, the long head was always inactive, and the short head was active in half the cases of adduction and only seldom active in internal rotation. The biceps was totally inactive in extension.
Laumann101,102 has divided the contributions to shoulder flexion by the shoulder muscles into percentages. He estimates that the biceps contributes approximately 7% of the power of flexion.
Furlani103 studied EMG activity of the biceps during movements at the glenohumeral joint. He found that during flexion of the shoulder with an extended elbow, both the long and short heads of the biceps brachii were active in the majority of cases. This was regardless of whether resistance was applied. This differed in abduction. With abduction without resistance, there was no biceps activity. The addition of resistance, however, increased biceps activity to 10%.
Ting and coworkers104 analyzed the EMG activity of the long head of the biceps in patients with rotator cuff tears. They then correlated their EMG findings with their operative findings. During both shoulder abduction and flexion, four of five subjects demonstrated significantly greater activity of the biceps on EMG in the presence of a rotator cuff tear when compared to the EMG activity of the biceps in their contralateral (uninjured) shoulder. In addition, all shoulders with compromised rotator cuffs proved to have a significantly larger tendon at the time of surgery than noted in controls. (This correlates with observations of Rowe.94) Ting and coauthors suggested that the lateral head of the biceps may be more important to abduction and flexion in the compromised shoulder than in the normal shoulder and that the concomitant enlargement of the tendon may represent use-induced hypertrophy. They therefore recommended against sacrificing the intracapsular portion of the tendon (e.g., for graft material) or tenodesing the tendon indiscriminately in the groove as a routine part of rotator cuff repair and acromioplasty.
The biceps’ contribution to the shoulder during throwing has been evaluated by Jobe and colleagues105 and by Perry.106 In these studies, biceps function correlated with motion occurring at the elbow and not in the shoulder. A relatively stable elbow position during acceleration was accompanied by a marked reduction in the muscle’s firing intensity. During follow through, the need for deceleration of the rapidly extending elbow and pronating forearm was accompanied by peak biceps action. There was no activity in the biceps muscle during the act of throwing, except in conjunction with elbow activity. Peak activity was only 36% of maximum. With a 9-cm2 cross section and only half of the muscle related to the long head, the humeral force is small. In Perry’s words, “It seems doubtful that the long head [biceps tendon] is a significant stabilizing force at the glenohumeral joint.” Glousman and coworkers,107 on the other hand, reported increased activity in the biceps during throwing in patients with unstable shoulders. Therefore, it may be that the biceps increases in importance if the primary stabilizers are injured.
EMG activity in patients with rotator cuff tears less than 5 cm was found to be increased in comparison to healthy subjects by Ozaki.108 This increased activity could be an attempt to compensate for the decreased function of the torn rotator cuff. Ozaki, like Ting, postulates that this increased activity could be responsible for the hypertrophy of the tendon often seen accompanying small and medium rotator cuff tears. Patients with massive rotator cuff tears and dislocated biceps tendons did not show a similar increase in activity.
Biceps and rotator cuff EMG activity were found to precede motion of the glenohumeral joint in a study by David and colleagues.109 They found that EMG activity in these muscles occurs before actual internal or external rotation movement (0.092 ± 0.038 to 0.215 ± 0.045 sec). The deltoid and pectoralis major muscles were also active before glenohumeral motion, but significantly later (0.030 ± 0.047 to 0.12 ± 0.053 sec). They postulated that the biceps and rotator cuff muscles were stiffening the joint, providing a stable platform before initiation of movement. Once movement was initiated, the activity of the biceps depended on the direction of motion. Little activity was found in the biceps during internal rotation, but more activity was seen during external rotation.
Sakurai and associates110 studied EMG activity in the biceps while maintaining the elbow in a brace in neutral rotation. Surface electrodes were used to measure EMG activity of the deltoid and biceps muscles. Their results showed EMG activity in both the long and short heads of the biceps with all motions of the shoulder independent of elbow position. According to their study, the biceps acted as an active flexor and abductor of the shoulder. There was also activity with rotation, and external rotation induced more activity than internal rotation did. They also demonstrated greater fatigability of the biceps in relation to the deltoid. Accordingly, they considered the biceps at higher risk for injury than the deltoid.
