Author and year
Sample size
Race
Age
Gender
Reduced signal intensity
Reduced disc height
Bulging
Annular tears
High-intensity zone
Videman (1995)a
232
Finnish
49.3 (35–69)
100 % M
L1/2
41.6 %
9.3 %
58.6 %
11.6 %
L2/3
48.7 %
13.6 %
74.7 %
17.5 %
L3/4
57.7 %
24.1 %
81.9 %
27.4 %
L4/5
80.4 %
51.3 %
92.8 %
53.1 %
L5/S1
86.0 %
55.6 %
78.5 %
49.8 %
Kjaer (2005b)a
439
Danish
13.1 (12–14)
46.7 % M
L1/2
12 %
3 %
0 %
1 %
0 %
L2/3
8 %
1 %
0.3 %
1 %
0 %
L3/4
13 %
6 %
3 %
2 %
0 %
L4/5
31 %
31 %
10 %
2 %
1 %
L5/S1
50 %
17 %
12 %
3 %
4 %
57.6 % of spine
38 %
16.1 %
7.3 %
5 %
Kjaer (2005a)a
412
Danish
40
48.2 % M
45.2 %
50.2 %
52.4 %
39.3 %
40.8 %
Takatalo (2009)b
558
Finnish
21 (20–22)
58 % M
L1/2
2 %
L2/3
3 %
L3/4
5 %
L4/5
22 %
L5/S1
35 %
47.2 % of spine
24.9 %
9.1 %
6.8 %
Cheung (2009)c
1,043
Chinese
(18–55)
–
18–29
42 %
27.3 %
3.4 %
30–39
48 %
20.6 %
8.2 %
40–49
70 %
27.3 %
16.1 %
≥50
88 %
43.1 %
29.0 %
Mok (2010)c
2,449
Chinese
40.4 ± 10.9 (9.7–88.4)
40 % M
L1/2
9.5 %
L2/3
15.7 %
L3/4
27.2 %
L4/5
45.8 %
L5/S1
51.0 %
Samartzis (2011)c
83
Chinese
18.3 ± 2.1 (13–20)
46 % M
34.9 %
22.9 %
3.6 %
As the prevalence of degenerative findings at different disc levels of the lumbar spine varies considerably, ideally prevalence would be presented level by level. Most disc-specific degenerative findings on MRI, such as bulging, herniation, disc space narrowing, and annular tears, are more common and more severe at the L4/5 and L5/S1 discs than the upper lumbar discs (Fig. 9.1) (Videman et al. 1995). This finding is also consistent between MR evaluation protocols of disc degeneration. In general, degenerative findings in the L1/2, L2/3, and L3/4 discs are more similar to each other, as compared to those in the L4/5 and L5/S1 discs (Table 9.1). Therefore, it may be advisable to, at least, divide the five lumbar intervertebral discs into upper (L1/2, L2/3, and L3/4 discs) and lower lumbar regions (L4/5 and L5/S1 discs) when investigating disc degeneration. There also appears to be a clear difference in genetic influences between upper and lower lumbar regions (Battie et al. 2008), further strengthening the case against using a summary score for degeneration across the entire lumbar spine.
