Chapter 51 Future trends and research in orthoses
Current devices
The large majority of orthoses currently used in clinical care are passive, energy-storing devices constructed of relatively rigid thermoplastics lined with soft foam. They contain springs and dampers that provide limited assist and control motion. Use of metal reinforcement can augment stiffness and thus support; however, this adds both weight and cost. More contemporary designs, which include lightweight, energy-storing composites such as carbon fiber, in orthotic construction have shown promising results in terms of patient acceptance and function;5,10 however, these devices are nearly an order of magnitude more expensive and offer little opportunity for postfabrication modification. Traditional ankle–foot orthoses (AFOs) can be fabricated with metal joints. These joints allow simple and much increased adjustment potential, but they increase weight, maintenance, and cost.
Stance control devices
In the past few years, electromechanical knee–ankle orthoses (KAFOs), known as stance control devices, have matured and established themselves, at least commercially. These devices automatically provide support during stance phase and allow knee flexion during swing phase, based on a few simple rules. For example, one device remains locked until contact sensors indicate the leg is in swing phase, and thus the knee is allowed to bend. Another device uses joint kinematics and kinetics to determine when to provide support and when to allow free knee motion. Generally, such selective control makes sense as an improvement over a permanently locked brace that disrupts the fluidity and biomechanics of walking at the cost of providing stance phase stability. How well patient function improves and how well this technology will be received remain to be seen.7 With a sufficiently strong response and interest from patients, practitioners, and insurance companies, more complex control rules may be developed to ensure appropriate functioning across a range of activities and patient abilities. Optimization of control rules likely will take considerable efforts and trial and error; coding up the rules into the mechanics or electronics will be relatively simpler. Others have developed low- or no-power dampened devices to provide variable stiffness. One group uses an oil damper in which flow rate is mechanically controlled, thus allowing variable resistance.13 Another group uses magnetorheological fluid to allow variation of resistance throughout stance phase.1 Both of these applications provide some variability and flexibility, as do the stance control devices. The trick will be to determine precisely what control each patient needs and to implement that control in a practical and tolerable manner with minimal limitations or tradeoffs. This concept of selective control is just another step toward restoring exactly the physiological function lost without providing any restrictions.
Powered devices
Related advancements have centered on developing exoskeleton-powered devices that assist weakness by actuating motion rather than just providing support. Several groups have developed prototypes that do exactly this for knee (Yobotics, Cincinnati, OH, USA) or ankle actuation.1,2 One device seems to have the capability of generating sufficient force outputs, but the device appears bulky and not sufficiently cosmetic to allow long-term use.2 In addition, the reliance on pneumatic power makes it unclear whether the device can be adapted to a low-power, sustained-use, portable electronic device. One limiting factor to full-time use of powered devices is whether their energy requirements can be made sufficiently low or easy and quick to replenish. Also, the need for minimal bulk to achieve high patient compliance is opposed by the need for relatively larger-size actuators to provide sufficient forces.
Although knee orthoses seem to be the target for many of the new product developments, others have realized the potential to provide considerable system-wide control from the ankle and have chosen to focus on this region.1,2,11 The latter group has quantified the effects of ankle support on control of knee stability and speculated that a smart device—one that matches the control required in a pathological gait—may be realizable from the ankle in many situations. AFOs are generally smaller, lighter, more invisible, and less expensive, making them popular choices. It seems reasonable that combinations of this approach with the myriad of new materials and controls being implemented will result in the most versatile and well-tolerated device.
Development of new materials, particularly new electrically controlled fluids (magnetorheological) and actuators that use ionic polymer–metal composites (IPMCs) and microelectromechanical system (MEMS) switches, have the potential to transform the orthotic industry with low-cost, high-performance components.1,13 Electroactive polymers are essentially plastics that can serve as actuators (Fig. 51-1). They can be made to generate forces and displacements on the order of muscles and can do so with considerably less bulk than a typical electrical motor. Field-activated polymers can respond quickly and have high electromechanical coupling that can allow for overall efficiencies as high as 80%. Perhaps most significantly, they can exceed the peak power of natural muscle, allowing for devices of size and mass comparable to natural muscles. These devices will be able to stabilize a joint at the exact time necessary and allow free motion when no or minimal control is needed; all of this at a fraction of the weight of current braces. Commercial development for biomechanical use is under way. Such technology holds promise to supplement or entirely replace conventional passive materials used in braces today. In theory, utilizing the muscles already in place is another step in this direction. Functional electrical stimulation aims to do just this but has limitations.