In direct contradiction, two other studies found no significant dynamic role for the biceps in glenohumeral stability. Yamaguchi and colleagues111,112 fixed the elbow at 100 degrees of flexion and held the forearm in neutral rotation with a brace in an attempt to relax the elbow flexors. EMG activity of the brachioradialis was used as a control for biceps EMG activity. They found little to no activity in the biceps tendon with shoulder motion, even in patients in whom the head-depressor effect of the cuff musculature had been compromised. Furthermore, no difference was noted between the EMG activity of the brachioradialis and the biceps. They concluded that the role of the biceps in shoulder stability is probably a passive one.
Using a similar experimental design, Levy and coworkers113 studied the EMG activity of the biceps and rotator cuff muscles with intramuscular electrodes during motion of the shoulder. Again, a brace was used to control elbow motion. Three different elbow positions were tested. The shoulders were moved in flexion, external rotation, and internal rotation at two different speeds and with a 5-lb weight. They found no activity in the biceps when elbow motion was controlled. The authors point out that many activities performed by the arm require both elbow and shoulder motion. In their experimental design, elbow motion was intentionally eliminated. The lack of biceps activity seems to reflect the fact that the elbow was controlled. The EMG activity of the biceps in other studies might therefore have been a consequence of active motion or stabilization of the elbow and not the shoulder. Based on this, they concluded that the biceps is not active in isolated motion of the shoulder.
Summary
The tendon’s importance seems to increase in pathologic states of the shoulder, such as rotator cuff tears and shoulder instability, as evidenced by increased EMG activity as well as observation of hypertrophy and resistance to translation. The observation of increased superior translation of the humeral head in patients with confirmed bicipital rupture reinforces these findings. The clinical relevance of this, however, is questionable given that measurable deterioration in shoulder function has not been demonstrated in patients who have undergone biceps tenotomy or tenodesis. Therefore, if the LHBT is implicated as a possible source of the patient’s symptoms (either through physical examination or at surgery), the risk of decreased shoulder function from tenotomy or tenodesis is negligible in comparison to the risk of continued pain from biceps pathology.
CLASSIFICATION OF BICIPITAL LESIONS
Biceps lesions have historically been divided into biceps tendinitis and biceps instability. Often, however, much like rotator cuff tears or lateral epicondylitis, a preponderance of inflammation is not present and the lesion would be more accurately termed tendinosis or tendinopathy. Biceps tendinitis has been subdivided into primary tendinitis (due to pathology of the biceps tendon sheath) and secondary biceps tendinitis (resulting from associated pathology such as rheumatoid or osteoarthritis.) Many authors38,39,78,86,114–117 believe that the pathology seen in the biceps is directly related to its intimate relationship with the rotator cuff. As they pass under the coracoacromial arch, both can be involved in the impingement syndrome. A classification by anatomic locale has been offered by Walch.118
Primary biceps tendinitis has been likened to de Quervain’s tenosynovitis by Lapidus and Guidotti.119 The presence of thickening and stenosis of the transverse ligament and sheath and narrowing of the tendon underneath the sheath was demonstrated by these authors. Post39 found consistent inflammation within the intertubercular portion of the tendon, a proliferative tenosynovitis, and irregularity of the walls of the groove. This inflammation can be visualized arthroscopically if the extra-articular portion of the tendon is pulled into the joint and has been referred to as the lipstick sign. There are no reports of inflammation involving the intra-articular portion of the biceps. Codman33 believed that primary biceps tendinitis was a rare entity. DePalma,37,78–80,83 on the other hand, considered it a common cause of stiff and painful shoulders. In DePalma’s series, 39% of cases had associated disorders. Crenshaw and Kilgore120 were also proponents of primary biceps tendinitis, although 40% of their patients also had an associated lesion. They concluded that whether the biceps tendon or its sheath is involved primarily or secondarily is not important. What is important is that tenosynovitis was the chief cause of pain and limitation of motion.