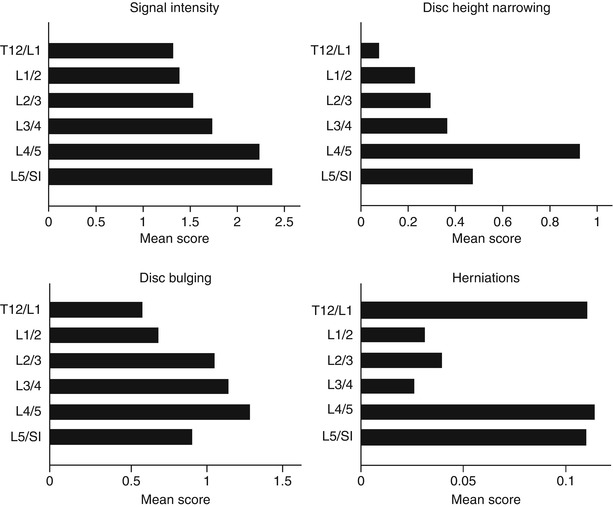
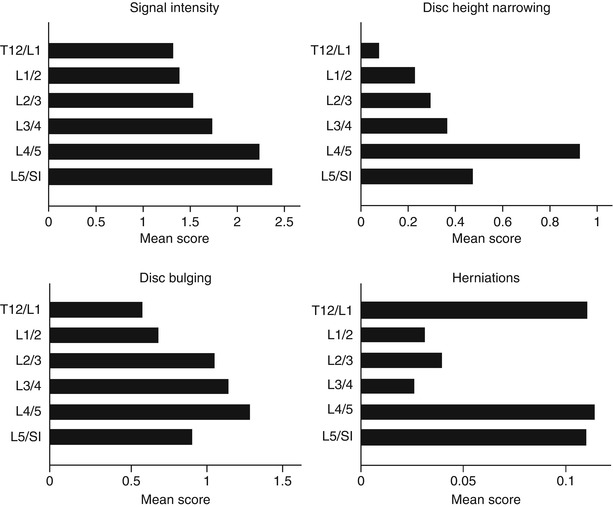
Fig. 9.1
Mean scores for specific manifestations of disc degeneration by disc level (Adapted from the paper by Battié et al. (2004), with permission)
9.5 Etiological Factors (Environmental, Behavioral, and Constitutional)
Before the mid- to late 1990s, the dominant model of the etiology of disc degeneration was one of repetitive loading or wear and tear. This paradigm generally viewed disc degeneration as a consequence of repeated loading and associated tissue insults and injuries. Correspondingly, behavioral and environmental factors, such as occupational materials handling, were viewed as the main causes of disc degeneration. Not surprisingly, these influences were the focus of research and prevention strategies. Based on knowledge of that time, Frymoyer summarized the findings of epidemiological studies on “degenerative disc disease” by stating “Among the factors associated with its occurrence are age, gender, occupation, cigarette smoking, and exposure to vehicular vibration. The contribution of other factors such as height, weight, and genetics is less certain” (Frymoyer 1992). A decade later, a major shift in views was underway. Taking into account new knowledge gained from the intervening decade, in 2002 Ala-Kokko concluded that “Even though several environmental and constitutional risk factors have been implicated in this disease, their effects are relatively minor, and recent family and twin studies have suggested that sciatica, disc herniation and disc degeneration may be explained to a large degree by genetic factors” (Ala-Kokko 2002). Disc degeneration is now considered a condition that is largely genetically influenced, with environmental factors, although elusive, also playing an important role (Battie et al. 2009). An overview of the main factors which have been of interest in the etiology of disc degeneration follows.
9.5.1 Age
With the possible exception of genetics, which will be discussed later, age is perhaps the factor most strongly associated with disc degeneration. The clear relationship between age and disc degeneration has been observed since the time of the pioneering pathologists using autopsy studies in the early twentieth century. Some classic autopsy studies using large samples clearly documented that the occurrence of disc degeneration increased “linearly” with age. For example, in a study from 1926 based on 1,000 consecutive autopsies, Heine reported the prevalence rate of macroscopic disc degeneration (or so-called spondylitis deformans) increased from 0 to 72 % from the age of 39–70 years (Heine 1926). Schmorl and Coventry further confirmed that the occurrence rates of histological findings of disc degeneration, such as disc cell death, nucleus clefts, annular tears, and cartilage endplate fissures, increase consistently with greater age (Schmorl and Junghanns 1971; Coventry et al. 1945a). More recently, Miller reported that the prevalence of macroscopic disc degeneration increased from 16 % at 20 years of age to 98 % at 70 years (Miller et al. 1988). Later, in a systematic investigation of age-related changes in lumbar intervertebral discs, Boos et al. clearly depicted the association of increased prevalence rates of almost all histological degenerative findings in the disc with greater age in a sample spanning from fetal to 88 years (Boos et al. 2002).