< div class='tao-gold-member'>
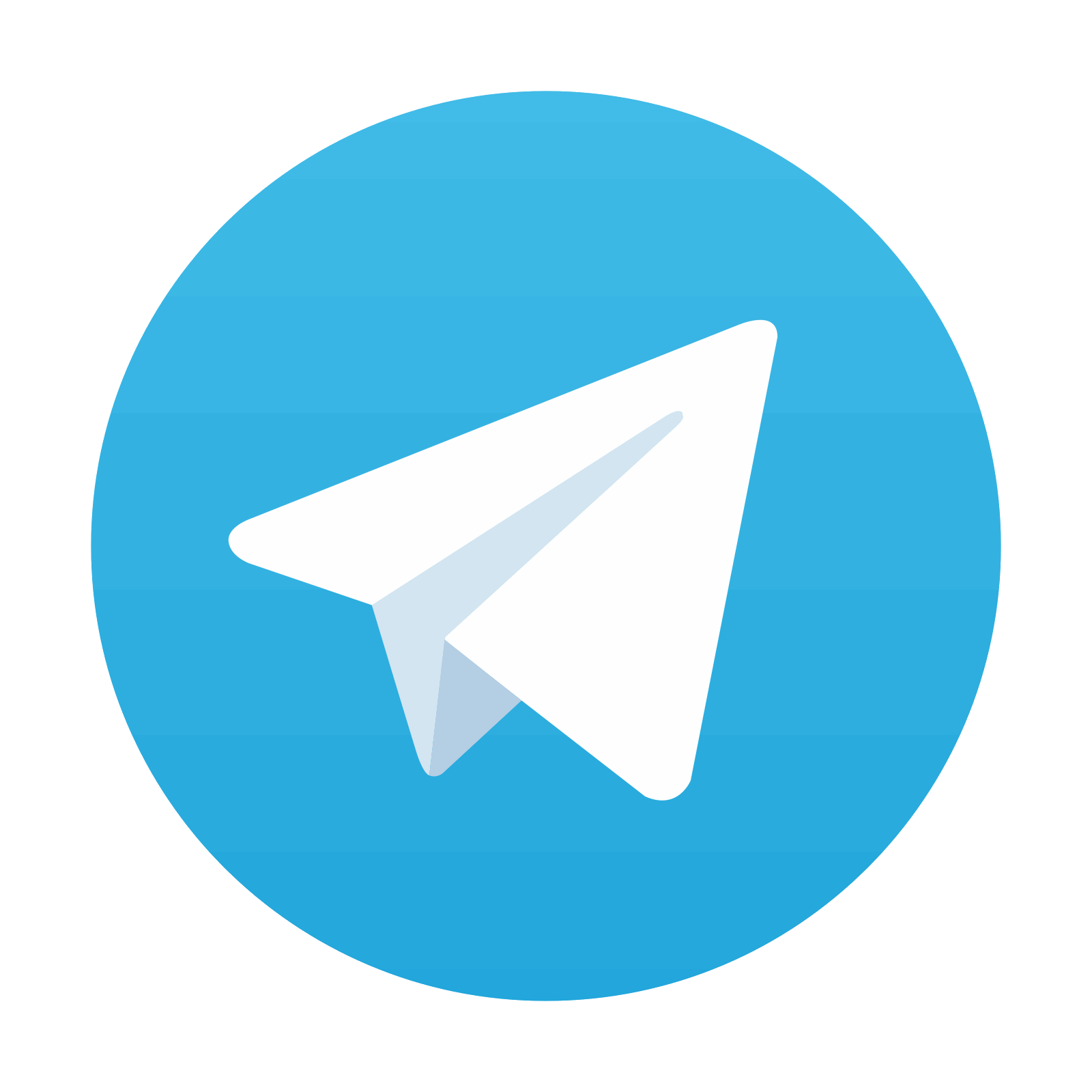
Stay updated, free articles. Join our Telegram channel

Full access? Get Clinical Tree
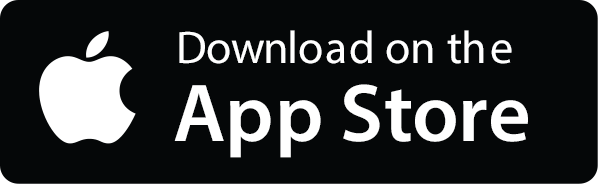
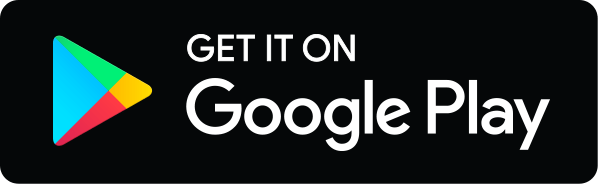