Isolated rupture of the biceps tendon has been described by several authors.28,91,121,122 Neer believed that most biceps tendon ruptures were associated with supraspinatus tendon tears.38,114,115 Some studies have shown isolated rupture of the biceps tendon occurring in 25% of patients.123 When computed tomographic (CT) arthrograms are performed on patients meeting the clinical criteria for isolated long head of the biceps rupture, the incidence of isolated lesions decreases to 6%.120 In an arthroscopically controlled study of isolated LHBT lesions, a 2.2% rate of isolated rupture was documented. These studies show the rarity of isolated rupture. Many authors have described bicipital groove osteophytes thought to be the cause of rupture of the long head of the biceps.28,121,124,125 Studies using CT scans have shown that the depth and width of the bicipital groove do not have pathognomonic significance.123
Slatis and Aalto126 have offered a useful clinical classification of biceps lesions (Box 26-1). It is based on pathoanatomy and appears to have prognostic significance according to their review.
BOX 26-1 Slatis and Aalto Classification
Type A: Impingement tendinitis
Type B: Subluxation of the biceps
Slatis and Aalto Classification
Type A: Impingement Tendinitis
Type A is tendinitis secondary to impingement syndrome and rotator cuff disease. The torn cuff exposes the biceps to the rigid coracoacromial arch, and such exposure results in tendinitis (tendinosis) (Figs. 26-22 and 26-23).
Type B: Subluxation of the Biceps Tendon
In the type B category, all pathologies of the biceps that involve subluxation and dislocation of the tendon are included (Fig. 26-24). In this group, lesions of the coracohumeral ligament allow the biceps tendon to gradually become displaced medially.
Habermeyer and Walch Classification
Habermeyer and Walch127 classified lesions of the biceps tendon differently (Box 26-2). They noted that the biceps tendon could be involved in pathology in different anatomic locations. In their classification, lesions of the biceps are found at the origin, in the rotator interval, or in association with rotator cuff tears.
Origin Lesions
Origin lesions are described as lesions affecting the attachment of the biceps tendon to the supraglenoid tubercle and to the superior glenoid labrum. These lesions have been observed to occur with the use of muscle stimulation. Biomechanical data by Grauer and coworkers128 have shown that strain on the labrum from the working biceps is greatest when the arm is in overhead abduction.
Interval Lesions
Habermeyer and Walch127 divided interval lesions into three types: biceps tendinitis, subluxation of the LHBT, and isolated rupture.
Biceps Tendinitis
Primary biceps tendinitis, according to Habermeyer and Walch,127 can be diagnosed only by arthroscopy. Findings of erythema and a vascular reaction around the long head of the biceps and in the groove are usually observed. For this diagnosis to be made, the shoulder must have a complete passive range of motion. The tendon should not be subluxated or dislocated out of its groove. Mechanical fraying of the bicipital tendon caused by osteophytes or narrowing of the groove from fracture is considered secondary. In their experience of more than 3 years of arthroscopy of the shoulder looking specifically for isolated biceps tendinitis, they have been unsuccessful in finding one case. Isolated biceps tendinitis remains a diagnosis of exception.43
Subluxation of the Long Head of the Biceps Tendon
The definition of instability of the LHBT is poorly standardized and controversial.40,85,125,129,130 Walch43 defined subluxation of the LHBT as partial or incomplete loss of contact between the tendon and its bony groove. He defined dislocation as complete loss of contact between the tendon and its bony groove. Subluxation has been divided into three types by Walch.