On the other hand, some degenerative changes seen from histology can occur in early childhood, complicating the role of age in disc degeneration. For example, mucoid degeneration and mild clefts of the nucleus pulposus may present before the age of two (Boos et al. 2002). In the second decade of life, nucleus pulposus clefts and radial tears are often observed in the disc center, as are cartilage cracks (Boos et al. 2002). From 20 years old on, disc degenerative findings have been found to progressively increase in both frequency and severity. However, there is considerable variability in degenerative disc findings within age groups.
In general, use of MRI has provided a good opportunity to investigate the association between age and disc degeneration. Cerebrospinal fluid-adjusted disc signal intensity measurements, reflecting the water content of the nucleus pulposus, have been found to decrease from early childhood through late adulthood (Videman et al. 1994). In a study of 116 men ranging in age from 35 to 70 years, the associations of different degenerative findings on MRI were plotted in relation to age. Disc signal intensity measurement was more highly associated with age than other MR findings (Fig. 9.2). A further study reported that age could explain up to 31.7 % of the variation of signal-based digital measurements of disc degeneration, such as cerebrospinal fluid-adjusted disc signal, in the upper lumbar intervertebral discs and 11.5 % in the lower lumbar intervertebral discs (Videman et al. 2008). Another advantage of signal intensity measurements acquired through standard MRI, as opposed to qualitative assessments, is that they are continuous in nature, providing greater sensitivity to change and facilitating statistical analyses. However, such quantitative measurements can be influenced by scanner variations and are currently only used for research purposes.
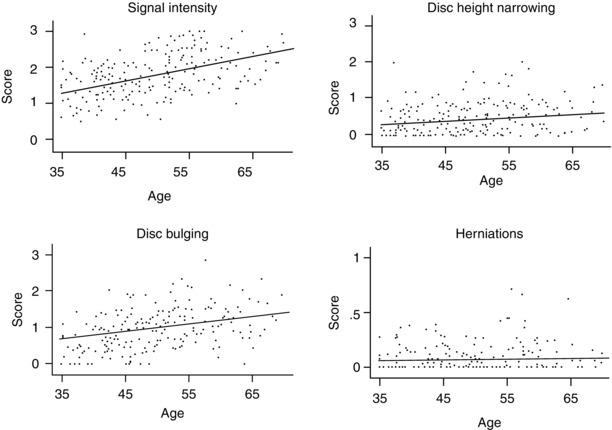
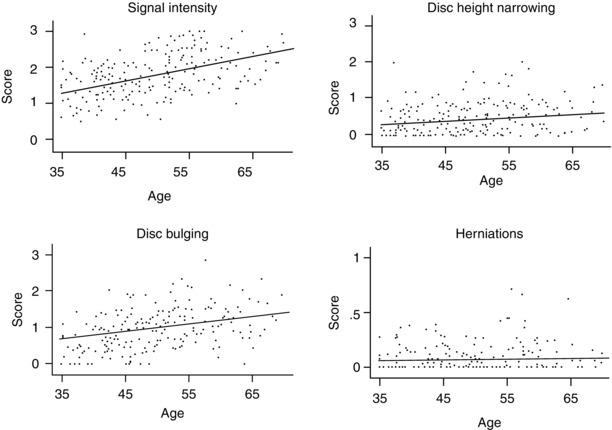
Fig. 9.2
Mean scores for specific manifestations of disc degeneration by age (Adapted from the paper by Battié el al. (2004), with permission)
In epidemiological studies age is a more complicated factor than it may appear (Videman et al. 2008). Age is an intrinsic indicator of the natural process of aging, with a dose-response effect of greater age associated with more degenerative changes. It reflects, however, more than natural aging and can be a complex risk factor with respect to disc degeneration and other outcomes, as it represents not only natural aging but also associated factors. For example, age also reflects cumulative exposure to a variety of known or unknown etiological factors that generally increase with the passage of time. In fact, many factors suspected of accelerating disc degeneration, such as excessive mechanical loading or trauma to the spine, cannot be accurately measured, particularly when lifetime exposures are of interest. However, the exposure to such factors, more or less, is associated with age, with greater age generally associated with greater exposure to all risk factors.
9.5.2 Occupational and Leisure Physical Demands and Spinal Loading
Before genetic factors began to assume a leading role in disc degeneration in the middle to late 1990s (Battie et al. 1995a, b; Sambrook et al. 1999), a wear-and-tear model of disc degeneration prevailed. Various forms of physical demands and spinal loading conditions, particularly heavy material handling, were regarded as the main causes of disc degeneration. The natural assumption under such an injury or wear-and-tear model was that heavy work and greater physical demands would lead to greater disc degeneration. Yet, epidemiological evidence of this has always been conflicted.
In early studies using radiography, it was observed that disc degeneration, as judged from disc space narrowing and endplate sclerosis, was more common in miners and manual workers than in non-laborers (Lawrence 1955) and that the onset of disc degeneration was on average 10 years earlier in workers with heavy physical demands (Hult 1954). Conversely, in a study of 15,160 “back trouble” patients, disc degeneration, as judged from disc space narrowing and osteophytes, was not found to be associated with heavy physical work (Friberg and Hirsch 1949) nor was occupational lifting as reported by others (Frymoyer et al. 1984). More recent studies have continued to produce mixed results, with some suggesting a negative effect of greater physical demands (Seidler et al. 2009), while others fail to observe detrimental effects on the disc (Porter et al. 1989; Videman et al. 2007). In fact, Porter put forward the hypothesis that physical activity strengthens both the vertebrae and discs (Porter et al. 1989). Results of a recent study by Videman et al. suggest that greater loading of the discs during routine activities may even have some positive effects on the disc, as seen through higher disc signal (hydration) (Videman et al. 2010). Also, although occupational loading history, with a dose-response effect, was greater in patients with pain attributed to disc pathology than in controls (Seidler et al. 2009), most studies of the association between mechanical loading and disc degeneration have failed to uncover a clear relationship (Caplan et al. 1966; Riihimaki et al. 1990; Sairanen et al. 1981), further questioning whether there is a strong causal link between physical loading and disc degeneration.
Among the problems underlying the inconsistencies are measurement limitations of exposures and outcomes. Epidemiological studies on mechanical loading and disc degeneration are particularly challenging, as lifetime physical activities and associated loading cannot be measured accurately, which invariably has the effect of diluting the appearance of true associations. Moreover, standard definitions of disc degeneration are also lacking. While most degenerative findings (e.g., signal loss, bulging, narrowing) are correlated to some degree, they do not necessarily represent the same phenomenon and important information may be lost by aggregating distinct findings into summary scores, which is a common practice.
Uncontrolled confounding factors are another major limitation of most epidemiological studies. Exposure-discordant twin studies, which may provide for the best control of known and unknown confounding factors while minimizing extraneous variability related to the substantial genetic influences, provide a particularly strong research design to investigate the effects of environmental exposures. Such studies have been employed to help clarify the effects of a number of suspected risk factors in disc degeneration, which are highlighted below.
It is well known that musculoskeletal tissues, such as bone, ligaments, cartilages, and muscles, are able to increase their physiological capacity and mechanical strength in response to repeated physical activities and greater physical loading. The adaptation to a changing mechanical environment is a rule in sport science and is typically the purpose of exercise and training. Yet, this rule is curiously often viewed as not “applicable” to the intervertebral disc. Instead, mechanical loading or repeated material handling, either from occupation or exercise, is viewed as a hazard that will accelerate disc degeneration. Herein is one of the great paradoxes in the relationship between physical loading and the lumbar spine as compared to other musculoskeletal structures.
Although doubts about the cumulative loading or wear-and-tear model of disc degeneration have long existed, substantial evidence to challenge this paradigm was forthcoming from exposure-discordant twin and heritability studies. Despite extreme exposure, discordance in monozygotic co-twins, very little variance in disc degeneration outcomes was explained by substantial, long-term occupational or sport physical demands and lumbar loading conditions (Battié et al. 2009).
Also, using monozygotic twins with similar lifestyle but substantially different body weights (average body weight difference of 30 lb), long-term physical loading in the form of additional body weight was not associated with increased disc degeneration. Instead, greater body weight within the range studied, which did not include extreme obesity, was associated with slightly less upper lumbar disc desiccation, suggesting that greater routine loading of the lumbar spine is not detrimental to the disc (Videman et al. 2010). The key, perhaps, is the amount of loading and the manner that loading is imposed on the spine in relation to tissue strength, which likely varies substantially between individuals and over the lifespan.