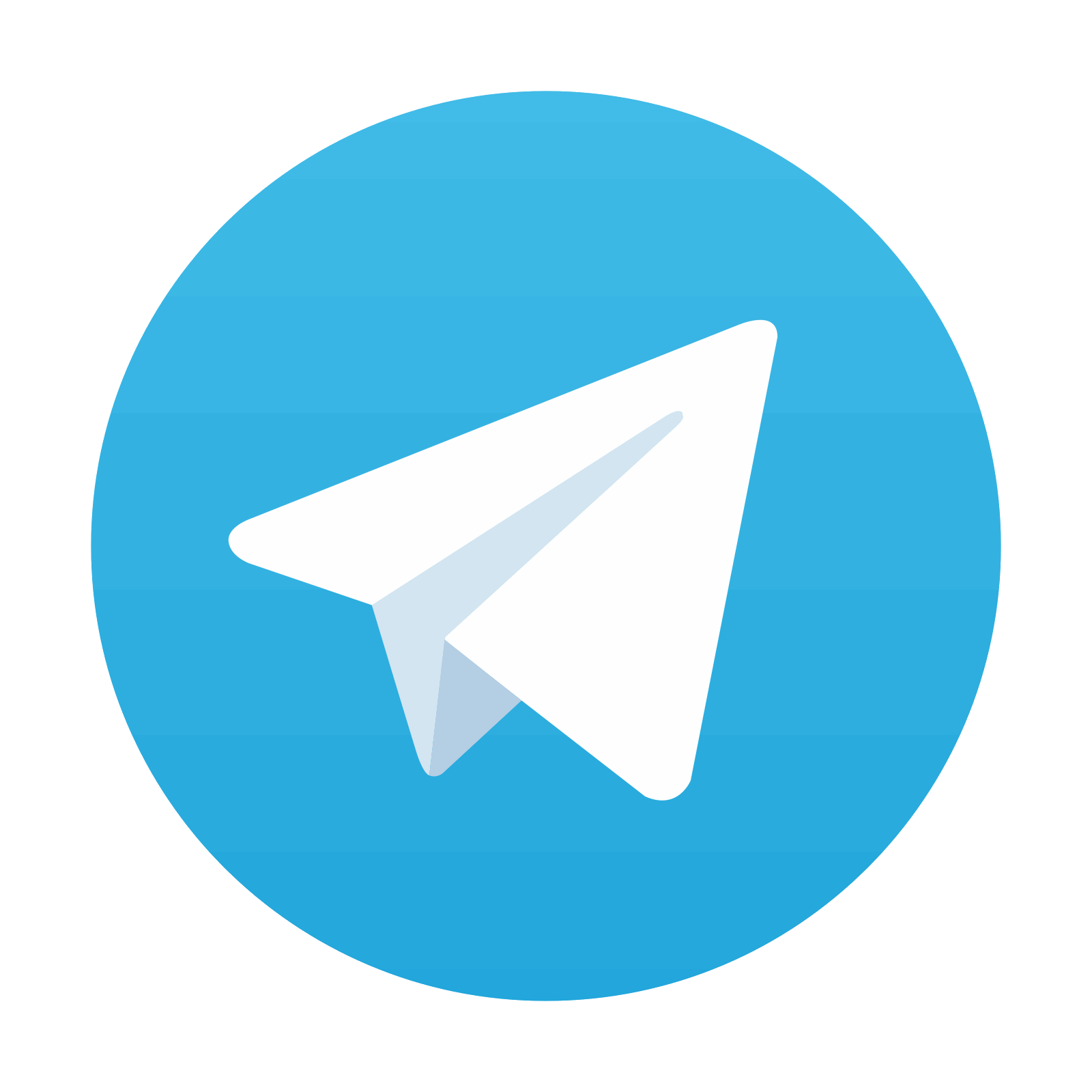
Stay updated, free articles. Join our Telegram channel

Full access? Get Clinical Tree
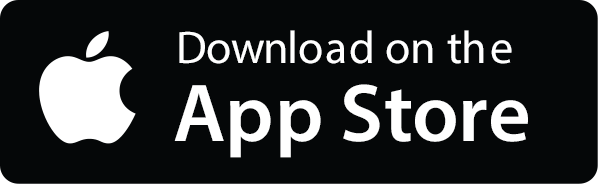
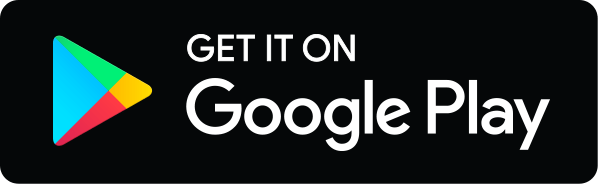