It has been quite common for epidemiological studies of physical loading and disc degeneration to focus on occupational exposures and ignore physical activities outside of work. Considering that activities outside of paid employment usually occupy more of a person’s time than work hours and that combined work and leisure physical loading may give a very different picture than occupational loading alone, this is no small oversight.
In one of the more comprehensive epidemiological studies to date investigating the effects of lifetime exposures to suspected risk factors, physical loading exposures explained only 7 % of the variance in disc degeneration in the upper lumbar levels and 2 % in the lower lumbar levels, suggesting a modest role of commonly experienced occupational and leisure activities in disc degeneration (Battie et al. 1995b). Interestingly, it was exposures reported during leisure activities that accounted for the variance in lower lumbar disc degeneration explained by physical loading exposures.
9.5.3 Driving and Whole-Body Vibration
Whole-body vibration (WBV) resulting from operating or riding in motorized vehicles is a special form of physical loading that was long thought to be deleterious to the lumbar spine and disc, in particular. According to an extensive review conducted in the early 1990s during the height of research activity and interest in whole-body vibration and lumbar disc degeneration, degenerative findings tended to be more common in subjects with greater exposure (Kjellberg et al. 1994). However, the authors of the review also noted “uncontrolled confounding factors may have affected the results in all studies, and the conclusions about the causal role of WBV for the observed injuries and/or disorders therefore become uncertain.”
Since that time, findings have been conflicting and effects uncertain. An exposure-discordant twin study, perhaps the most well-controlled epidemiological study of the association of lifetime exposure to motorized vehicles and related WBV, investigated 45 pairs of monozygotic twin siblings highly discordant for lifetime vehicular driving. No significant differences, nor trends, of greater disc degeneration (e.g., disc signal loss, bulging, herniation, narrowing) were found in drivers as compared to their lesser exposed twin brothers (Battie et al. 2002).
Whether or not WBV related to motorized vehicles exacerbates symptoms from lumbar degenerative conditions or affects other structures is unclear (Lings and Leboeuf-Yde 2000), but these are different issues. Interestingly, vibration has also been pursued more recently as a treatment for low back pain, using various frequencies (del Pozo-Cruz et al. 2011; Rittweger et al. 2002). With respect to deleterious effects of exposures to motorized vehicles and related WBV during work and leisure on disc degeneration in humans, findings have not been persuasive and, in any event, do not suggest a major effect. Yet, after more than 50 years of research on the topic, pleas for continued in-depth biomechanical studies of the effects of vibration on the disc, both good and bad, continue (Hill et al. 2009). This may signify the desire and need for rigorous scientific evaluation to clarify effects, better taking into account frequency, amplitude, and other aspects of vibration. On the other hand, it may also reflect the absence of clear environmental risk factors with substantial effects on which to focus etiological and intervention research.
9.5.4 Trauma
Evidence from both experimental and clinical observations support that clear trauma is a risk factor for disc degeneration. One animal model of disc degeneration involves surgically producing a peripheral annular lesion, which quickly leads to degenerative changes in the disc (Osti et al. 1990). A recent clinical study observed a similar, although less dramatic, causal association between injury to the annulus fibrosus and disc degeneration related to needle puncture in discography. Greater disc degeneration was observed in the non-degenerated or healthy discs following discography over a 10-year follow-up (Carragee et al. 2009). Direct trauma or injury to the disc may destroy the homeostasis of the disc, influence the metabolism of the disc cell, and alter biomechanics of the disc, leading to an accelerated degenerative process.
Trauma to tissues adjacent to the disc, including the vertebral body and endplate, may also accelerate disc degeneration. In animal models, endplate trauma directly induces disc cell apoptosis and promotes disc degeneration (Haschtmann et al. 2008). Clinical long-term follow-up of young people with previous vertebral compression fractures revealed greater amounts of disc degeneration than in healthy controls, also supporting an association of endplate trauma with accelerated disc degeneration (Kerttula et al. 2000).
The question is: how much trauma or force is needed to damage the human disc in vivo and accelerate disc degeneration? A recent autopsy study found that a history of lumbar injury, which referred to sudden onset back pain associated with a specific accident or unusual activity, was associated with the presence of lumbar endplate lesions, which were closely associated with more severe discographic disc degeneration (Wang et al. 2012a; Wang 2011) Endplate lesions may be one mechanism through which trauma or injury eventually leads to disc degeneration. Unfortunately, current clinical imaging modalities are unlikely to be capable of detecting endplate and annular lesions to the same degree, although the sensitivity of MRI to disc disruption may be improving.
Another study of recalled back “injuries” related to sport, leisure, or work activities, and episodes of sudden onset low back pain associated with an exceptional physical activity, used an exposure-discordant twin model. The study revealed that “back injury” based on recollection of such traumatic incidents was not associated with a subsequent increase in disc degeneration, as judged from disc height narrowing or reduced signal intensity (Hancock et al. 2010). The inconsistencies between studies could be explained by high variability in the degree of trauma experienced and reported and that pain was the indicator of “injury,” which may not have been associated with substantial or consequential tissue change to the disc. Also, some painful injuries to the back may have been forgotten, resulting in recall bias.
9.5.5 Smoking
Cigarette smoking is the only chemical exposure known to associate with lumbar disc degeneration. While it is commonly accepted that smoking has deleterious effects on the disc and research has turned to understanding the mechanisms involved (Vo et al. 2011), it is important to keep in mind that the effects are likely to be very modest. Using identical twins grossly discordant for smoking exposure (over 32 pack-years), the overall disc degeneration across the entire lumbar spine was greater in smokers as compared to their nonsmoker siblings. Yet, the effect size was very small, explaining less than 2 % of the variance in degeneration scores (Battie et al. 1991).
9.5.6 Vertebral Endplate Morphology and Status
Although substantial progress has been made in understanding the pathogenesis of disc degeneration, the pathological mechanisms or pathways leading to degeneration are far from explicit. Recent research suggests that the endplate, an interface tissue between the vertebral body and the intervertebral disc, may be the structure through which a number of etiological factors lead to disc degeneration (Wang 2011).
The endplate is essential to maintaining the structural integrity and physiological function of the intervertebral disc (Moore 2006). It is the physical shield preventing the nucleus pulposus from “escaping” (Roberts et al. 1996) and the mechanical interface facilitating load distribution in the vertebra-disc complex (Brinckmann et al. 1983; Setton et al. 1993; Ferguson and Steffen 2003). In addition, it is the gateway for metabolite transport between the vertebral marrow and intervertebral disc (Benneker et al. 2005; Nachemson et al. 1970; Urban et al. 2004).
Given the multiple functions of the endplate, its morphometrics are generally thought to associate with disc degeneration. Yet, due to variations in study materials and limitations of inadequate measurements, the association between endplate morphometrics and disc degeneration remains largely unclear and controversial. In a series of studies to investigate the role of endplate morphometrics in disc degeneration (Wang 2011), a large sample of lumbar endplates was extensively measured in vitro using accurate technologies, such as laser scanning and micro-CT. Among the endplate morphometrics studied, including thickness, concavity, circularity, size, and bone mineral density, only endplate thickness (Wang et al. 2011) and size were found to associate with the degenerative disc (Wang 2011). However, the observed associations were relatively weak, suggesting their contribution may be relatively small.
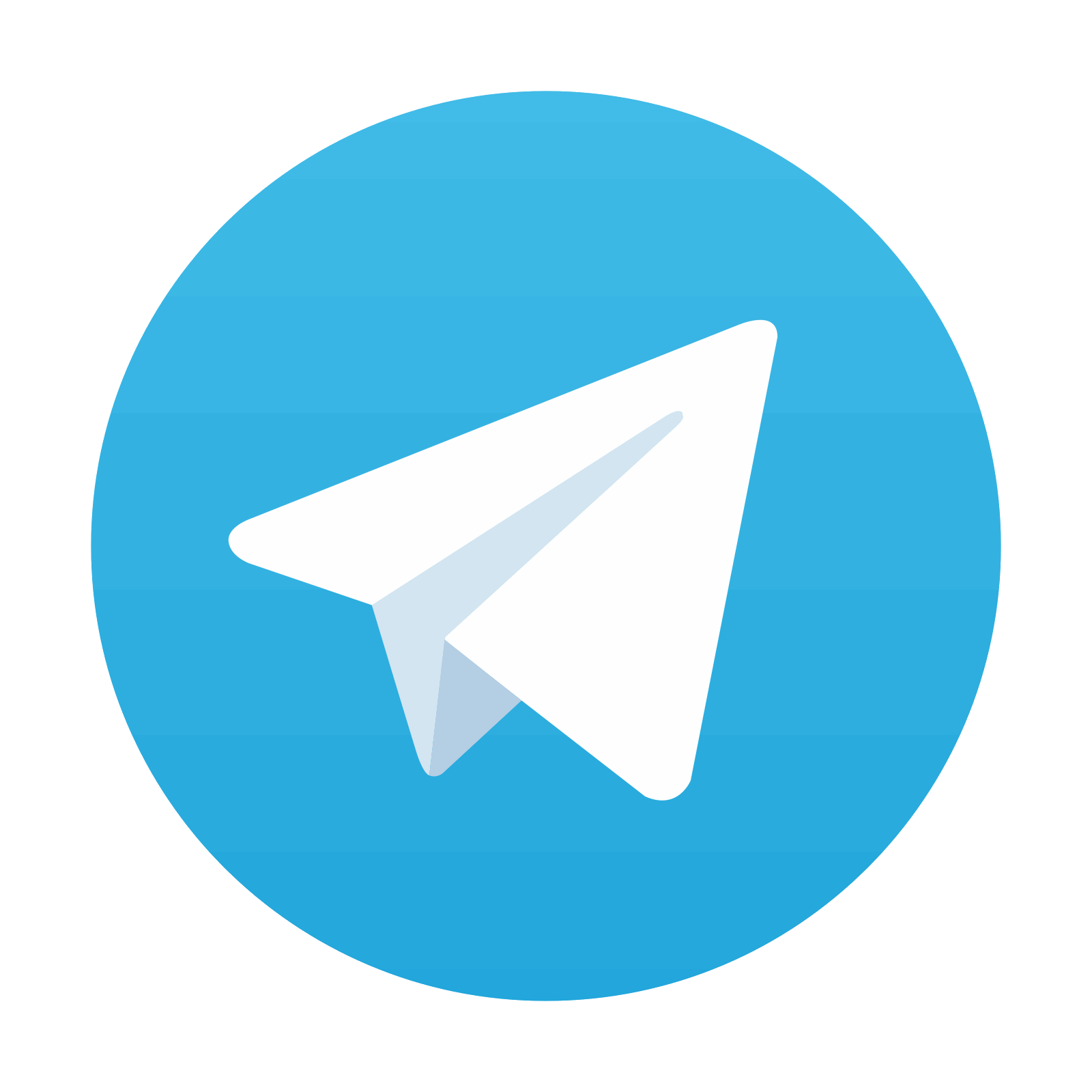
Stay updated, free articles. Join our Telegram channel

Full access? Get Clinical Tree
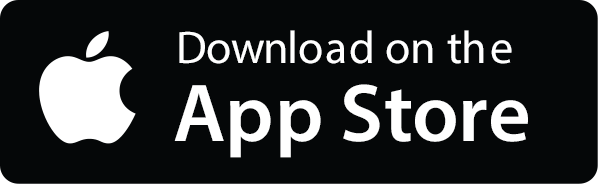
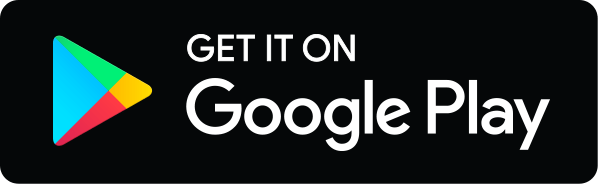